Abstract
The UDP-Glc:glycoprotein glucosyltransferase is a soluble enzyme of the endoplasmic reticulum that glucosylates protein-linked Man7-9GlcNAc2 to form the monoglucosylated derivatives. In vivo the reaction products are immediately deglucosylated by glucosidase II. The glucosyltransferase has a unique property: it glucosylates misfolded, but not native, glycoproteins. It has been proposed that the glucosyltransferase participates, together with calnexin, in the control mechanism by which only properly folded glycoproteins can exit from the endoplasmic reticulum. In this paper it is demonstrated that the glucosyltransferase recognizes two elements in the acceptor substrates: the innermost N-acetylglucosamine unit of the oligosaccharide and protein domains exposed in denatured, but not in native, conformations. Both determinants have to be covalently linked. In many cases the first element is not accessible to macromolecular probes in native conformations. Concerning the protein domains, it is demonstrated here that the glucosyltransferase interacts with hydrophobic amino acids exposed in denatured conformations. More disordered conformations, i.e. those exposing more hydrophobic amino acids, were found to be those having higher glucose acceptor capacity. It is suggested that both accessibility of the innermost N-acetylglucosamine unit and binding to hydrophobic patches determine the exclusive glucosylation of misfolded conformations by the glucosyltransferase.
Full text
PDF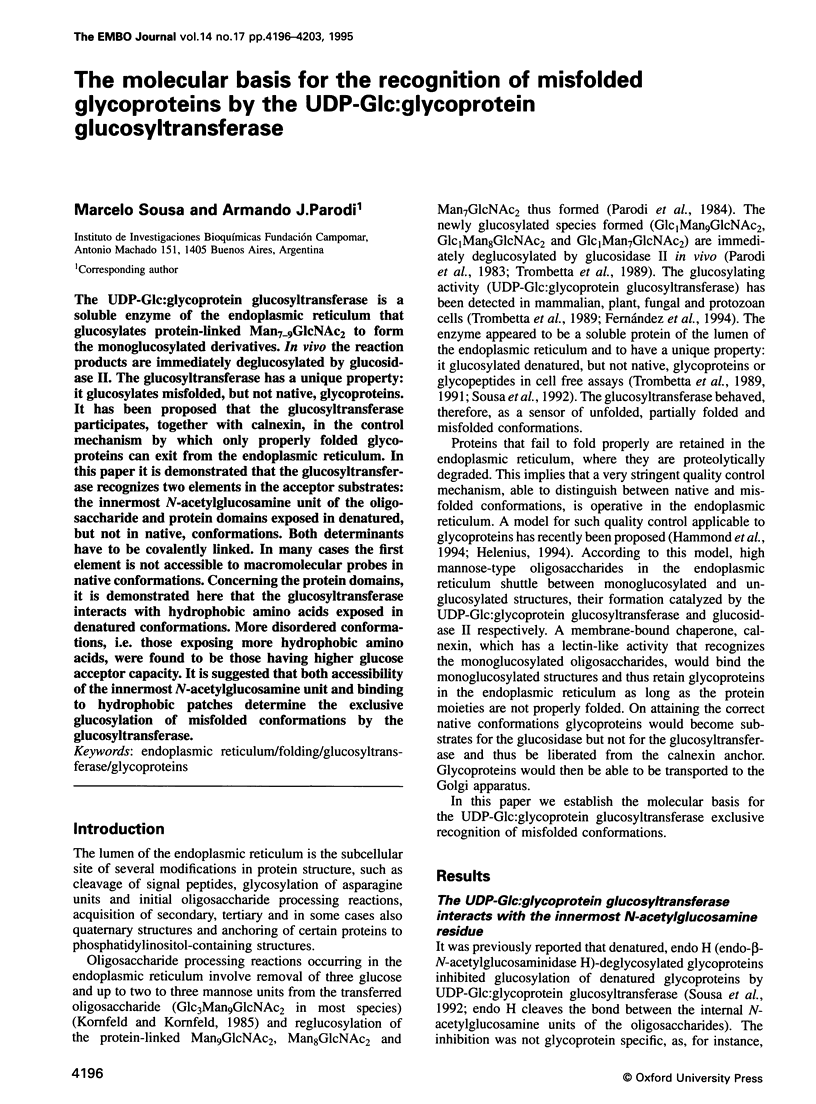
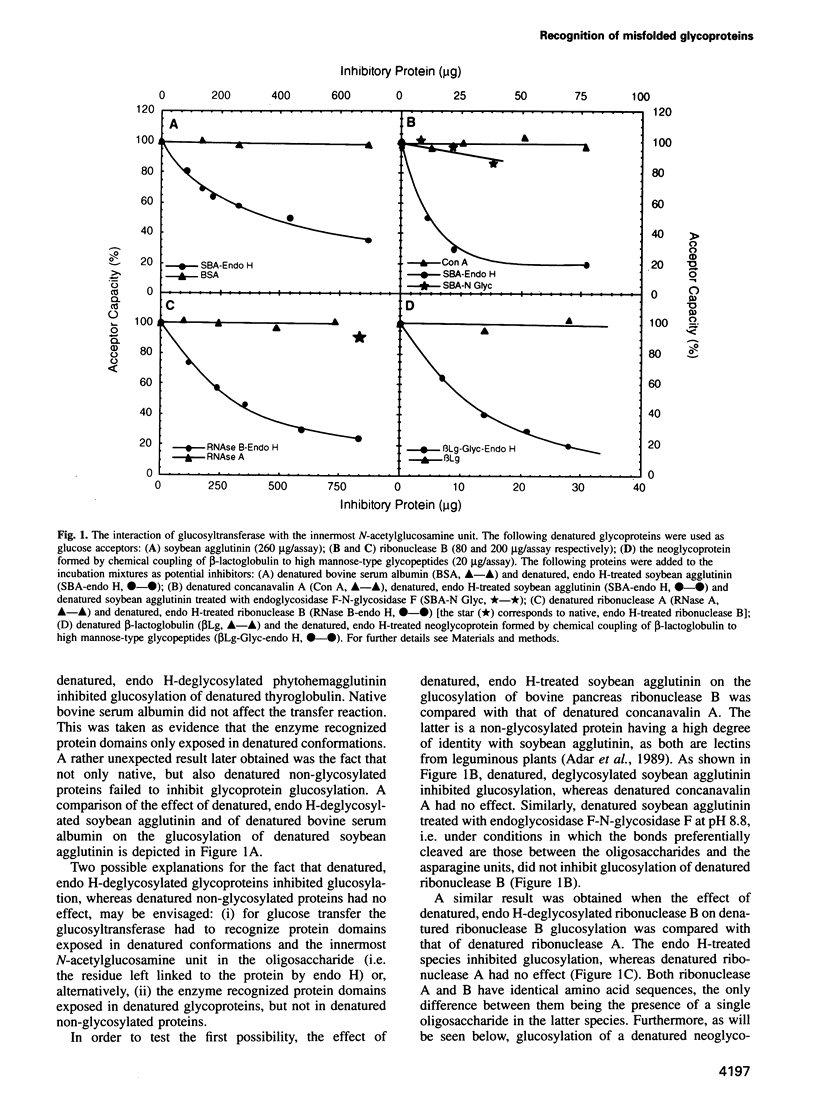
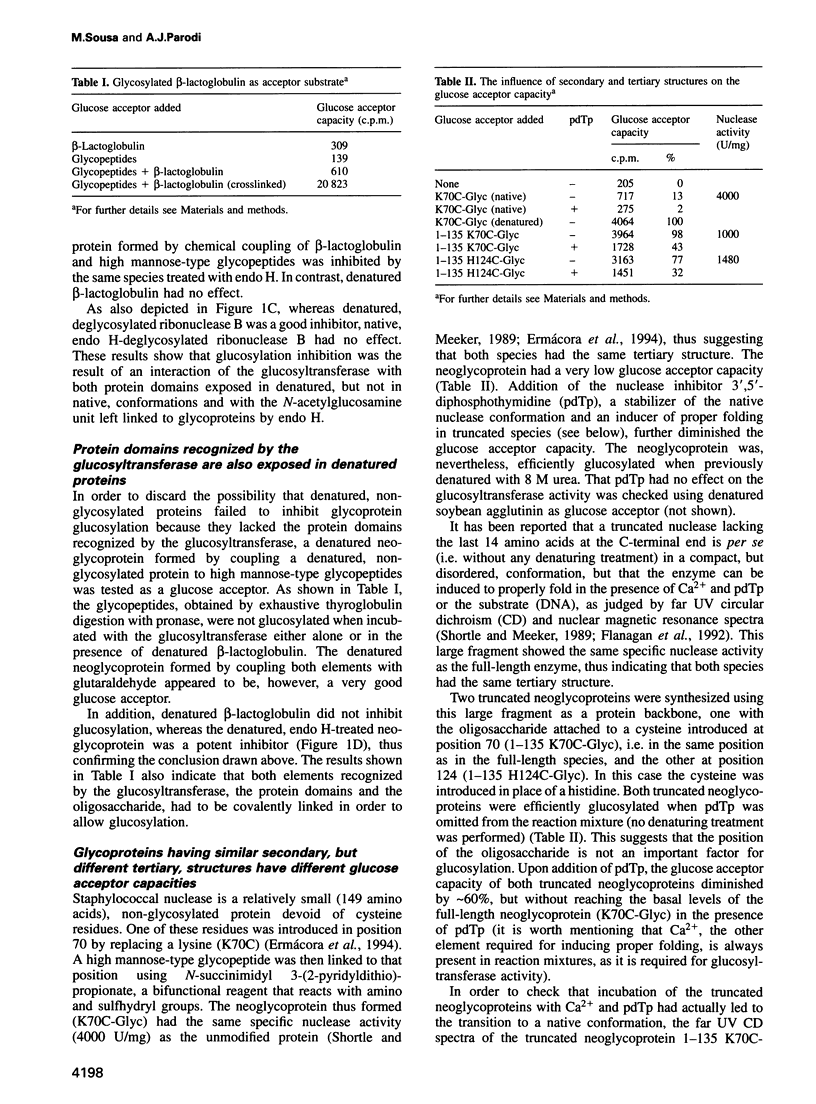
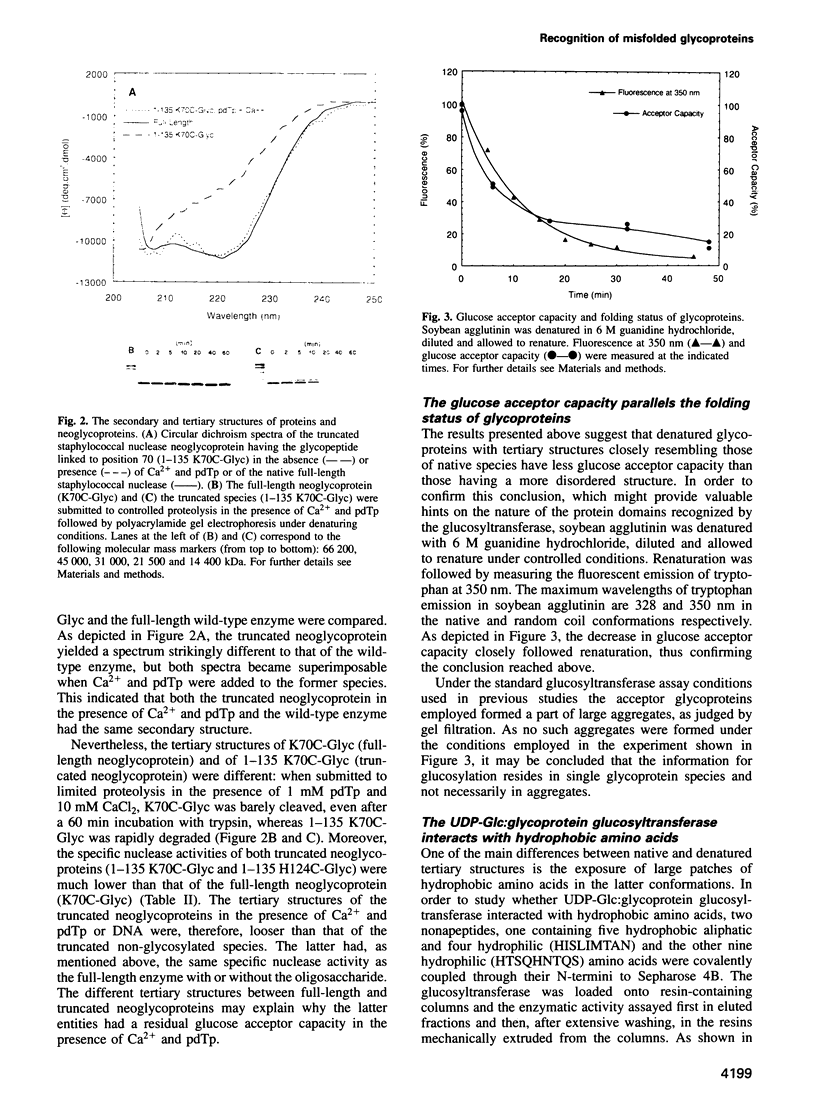
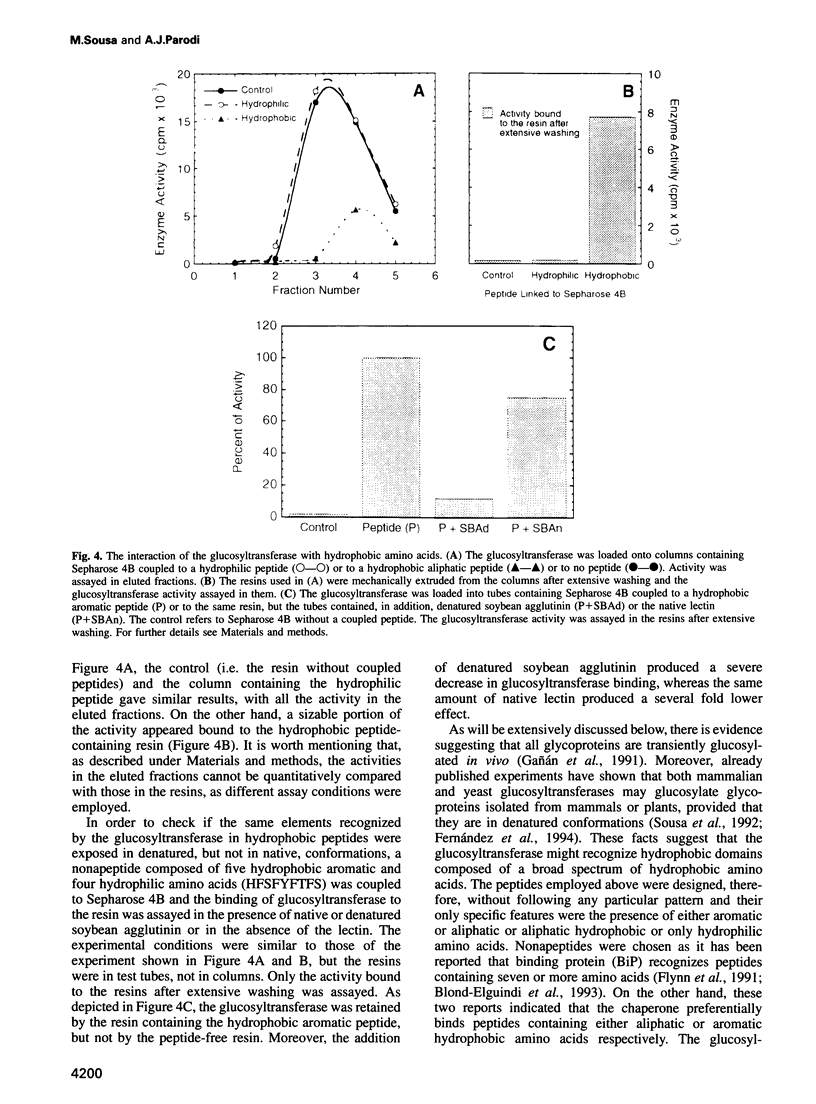
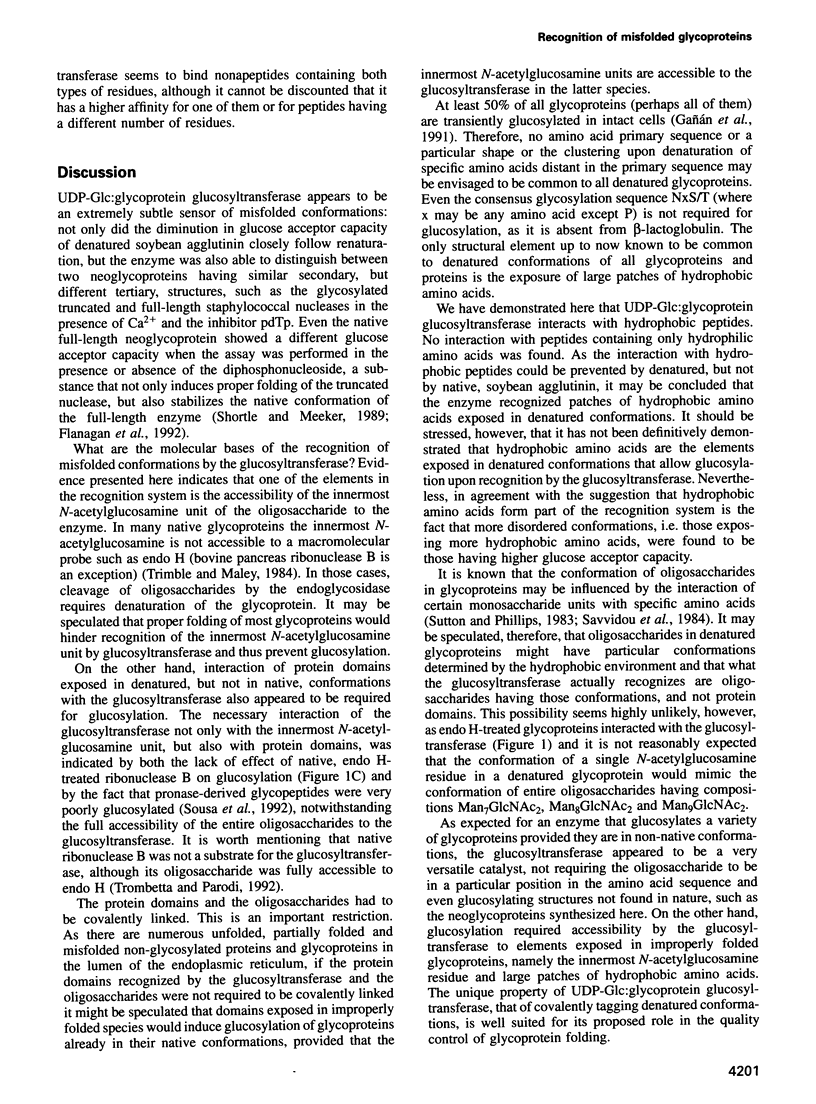
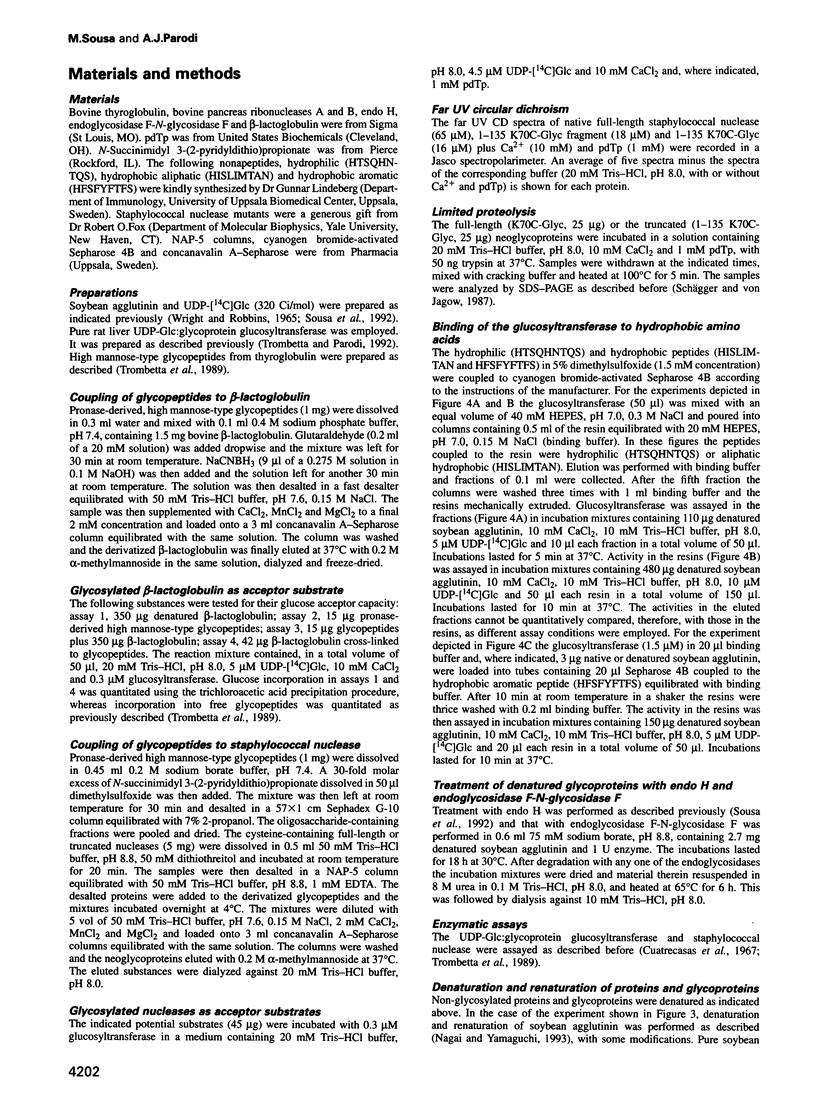
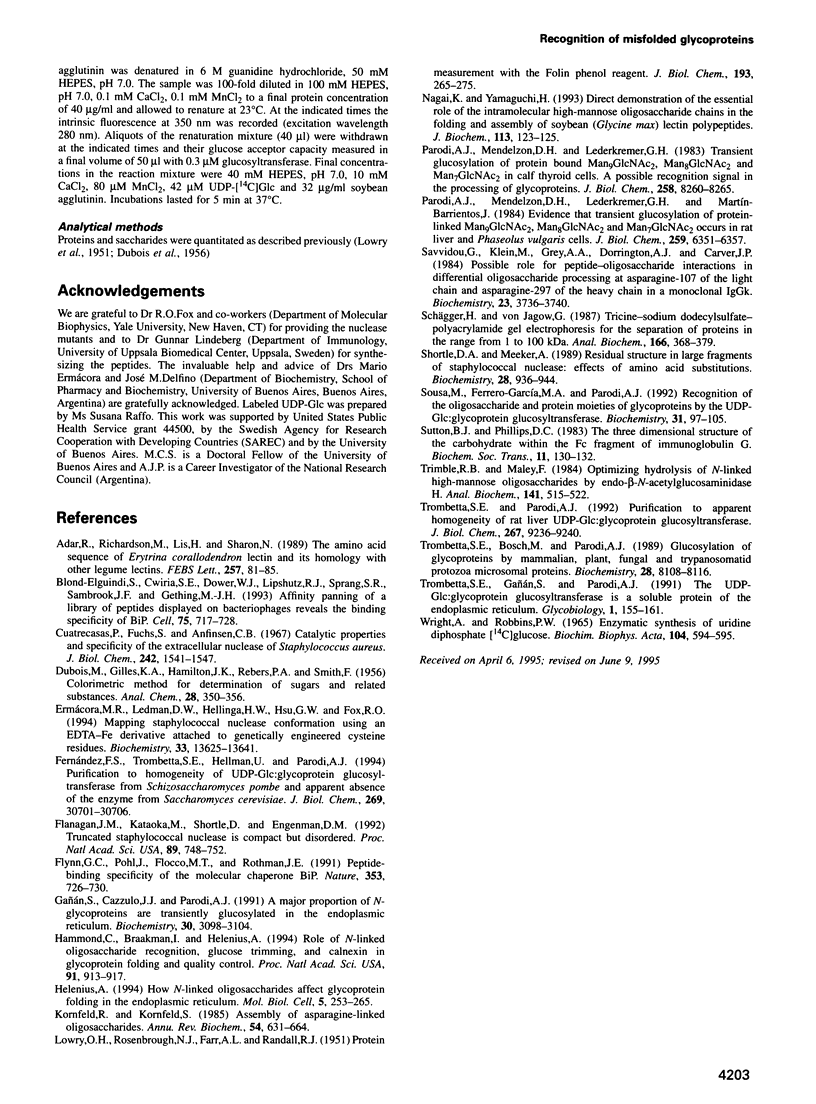
Images in this article
Selected References
These references are in PubMed. This may not be the complete list of references from this article.
- Adar R., Richardson M., Lis H., Sharon N. The amino acid sequence of Erythrina corallodendron lectin and its homology with other legume lectins. FEBS Lett. 1989 Oct 23;257(1):81–85. doi: 10.1016/0014-5793(89)81791-0. [DOI] [PubMed] [Google Scholar]
- Blond-Elguindi S., Cwirla S. E., Dower W. J., Lipshutz R. J., Sprang S. R., Sambrook J. F., Gething M. J. Affinity panning of a library of peptides displayed on bacteriophages reveals the binding specificity of BiP. Cell. 1993 Nov 19;75(4):717–728. doi: 10.1016/0092-8674(93)90492-9. [DOI] [PubMed] [Google Scholar]
- Cuatrecasas P., Fuchs S., Anfinsen C. B. Catalytic properties and specificity of the extracellular nuclease of Staphylococcus aureus. J Biol Chem. 1967 Apr 10;242(7):1541–1547. [PubMed] [Google Scholar]
- Ermácora M. R., Ledman D. W., Hellinga H. W., Hsu G. W., Fox R. O. Mapping staphylococcal nuclease conformation using an EDTA-Fe derivative attached to genetically engineered cysteine residues. Biochemistry. 1994 Nov 22;33(46):13625–13641. doi: 10.1021/bi00250a013. [DOI] [PubMed] [Google Scholar]
- Fernández F. S., Trombetta S. E., Hellman U., Parodi A. J. Purification to homogeneity of UDP-glucose:glycoprotein glucosyltransferase from Schizosaccharomyces pombe and apparent absence of the enzyme fro Saccharomyces cerevisiae. J Biol Chem. 1994 Dec 2;269(48):30701–30706. [PubMed] [Google Scholar]
- Flanagan J. M., Kataoka M., Shortle D., Engelman D. M. Truncated staphylococcal nuclease is compact but disordered. Proc Natl Acad Sci U S A. 1992 Jan 15;89(2):748–752. doi: 10.1073/pnas.89.2.748. [DOI] [PMC free article] [PubMed] [Google Scholar]
- Flynn G. C., Pohl J., Flocco M. T., Rothman J. E. Peptide-binding specificity of the molecular chaperone BiP. Nature. 1991 Oct 24;353(6346):726–730. doi: 10.1038/353726a0. [DOI] [PubMed] [Google Scholar]
- Gañn S., Cazzulo J. J., Parodi A. J. A major proportion of N-glycoproteins are transiently glucosylated in the endoplasmic reticulum. Biochemistry. 1991 Mar 26;30(12):3098–3104. doi: 10.1021/bi00226a017. [DOI] [PubMed] [Google Scholar]
- Hammond C., Braakman I., Helenius A. Role of N-linked oligosaccharide recognition, glucose trimming, and calnexin in glycoprotein folding and quality control. Proc Natl Acad Sci U S A. 1994 Feb 1;91(3):913–917. doi: 10.1073/pnas.91.3.913. [DOI] [PMC free article] [PubMed] [Google Scholar]
- Helenius A. How N-linked oligosaccharides affect glycoprotein folding in the endoplasmic reticulum. Mol Biol Cell. 1994 Mar;5(3):253–265. doi: 10.1091/mbc.5.3.253. [DOI] [PMC free article] [PubMed] [Google Scholar]
- Kornfeld R., Kornfeld S. Assembly of asparagine-linked oligosaccharides. Annu Rev Biochem. 1985;54:631–664. doi: 10.1146/annurev.bi.54.070185.003215. [DOI] [PubMed] [Google Scholar]
- Nagai K., Yamaguchi H. Direct demonstration of the essential role of the intramolecular high-mannose oligosaccharide chains in the folding and assembly of soybean (glycine max) lectin polypeptides. J Biochem. 1993 Feb;113(2):123–125. doi: 10.1093/oxfordjournals.jbchem.a124012. [DOI] [PubMed] [Google Scholar]
- Parodi A. J., Mendelzon D. H., Lederkremer G. Z., Martin-Barrientos J. Evidence that transient glucosylation of protein-linked Man9GlcNAc2, Man8GlcNAc2, and Man7GlcNAc2 occurs in rat liver and Phaseolus vulgaris cells. J Biol Chem. 1984 May 25;259(10):6351–6357. [PubMed] [Google Scholar]
- Parodi A. J., Mendelzon D. H., Lederkremer G. Z. Transient glucosylation of protein-bound Man9GlcNAc2, Man8GlcNAc2, and Man7GlcNAc2 in calf thyroid cells. A possible recognition signal in the processing of glycoproteins. J Biol Chem. 1983 Jul 10;258(13):8260–8265. [PubMed] [Google Scholar]
- Savvidou G., Klein M., Grey A. A., Dorrington K. J., Carver J. P. Possible role for peptide-oligosaccharide interactions in differential oligosaccharide processing at asparagine-107 of the light chain and asparagine-297 of the heavy chain in a monoclonal IgG1 kappa. Biochemistry. 1984 Jul 31;23(16):3736–3740. doi: 10.1021/bi00311a026. [DOI] [PubMed] [Google Scholar]
- Schägger H., von Jagow G. Tricine-sodium dodecyl sulfate-polyacrylamide gel electrophoresis for the separation of proteins in the range from 1 to 100 kDa. Anal Biochem. 1987 Nov 1;166(2):368–379. doi: 10.1016/0003-2697(87)90587-2. [DOI] [PubMed] [Google Scholar]
- Shortle D., Meeker A. K. Residual structure in large fragments of staphylococcal nuclease: effects of amino acid substitutions. Biochemistry. 1989 Feb 7;28(3):936–944. doi: 10.1021/bi00429a003. [DOI] [PubMed] [Google Scholar]
- Sousa M. C., Ferrero-Garcia M. A., Parodi A. J. Recognition of the oligosaccharide and protein moieties of glycoproteins by the UDP-Glc:glycoprotein glucosyltransferase. Biochemistry. 1992 Jan 14;31(1):97–105. doi: 10.1021/bi00116a015. [DOI] [PubMed] [Google Scholar]
- Sutton B. J., Phillips D. C. The three-dimensional structure of the carbohydrate within the Fc fragment of immunoglobulin G. Biochem Soc Trans. 1983 Apr;11(Pt 2):130–132. [PubMed] [Google Scholar]
- Trimble R. B., Maley F. Optimizing hydrolysis of N-linked high-mannose oligosaccharides by endo-beta-N-acetylglucosaminidase H. Anal Biochem. 1984 Sep;141(2):515–522. doi: 10.1016/0003-2697(84)90080-0. [DOI] [PubMed] [Google Scholar]
- Trombetta S. E., Bosch M., Parodi A. J. Glucosylation of glycoproteins by mammalian, plant, fungal, and trypanosomatid protozoa microsomal membranes. Biochemistry. 1989 Oct 3;28(20):8108–8116. doi: 10.1021/bi00446a022. [DOI] [PubMed] [Google Scholar]
- Trombetta S. E., Gañan S. A., Parodi A. J. The UDP-Glc:glycoprotein glucosyltransferase is a soluble protein of the endoplasmic reticulum. Glycobiology. 1991 Mar;1(2):155–161. doi: 10.1093/glycob/1.2.155. [DOI] [PubMed] [Google Scholar]
- Trombetta S. E., Parodi A. J. Purification to apparent homogeneity and partial characterization of rat liver UDP-glucose:glycoprotein glucosyltransferase. J Biol Chem. 1992 May 5;267(13):9236–9240. [PubMed] [Google Scholar]