Abstract
Metalloendoproteases have been implicated in the calcium-dependent exocytosis of histamine from mast cells and in the calcium-dependent fusion of myoblasts. Because metalloendoproteases have also been identified in nervous tissue, we investigated the possibility that these proteases may be involved in neurotransmitter release at mammalian synapses. End-plate potentials were recorded intracellularly from mouse diaphragm/phrenic nerve preparations in vitro. The amplitude of the endplate potentials were reduced by as much as 90% during bath application of phosphoramidon, a specific inhibitor of metalloendoproteases, and by carbobenzoxy-dipeptide-amide synthetic substrates for metalloendoproteases. Only those synthetic dipeptides in which the amino group of the peptide bond was provided by a bulky hydrophobic amino acid, such as phenylalanine or leucine, which are substrates for metalloendoproteases, reduced synaptic transmission. Synthetic substrates in which proline or glycine provided the amino group of the peptide bond, which are not metalloendoprotease substrates, had little or no effect on the amplitude of end-plate potentials. The ability of synthetic substrates to reduce synaptic transmission was also dependent on the amino acid that provided the carboxyl group of the peptide bond, with glycine being more effective than tyrosine or serine. In addition, synthetic dipeptides with free carboxyl or amino termini, which have a low affinity for metalloendoproteases, also had little effect on synaptic transmission. The inhibition of synaptic transmission by phosphoramidon and the synthetic substrates occurred within 2 to 3 min and was completely reversible. Neither phosphoramidon nor the synthetic substrates altered the dose-response characteristics of the postsynaptic membrane to bath-applied carbachol. These results suggest that synaptic transmission requires the activity of a metalloendoprotease in the presynaptic nerve terminal and that proteolysis may be an important step during neurotransmitter exocytosis.
Full text
PDF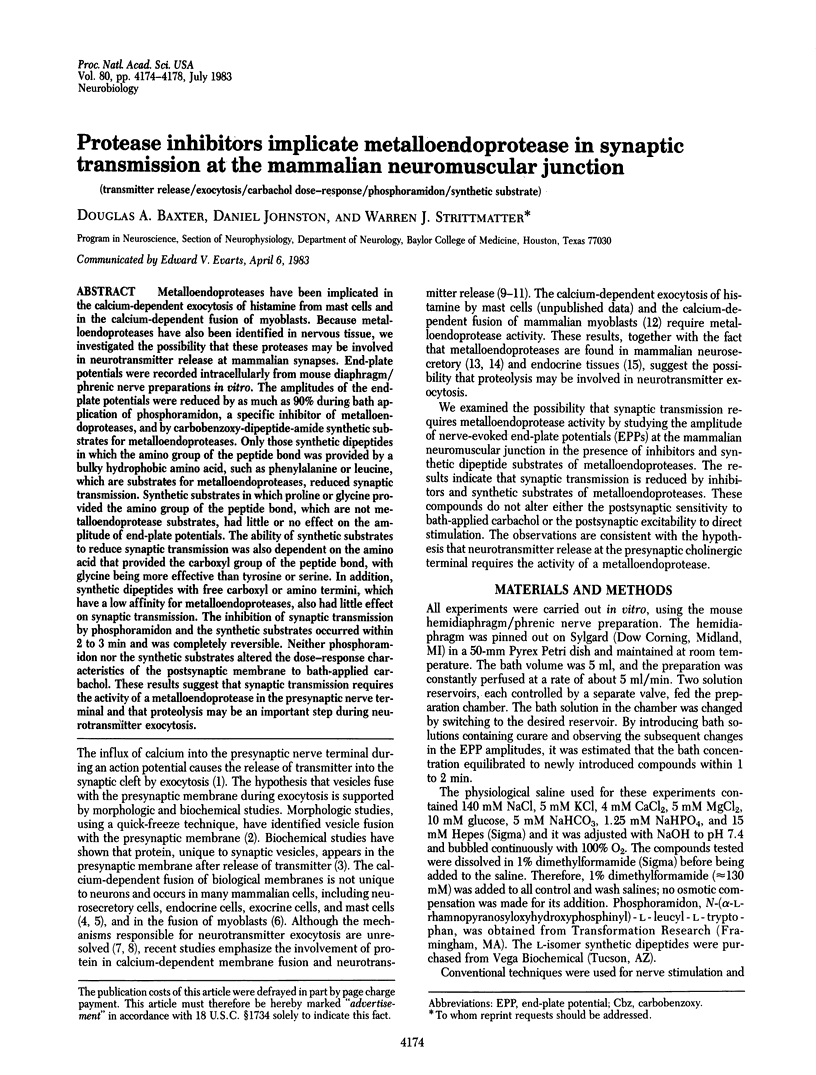
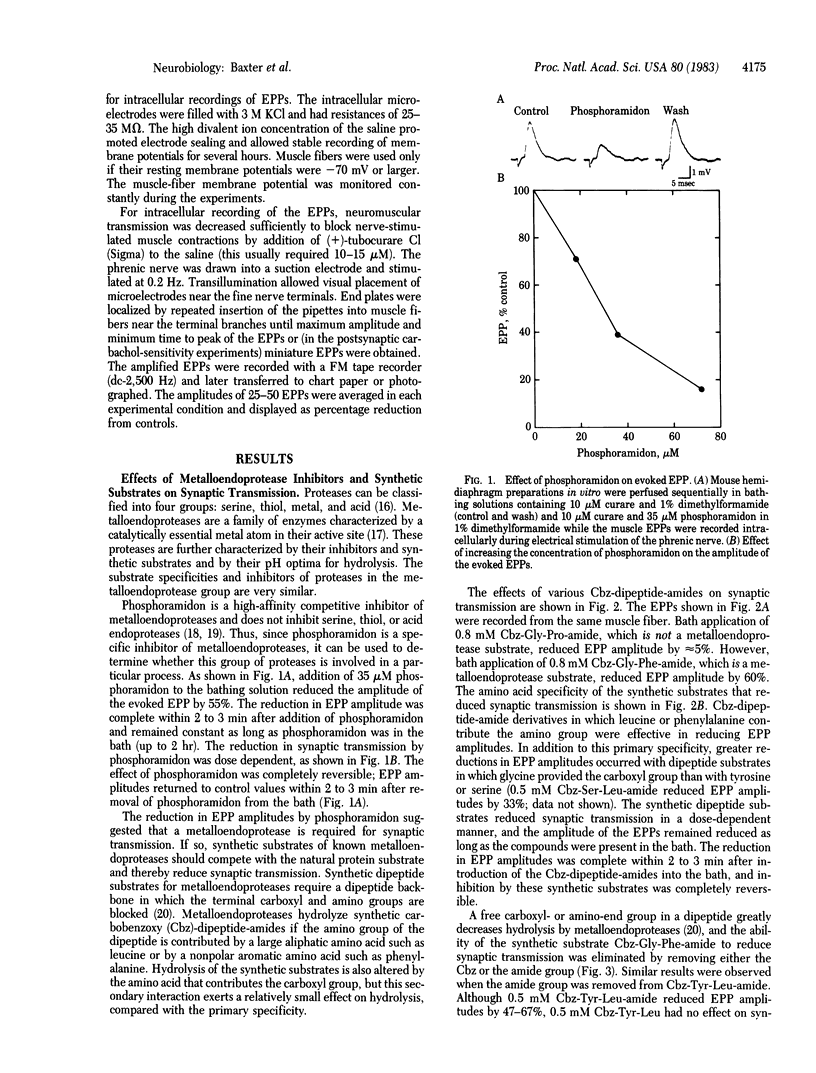
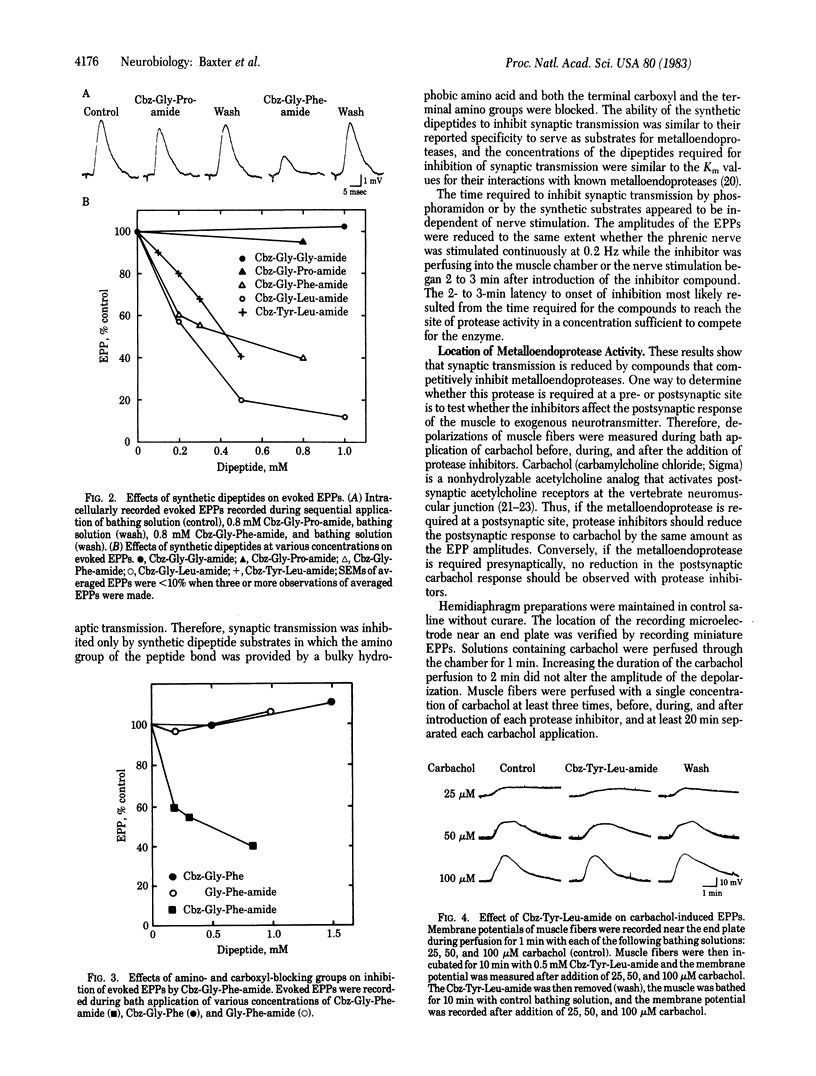
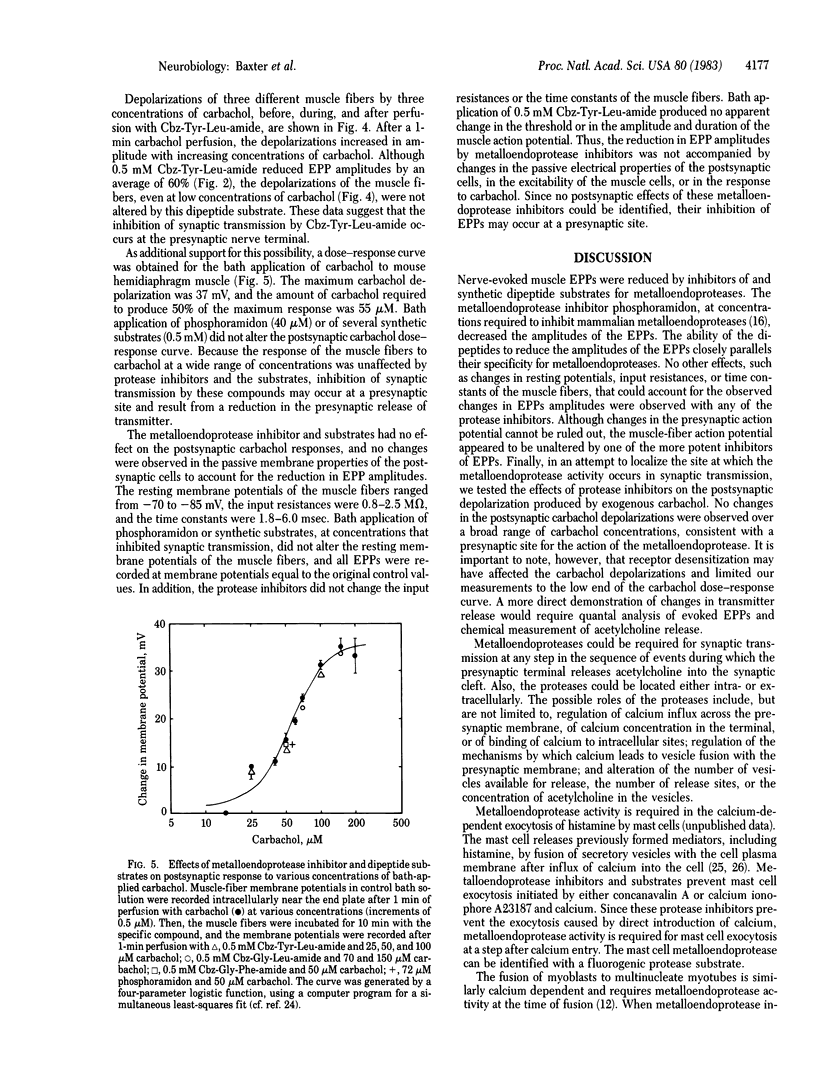
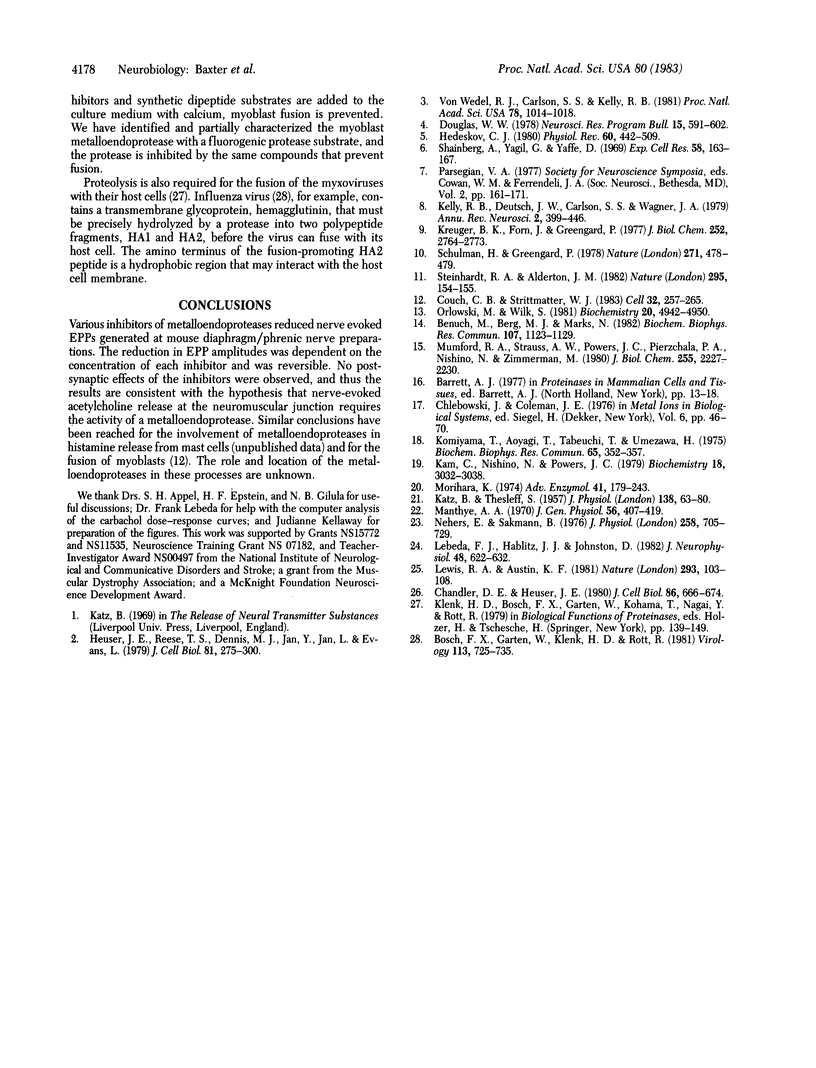
Selected References
These references are in PubMed. This may not be the complete list of references from this article.
- Benuck M., Berg M. J., Marks N. Rat brain and kidney metalloendopeptidase: enkephalin heptapeptide conversion to form a cardioactive neuropeptide, Phe-Met-Arg-Phe-amide. Biochem Biophys Res Commun. 1982 Aug;107(3):1123–1129. doi: 10.1016/0006-291x(82)90638-6. [DOI] [PubMed] [Google Scholar]
- Bosch F. X., Garten W., Klenk H. D., Rott R. Proteolytic cleavage of influenza virus hemagglutinins: primary structure of the connecting peptide between HA1 and HA2 determines proteolytic cleavability and pathogenicity of Avian influenza viruses. Virology. 1981 Sep;113(2):725–735. doi: 10.1016/0042-6822(81)90201-4. [DOI] [PubMed] [Google Scholar]
- Chandler D. E., Heuser J. E. Arrest of membrane fusion events in mast cells by quick-freezing. J Cell Biol. 1980 Aug;86(2):666–674. doi: 10.1083/jcb.86.2.666. [DOI] [PMC free article] [PubMed] [Google Scholar]
- Couch C. B., Strittmatter W. J. Rat myoblast fusion requires metalloendoprotease activity. Cell. 1983 Jan;32(1):257–265. doi: 10.1016/0092-8674(83)90516-0. [DOI] [PubMed] [Google Scholar]
- Hedeskov C. J. Mechanism of glucose-induced insulin secretion. Physiol Rev. 1980 Apr;60(2):442–509. doi: 10.1152/physrev.1980.60.2.442. [DOI] [PubMed] [Google Scholar]
- Heuser J. E., Reese T. S., Dennis M. J., Jan Y., Jan L., Evans L. Synaptic vesicle exocytosis captured by quick freezing and correlated with quantal transmitter release. J Cell Biol. 1979 May;81(2):275–300. doi: 10.1083/jcb.81.2.275. [DOI] [PMC free article] [PubMed] [Google Scholar]
- KATZ B., THESLEFF S. A study of the desensitization produced by acetylcholine at the motor end-plate. J Physiol. 1957 Aug 29;138(1):63–80. doi: 10.1113/jphysiol.1957.sp005838. [DOI] [PMC free article] [PubMed] [Google Scholar]
- Kam C. M., Nishino N., Powers J. C. Inhibition of thermolysin and carboxypeptidase A by phosphoramidates. Biochemistry. 1979 Jul 10;18(14):3032–3038. doi: 10.1021/bi00581a019. [DOI] [PubMed] [Google Scholar]
- Kelly R. B., Deutsch J. W., Carlson S. S., Wagner J. A. Biochemistry of neurotransmitter release. Annu Rev Neurosci. 1979;2:399–446. doi: 10.1146/annurev.ne.02.030179.002151. [DOI] [PubMed] [Google Scholar]
- Komiyama T., Aoyagi T., Takeuchi T., Umezawa H. Inhibitory effects of phosphoramidon on neutral metalloendopeptidases and its application on affinity chromatography. Biochem Biophys Res Commun. 1975 Jul 8;65(1):352–357. doi: 10.1016/s0006-291x(75)80100-8. [DOI] [PubMed] [Google Scholar]
- Krueger B. K., Forn J., Greengard P. Depolarization-induced phosphorylation of specific proteins, mediated by calcium ion influx, in rat brain synaptosomes. J Biol Chem. 1977 Apr 25;252(8):2764–2773. [PubMed] [Google Scholar]
- Lebeda F. J., Hablitz J. J., Johnston D. Antagonism of GABA-mediated responses by d-tubocurarine in hippocampal neurons. J Neurophysiol. 1982 Sep;48(3):622–632. doi: 10.1152/jn.1982.48.3.622. [DOI] [PubMed] [Google Scholar]
- Lewis R. A., Austen K. F. Mediation of local homeostasis and inflammation by leukotrienes and other mast cell-dependent compounds. Nature. 1981 Sep 10;293(5828):103–108. doi: 10.1038/293103a0. [DOI] [PubMed] [Google Scholar]
- Manthey A. A. Further studies of the effect of calcium on the time course of action of carbamylcholine at the neuromuscular junction. J Gen Physiol. 1970 Sep;56(3):407–419. doi: 10.1085/jgp.56.3.407. [DOI] [PMC free article] [PubMed] [Google Scholar]
- Morihara K. Comparative specificity of microbial proteinases. Adv Enzymol Relat Areas Mol Biol. 1974;41(0):179–243. doi: 10.1002/9780470122860.ch5. [DOI] [PubMed] [Google Scholar]
- Mumford R. A., Strauss A. W., Powers J. C., Pierzchala P. A., Nishino N., Zimmerman M. A zinc metalloendopeptidase associated with dog pancreatic membranes. J Biol Chem. 1980 Mar 25;255(6):2227–2230. [PubMed] [Google Scholar]
- Neher E., Sakmann B. Noise analysis of drug induced voltage clamp currents in denervated frog muscle fibres. J Physiol. 1976 Jul;258(3):705–729. doi: 10.1113/jphysiol.1976.sp011442. [DOI] [PMC free article] [PubMed] [Google Scholar]
- Orlowski M., Wilk S. Purification and specificity of a membrane-bound metalloendopeptidase from bovine pituitaries. Biochemistry. 1981 Aug 18;20(17):4942–4950. doi: 10.1021/bi00520a021. [DOI] [PubMed] [Google Scholar]
- Schulman H., Greengard P. Stimulation of brain membrane protein phosphorylation by calcium and an endogenous heat-stable protein. Nature. 1978 Feb 2;271(5644):478–479. doi: 10.1038/271478a0. [DOI] [PubMed] [Google Scholar]
- Shainberg A., Yagil G., Yaffe D. Control of myogenesis in vitro by Ca 2 + concentration in nutritional medium. Exp Cell Res. 1969 Nov;58(1):163–167. doi: 10.1016/0014-4827(69)90127-x. [DOI] [PubMed] [Google Scholar]
- Steinhardt R. A., Alderton J. M. Calmodulin confers calcium sensitivity on secretory exocytosis. Nature. 1982 Jan 14;295(5845):154–155. doi: 10.1038/295154a0. [DOI] [PubMed] [Google Scholar]
- von Wedel R. J., Carlson S. S., Kelly R. B. Transfer of synaptic vesicle antigens to the presynaptic plasma membrane during exocytosis. Proc Natl Acad Sci U S A. 1981 Feb;78(2):1014–1018. doi: 10.1073/pnas.78.2.1014. [DOI] [PMC free article] [PubMed] [Google Scholar]