Abstract
The maltose transport system of Escherichia coli is a well-characterized member of the ATP binding cassette transporter superfamily. Members of this family share sequence similarity surrounding two short sequences (the Walker A and B sequences) which constitute a nucleotide binding pocket. It is likely that the energy from binding and hydrolysis of ATP is used to accomplish the translocation of substrate from one location to another. Periplasmic binding protein-dependent transport systems, like the maltose transport system of E.coli, possess a water-soluble ligand binding protein that is essential for transport activity. In addition to delivering ligand to the membrane-bound components of the system on the external face of the membrane, the interaction of the binding protein with the membrane complex initiates a signal that is transmitted to the ATP binding subunit on the cytosolic side and stimulates its hydrolytic activity. Mutations that alter the membrane complex so that it transports independently of the periplasmic binding protein also result in constitutive activation of the ATPase. Genetic analysis indicates that, in general, two mutations are required for binding protein-independent transport and constitutive ATPase. The mutations alter residues that cluster to specific regions within the membrane spanning segments of the integral membrane components MalF and MalG. Individually, the mutations perturb the ability of MBP to interact productively with the membrane complex. Genetic alteration of this signalling pathway suggests that other agents might have similar effects. These could be potentially useful for modulating the activities of ABC transporters such as P-glycoprotein or CFTR, that are implicated in disease.
Full text
PDF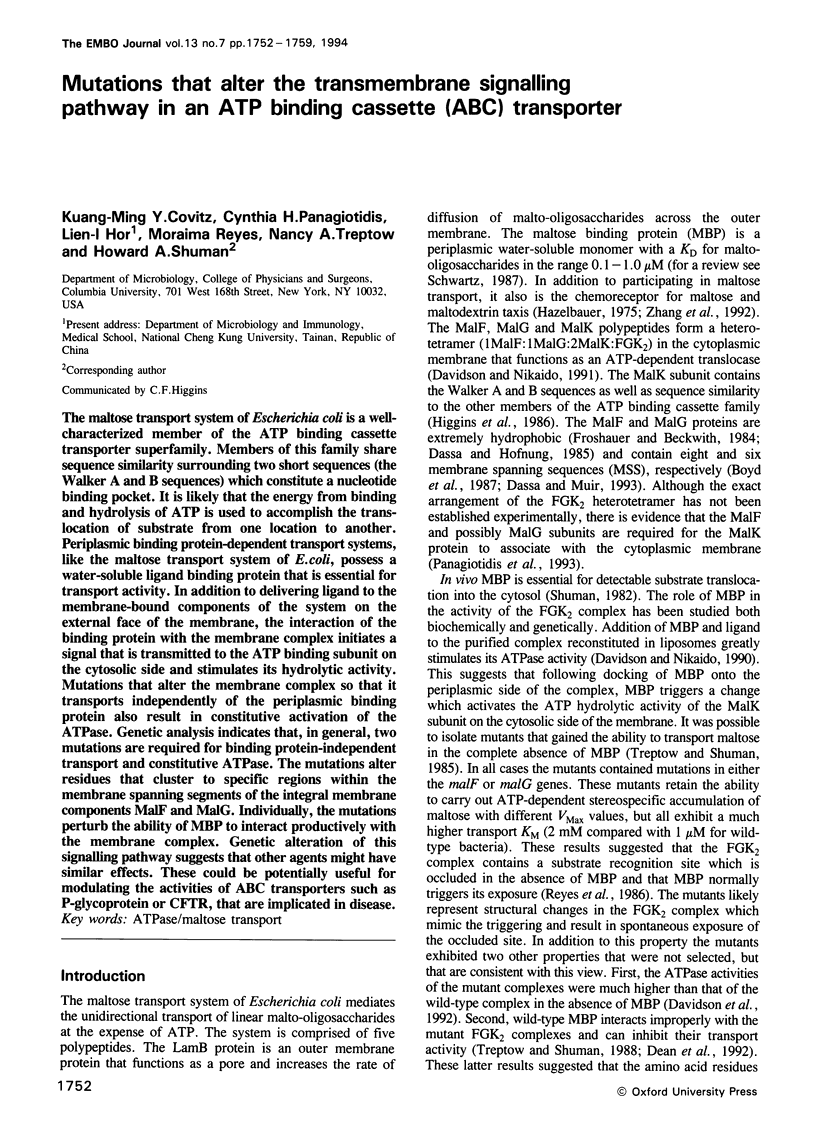
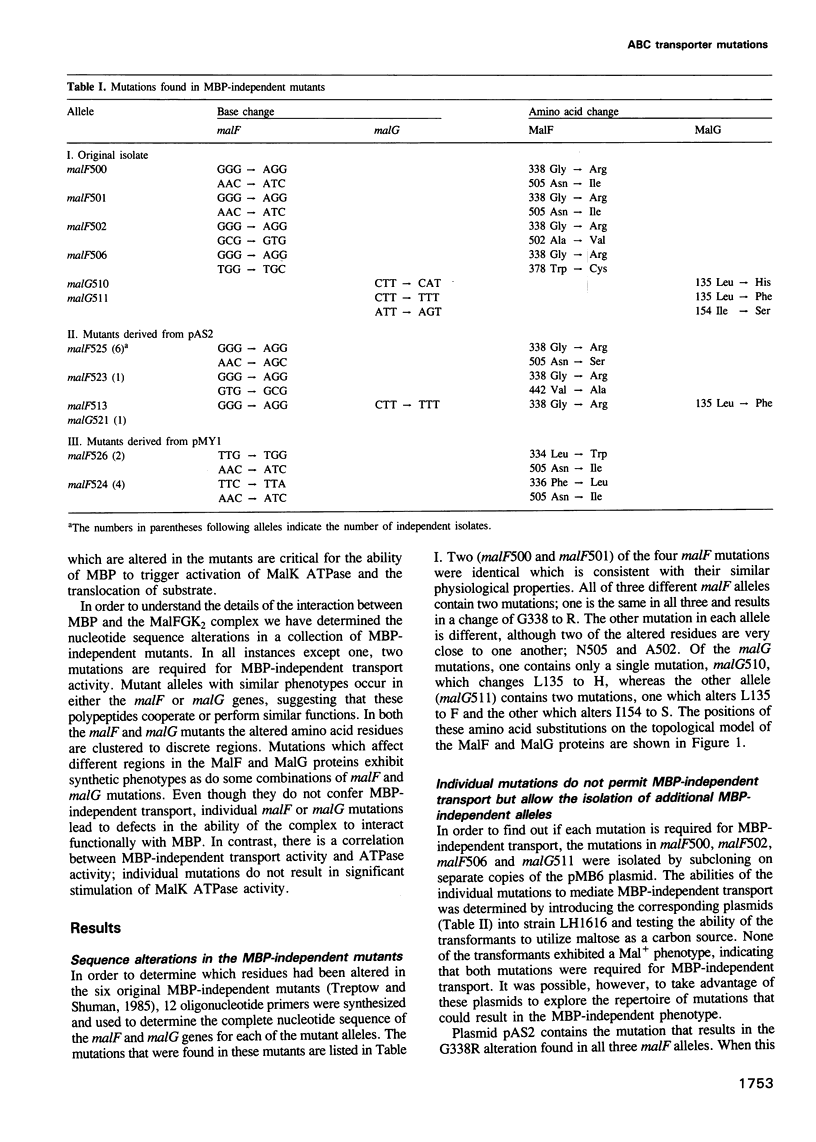
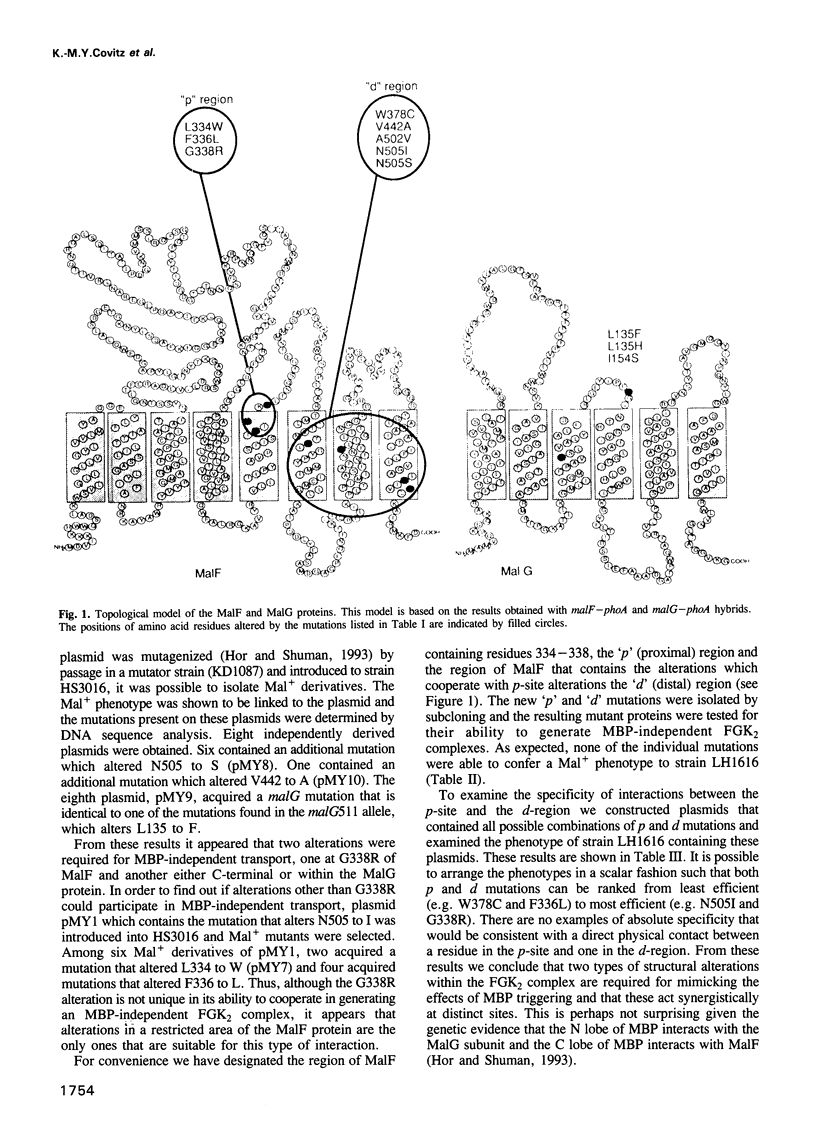
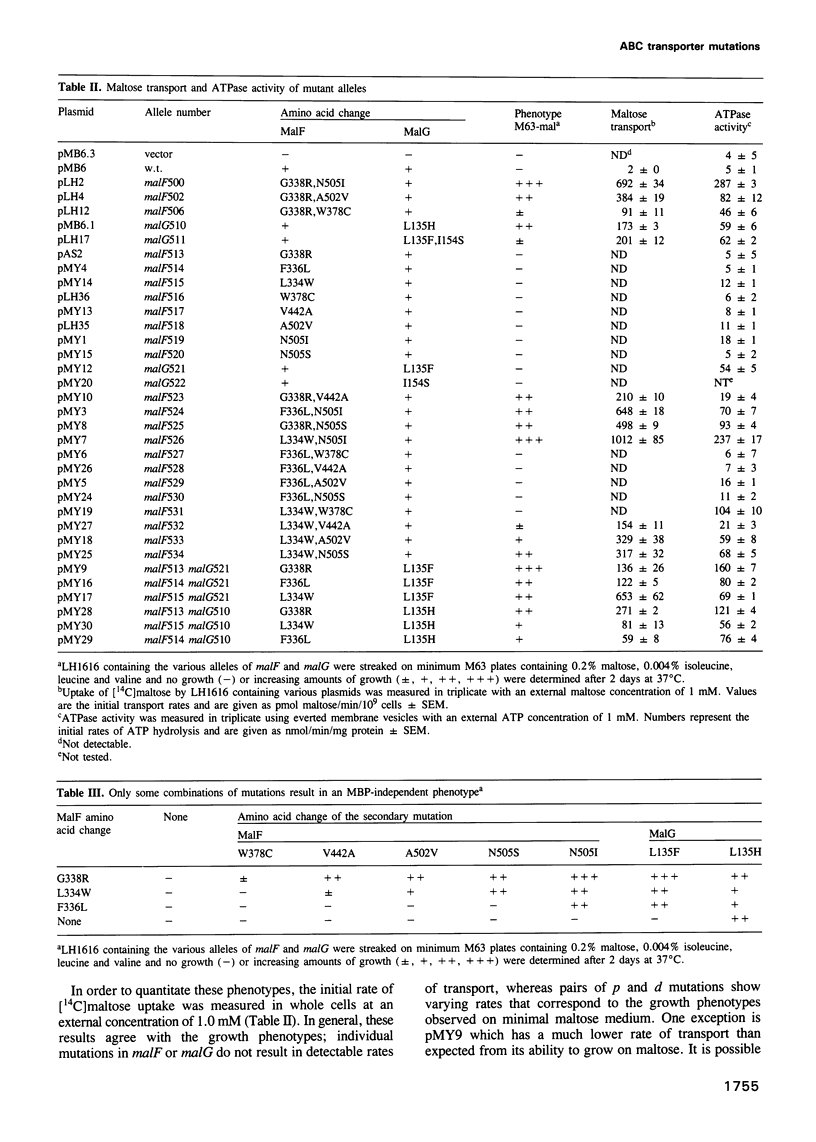
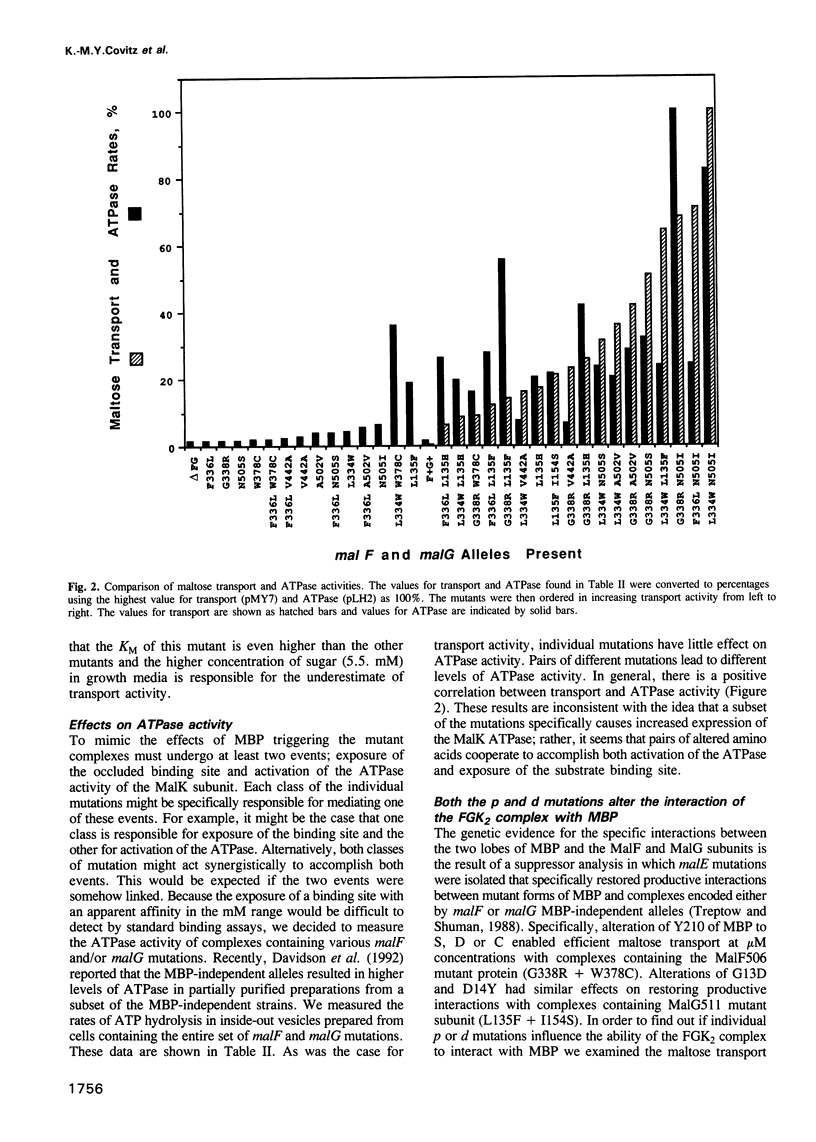
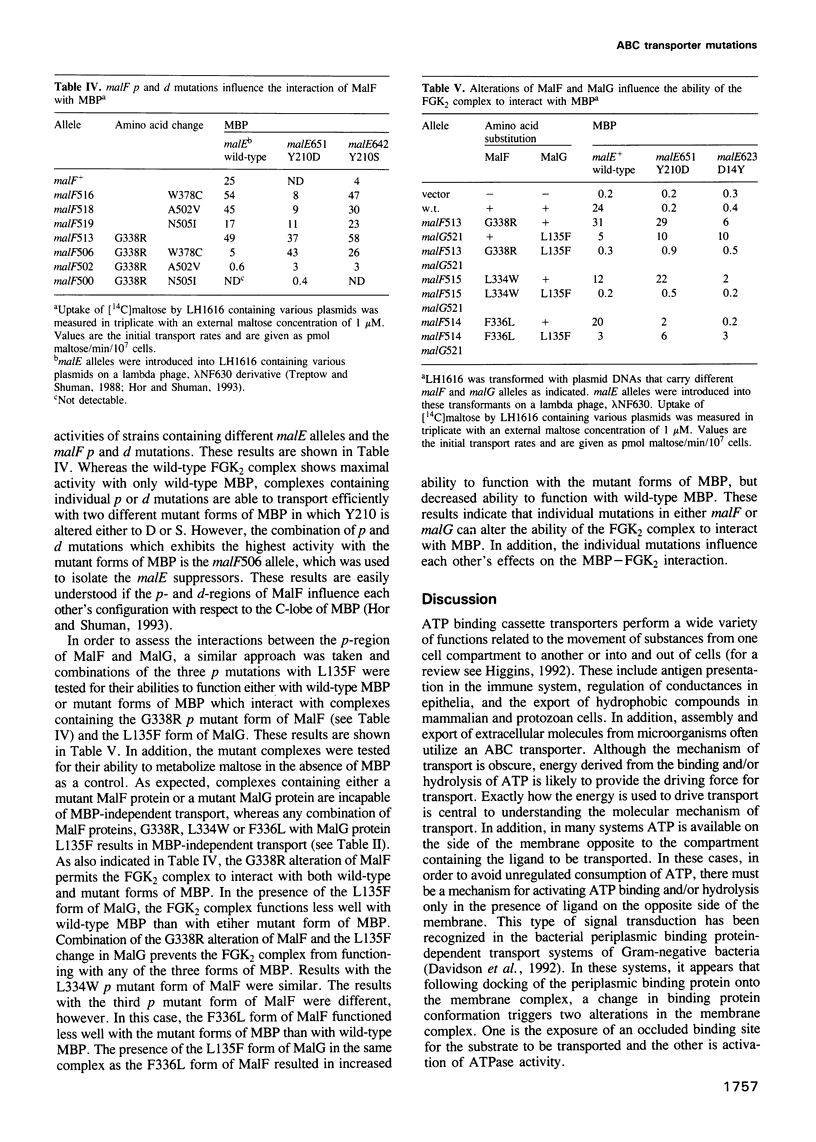
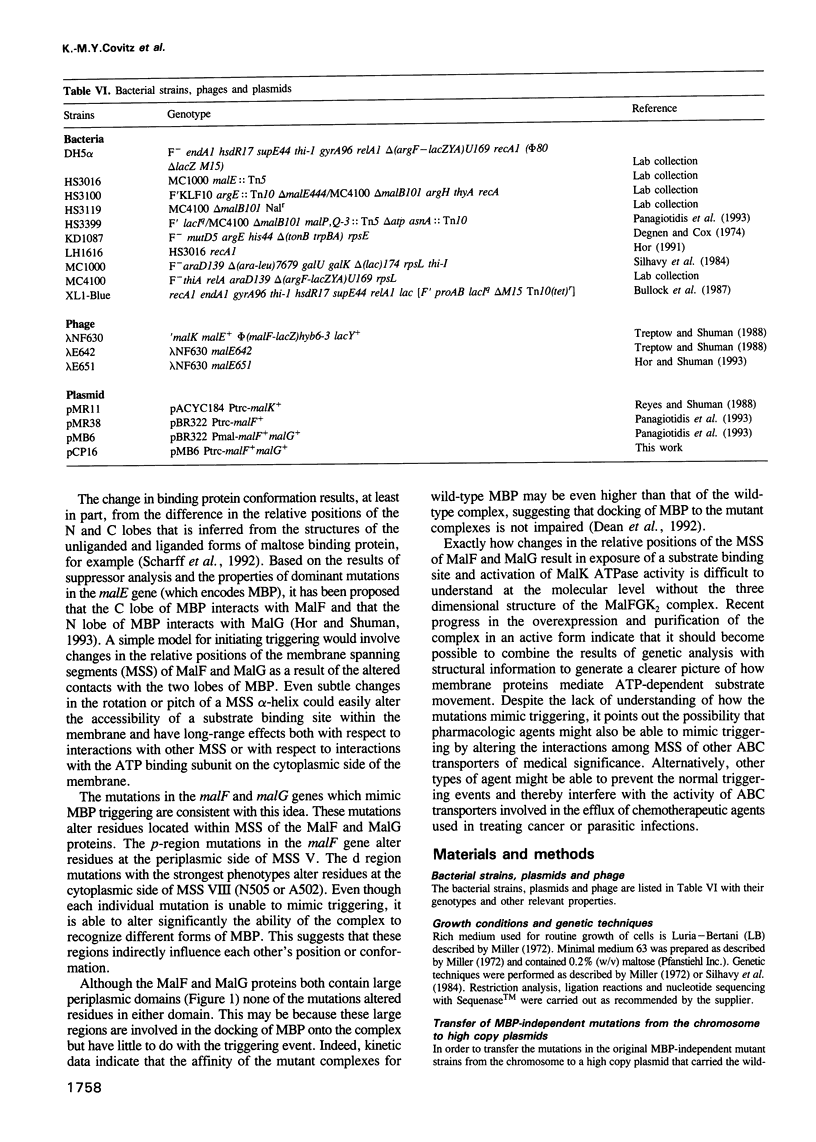
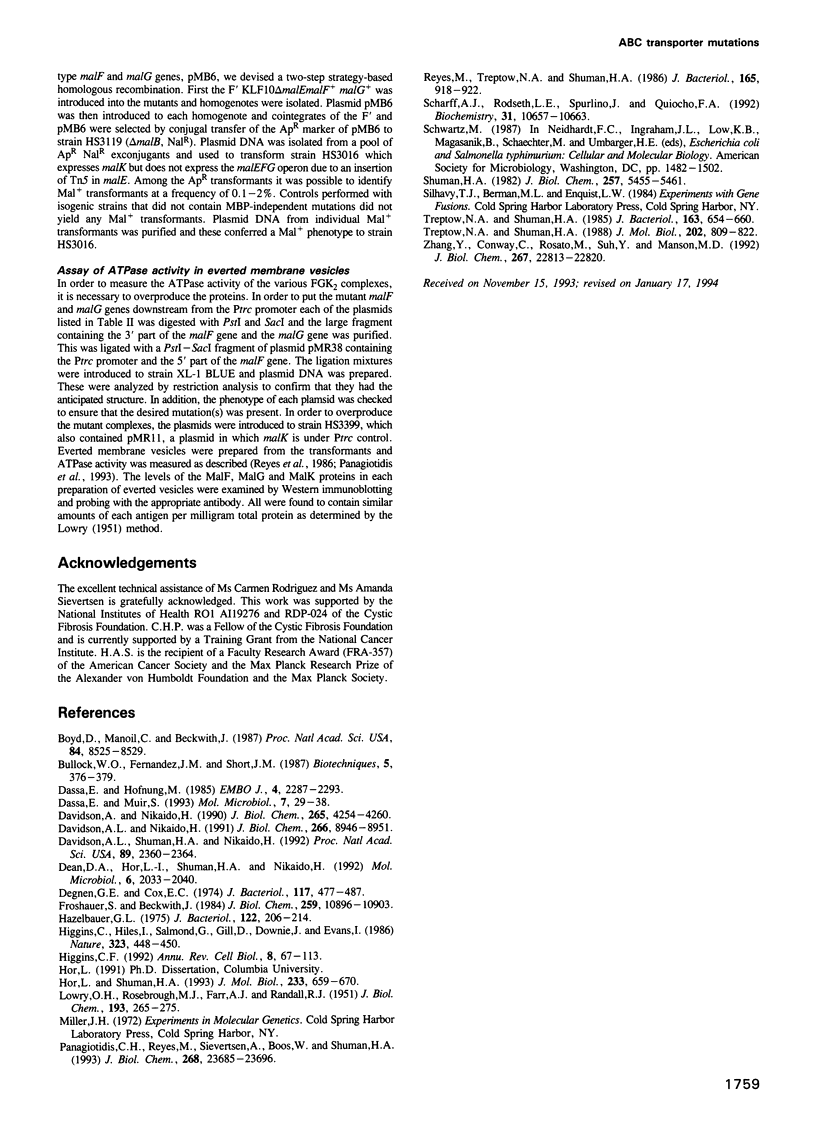
Images in this article
Selected References
These references are in PubMed. This may not be the complete list of references from this article.
- Boyd D., Manoil C., Beckwith J. Determinants of membrane protein topology. Proc Natl Acad Sci U S A. 1987 Dec;84(23):8525–8529. doi: 10.1073/pnas.84.23.8525. [DOI] [PMC free article] [PubMed] [Google Scholar]
- Dassa E., Hofnung M. Sequence of gene malG in E. coli K12: homologies between integral membrane components from binding protein-dependent transport systems. EMBO J. 1985 Sep;4(9):2287–2293. doi: 10.1002/j.1460-2075.1985.tb03928.x. [DOI] [PMC free article] [PubMed] [Google Scholar]
- Dassa E., Muir S. Membrane topology of MalG, an inner membrane protein from the maltose transport system of Escherichia coli. Mol Microbiol. 1993 Jan;7(1):29–38. doi: 10.1111/j.1365-2958.1993.tb01094.x. [DOI] [PubMed] [Google Scholar]
- Davidson A. L., Nikaido H. Overproduction, solubilization, and reconstitution of the maltose transport system from Escherichia coli. J Biol Chem. 1990 Mar 15;265(8):4254–4260. [PubMed] [Google Scholar]
- Davidson A. L., Nikaido H. Purification and characterization of the membrane-associated components of the maltose transport system from Escherichia coli. J Biol Chem. 1991 May 15;266(14):8946–8951. [PubMed] [Google Scholar]
- Davidson A. L., Shuman H. A., Nikaido H. Mechanism of maltose transport in Escherichia coli: transmembrane signaling by periplasmic binding proteins. Proc Natl Acad Sci U S A. 1992 Mar 15;89(6):2360–2364. doi: 10.1073/pnas.89.6.2360. [DOI] [PMC free article] [PubMed] [Google Scholar]
- Dean D. A., Hor L. I., Shuman H. A., Nikaido H. Interaction between maltose-binding protein and the membrane-associated maltose transporter complex in Escherichia coli. Mol Microbiol. 1992 Aug;6(15):2033–2040. doi: 10.1111/j.1365-2958.1992.tb01376.x. [DOI] [PubMed] [Google Scholar]
- Degnen G. E., Cox E. C. Conditional mutator gene in Escherichia coli: isolation, mapping, and effector studies. J Bacteriol. 1974 Feb;117(2):477–487. doi: 10.1128/jb.117.2.477-487.1974. [DOI] [PMC free article] [PubMed] [Google Scholar]
- Froshauer S., Beckwith J. The nucleotide sequence of the gene for malF protein, an inner membrane component of the maltose transport system of Escherichia coli. Repeated DNA sequences are found in the malE-malF intercistronic region. J Biol Chem. 1984 Sep 10;259(17):10896–10903. [PubMed] [Google Scholar]
- Hazelbauer G. L. Maltose chemoreceptor of Escherichia coli. J Bacteriol. 1975 Apr;122(1):206–214. doi: 10.1128/jb.122.1.206-214.1975. [DOI] [PMC free article] [PubMed] [Google Scholar]
- Higgins C. F. ABC transporters: from microorganisms to man. Annu Rev Cell Biol. 1992;8:67–113. doi: 10.1146/annurev.cb.08.110192.000435. [DOI] [PubMed] [Google Scholar]
- Higgins C. F., Hiles I. D., Salmond G. P., Gill D. R., Downie J. A., Evans I. J., Holland I. B., Gray L., Buckel S. D., Bell A. W. A family of related ATP-binding subunits coupled to many distinct biological processes in bacteria. Nature. 1986 Oct 2;323(6087):448–450. doi: 10.1038/323448a0. [DOI] [PubMed] [Google Scholar]
- Hor L. I., Shuman H. A. Genetic analysis of periplasmic binding protein dependent transport in Escherichia coli. Each lobe of maltose-binding protein interacts with a different subunit of the MalFGK2 membrane transport complex. J Mol Biol. 1993 Oct 20;233(4):659–670. doi: 10.1006/jmbi.1993.1543. [DOI] [PubMed] [Google Scholar]
- LOWRY O. H., ROSEBROUGH N. J., FARR A. L., RANDALL R. J. Protein measurement with the Folin phenol reagent. J Biol Chem. 1951 Nov;193(1):265–275. [PubMed] [Google Scholar]
- Panagiotidis C. H., Reyes M., Sievertsen A., Boos W., Shuman H. A. Characterization of the structural requirements for assembly and nucleotide binding of an ATP-binding cassette transporter. The maltose transport system of Escherichia coli. J Biol Chem. 1993 Nov 5;268(31):23685–23696. [PubMed] [Google Scholar]
- Reyes M., Treptow N. A., Shuman H. A. Transport of p-nitrophenyl-alpha-maltoside by the maltose transport system of Escherichia coli and its subsequent hydrolysis by a cytoplasmic alpha-maltosidase. J Bacteriol. 1986 Mar;165(3):918–922. doi: 10.1128/jb.165.3.918-922.1986. [DOI] [PMC free article] [PubMed] [Google Scholar]
- Sharff A. J., Rodseth L. E., Spurlino J. C., Quiocho F. A. Crystallographic evidence of a large ligand-induced hinge-twist motion between the two domains of the maltodextrin binding protein involved in active transport and chemotaxis. Biochemistry. 1992 Nov 10;31(44):10657–10663. doi: 10.1021/bi00159a003. [DOI] [PubMed] [Google Scholar]
- Shuman H. A. Active transport of maltose in Escherichia coli K12. Role of the periplasmic maltose-binding protein and evidence for a substrate recognition site in the cytoplasmic membrane. J Biol Chem. 1982 May 25;257(10):5455–5461. [PubMed] [Google Scholar]
- Treptow N. A., Shuman H. A. Allele-specific malE mutations that restore interactions between maltose-binding protein and the inner-membrane components of the maltose transport system. J Mol Biol. 1988 Aug 20;202(4):809–822. doi: 10.1016/0022-2836(88)90560-8. [DOI] [PubMed] [Google Scholar]
- Treptow N. A., Shuman H. A. Genetic evidence for substrate and periplasmic-binding-protein recognition by the MalF and MalG proteins, cytoplasmic membrane components of the Escherichia coli maltose transport system. J Bacteriol. 1985 Aug;163(2):654–660. doi: 10.1128/jb.163.2.654-660.1985. [DOI] [PMC free article] [PubMed] [Google Scholar]
- Zhang Y., Conway C., Rosato M., Suh Y., Manson M. D. Maltose chemotaxis involves residues in the N-terminal and C-terminal domains on the same face of maltose-binding protein. J Biol Chem. 1992 Nov 15;267(32):22813–22820. [PubMed] [Google Scholar]