Abstract
Phosphoglycolate and phosphoglycerate phosphatases and glycolate dehydrogenase activities were determined in division synchronized Euglena gracilis strain Z cultures. Phosphoglycolate phosphatase activity remained nearly constant in the light but doubled in the dark, whereas phosphoglycerate phosphatase activity decreased by half in the light and increased 4-fold over the dark phase of the cycle. Glycolate dehydrogenase activity assayed by dye reduction increased over the light and remained constant during the dark phase, but when determined by the phenylhydrazone method, an assay dependent upon the presence of a natural hydrogen acceptor, activity decreased in the dark phase. The acceptor decayed in the dark in all cell-free extracts and 3-(3,4-dichlorophenyl)-1, 1-dimethylurea inhibited light regeneration.
Enzyme activity regulated flow of carbon via the glycolate pathway; with cells at end of light phase and early dark phase, high levels of glycolate dehydrogenase activity were accompanied by an early and rapid labeling of glycerate. Degradation of early samples gave uniformly labeled glycolate and glycerate while phosphoglycerate was predominantly carboxyl labeled. At these stages in the division cycle, glycerate was formed from glycolate via the glycolate pathway, all the enzymes of the pathway being recorded in Euglena. In contrast to cells from end of dark phase and early light phase, times of maximum phosphoglycerate phosphatase activity, glycerate was predominantly carboxyl labeled as was phosphoglycerate, but glycolate was still uniformly labeled.
Glycolate excretion varied over the cycle, being maximal at end of dark phase, decreasing throughout the light phase until not detectable in early dark phase. When α-hydroxy-2-pyridinemethanesulfonate was used as a measure of glycolate biosynthesis, sufficient glycolate dehydrogenase was present to oxidize the glycolate produced at all stages over the cycle.
Full text
PDF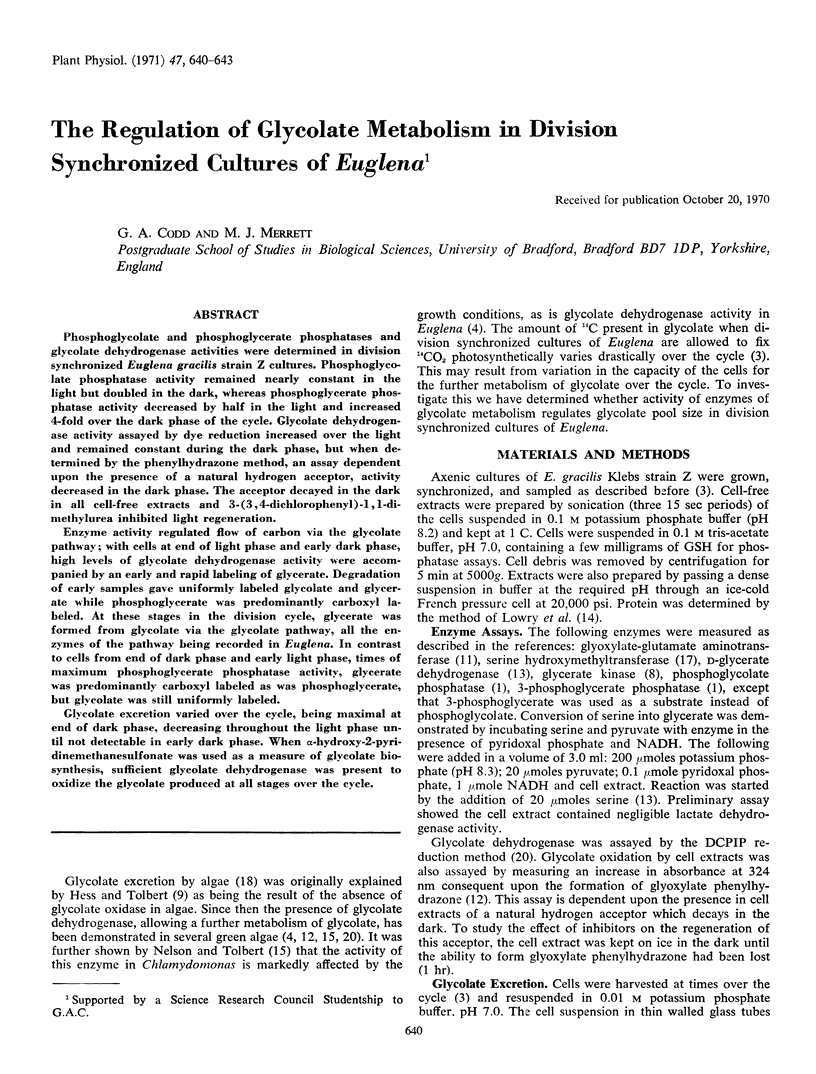
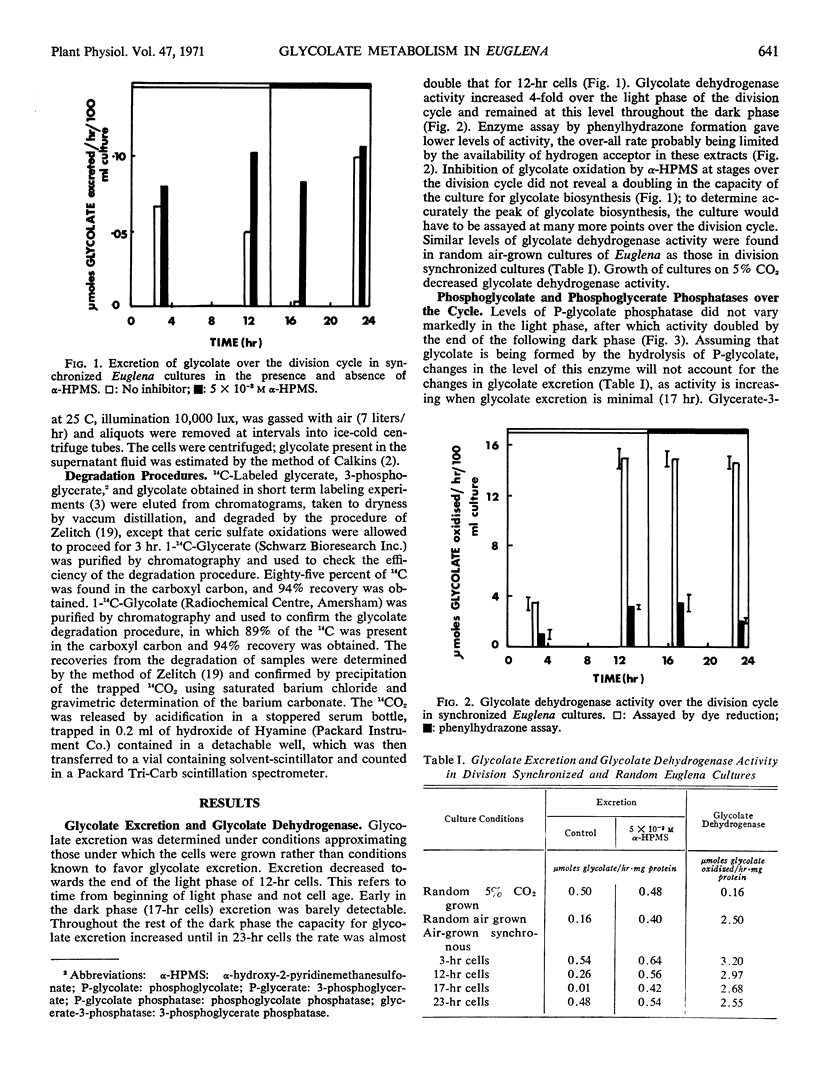
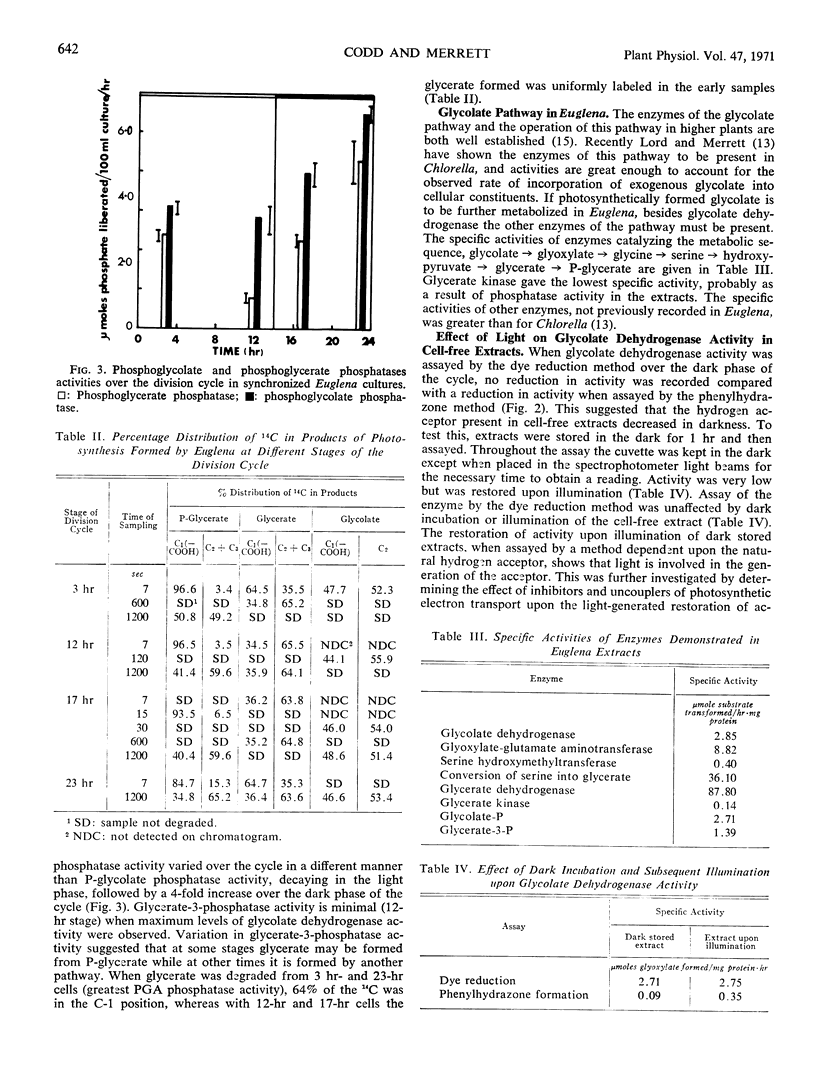
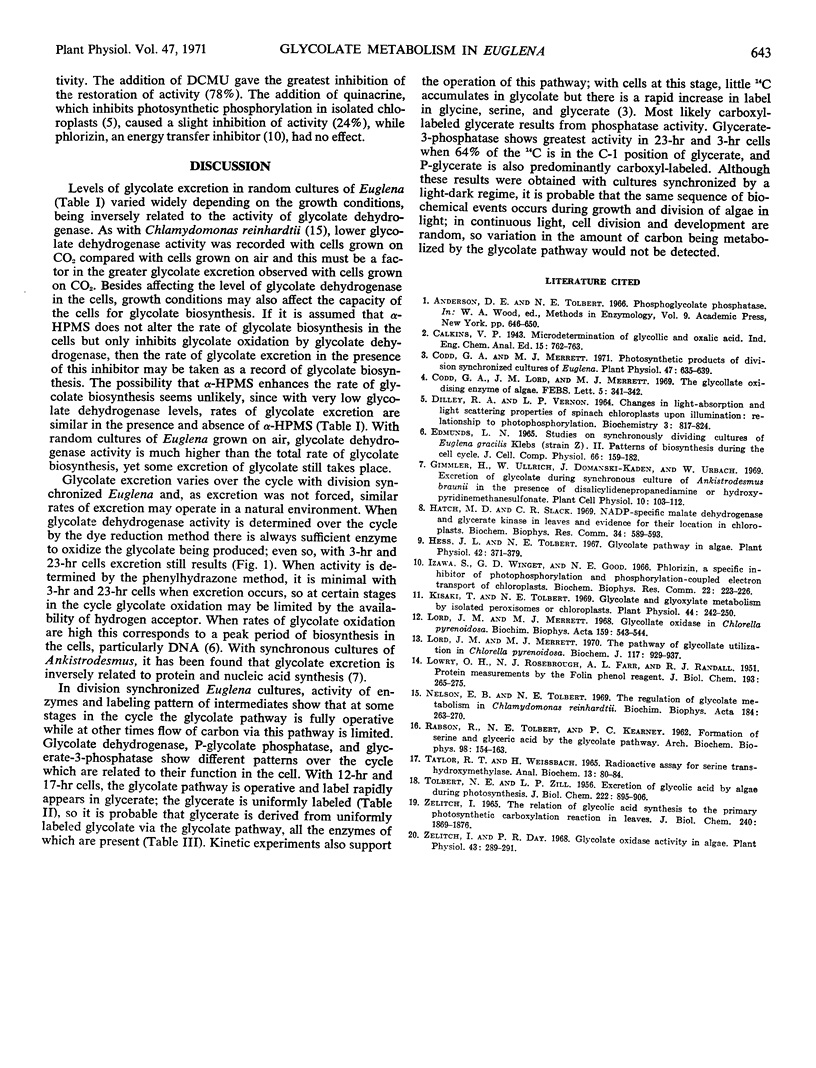
Selected References
These references are in PubMed. This may not be the complete list of references from this article.
- Codd G. A., Lord J. M., Merrett M. J. The glycollate oxidising enzyme of algae. FEBS Lett. 1969 Dec 30;5(5):341–342. doi: 10.1016/0014-5793(69)80352-2. [DOI] [PubMed] [Google Scholar]
- Codd G. A., Merrett M. J. Photosynthetic products of division synchronized cultures of euglena. Plant Physiol. 1971 May;47(5):635–639. doi: 10.1104/pp.47.5.635. [DOI] [PMC free article] [PubMed] [Google Scholar]
- DILLEY R. A., VERNON L. P. CHANGES IN LIGHT-ABSORPTION AND LIGHT-SCATTERING PROPERTIES OF SPINACH CHLOROPLASTS UPON ILLUMINATION: RELATIONSHIP TO PHOTOPHOSPHORYLATION. Biochemistry. 1964 Jun;3:817–824. doi: 10.1021/bi00894a016. [DOI] [PubMed] [Google Scholar]
- Hatch M. D., Slack C. R. NADP-specific malate dehydrogenase and glycerate kinase in leaves and evidence for their location in chloroplasts. Biochem Biophys Res Commun. 1969 Mar 10;34(5):589–593. doi: 10.1016/0006-291x(69)90778-5. [DOI] [PubMed] [Google Scholar]
- Hess J. L., Tolbert N. E. Glycolate pathway in algae. Plant Physiol. 1967 Mar;42(3):371–379. doi: 10.1104/pp.42.3.371. [DOI] [PMC free article] [PubMed] [Google Scholar]
- Izawa S., Winget G. D., Good N. E. Phlorizin, a specific inhibitor of photophosphorylation and phosphorylation-coupled electron transport in chloroplasts. Biochem Biophys Res Commun. 1966 Jan 24;22(2):223–226. doi: 10.1016/0006-291x(66)90436-0. [DOI] [PubMed] [Google Scholar]
- Kisaki T., Tolbert N. E. Glycolate and glyoxylate metabolism by isolated peroxisomes or chloroplasts. Plant Physiol. 1969 Feb;44(2):242–250. doi: 10.1104/pp.44.2.242. [DOI] [PMC free article] [PubMed] [Google Scholar]
- LOWRY O. H., ROSEBROUGH N. J., FARR A. L., RANDALL R. J. Protein measurement with the Folin phenol reagent. J Biol Chem. 1951 Nov;193(1):265–275. [PubMed] [Google Scholar]
- Lord J. M., Merrett M. J. The pathway of glycollate utilization in Chlorella pyrenoidosa. Biochem J. 1970 May;117(5):929–937. doi: 10.1042/bj1170929. [DOI] [PMC free article] [PubMed] [Google Scholar]
- Lord M. J., Merrett M. J. Glycollate oxidase in Chlorella pyrenoidosa. Biochim Biophys Acta. 1968 Jul 9;159(3):543–544. doi: 10.1016/0005-2744(68)90140-x. [DOI] [PubMed] [Google Scholar]
- Nelson E. B., Tolbert N. E. The regulation of glycolate metabolism in Chlamydomonas reinhardtii. Biochim Biophys Acta. 1969 Jul 30;184(2):263–270. doi: 10.1016/0304-4165(69)90028-2. [DOI] [PubMed] [Google Scholar]
- RABSON R., TOLBERTNE, KEARNEY P. C. Formation of serine and glyceric acid by the glycolate pathway. Arch Biochem Biophys. 1962 Jul;98:154–163. doi: 10.1016/0003-9861(62)90161-3. [DOI] [PubMed] [Google Scholar]
- TOLBERT N. E., ZILL L. P. Excretion of glycolic acid by algae during photosynthesis. J Biol Chem. 1956 Oct;222(2):895–906. [PubMed] [Google Scholar]
- ZELITCH I. THE RELATION OF GLYCOLIC ACID SYNTHESIS TO THE PRIMARY PHOTOSYNTHETIC CARBOXYLATION REACTION IN LEAVES. J Biol Chem. 1965 May;240:1869–1876. [PubMed] [Google Scholar]
- Zelitch I., Day P. R. Glycolate oxidase activity in algae. Plant Physiol. 1968 Feb;43(2):289–291. doi: 10.1104/pp.43.2.289. [DOI] [PMC free article] [PubMed] [Google Scholar]