Abstract
We have replaced the glutamic acid-165 at the active site of chicken triosephosphate isomerase with an aspartic acid residue using site-directed mutagenesis. Expression of the mutant protein in a strain of Escherichia coli that lacks the bacterial isomerase results in a complementation phenotype that is intermediate between strains that have no isomerase and strains that produce either the wild-type chicken enzyme or the native E. coli isomerase. The value of kcat for the purified mutant enzyme when glyceraldehyde 3-phosphate is the substrate is 1/1500th that of the wild-type enzyme, and the Km is decreased by a factor of 3.6. With dihydroxyacetone phosphate as substrate, the kcat value is 1/240th that of the wild-type enzyme, and Km is 2 times higher. The value of Ki for a competitive inhibitor, phosphoglycolate, is the same for the mutant and wild-type enzymes, at 2 X 10(-5) M. By treating the enzyme-catalyzed isomerization as a simple three step process and assuming that substrate binding is diffusion limited, it is evident that the mutation of glutamic acid-165 to aspartic acid principally affects the free energy of the transition state(s) for the catalytic reaction itself.
Full text
PDF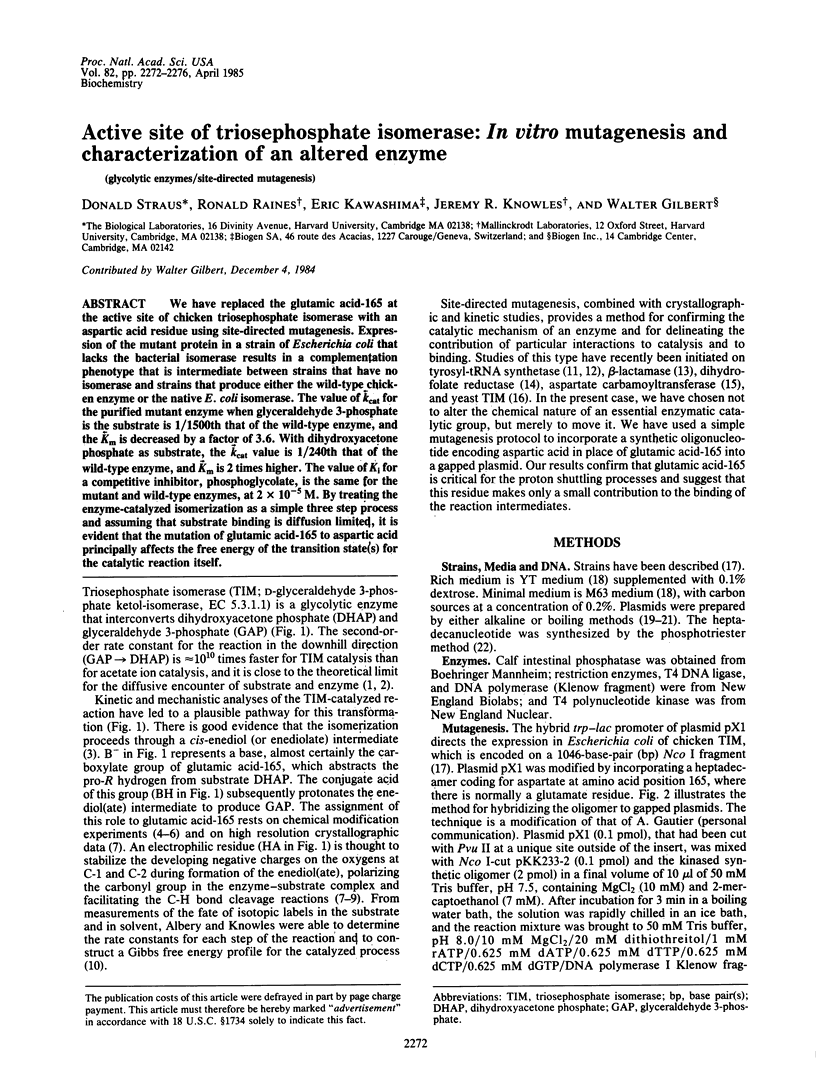
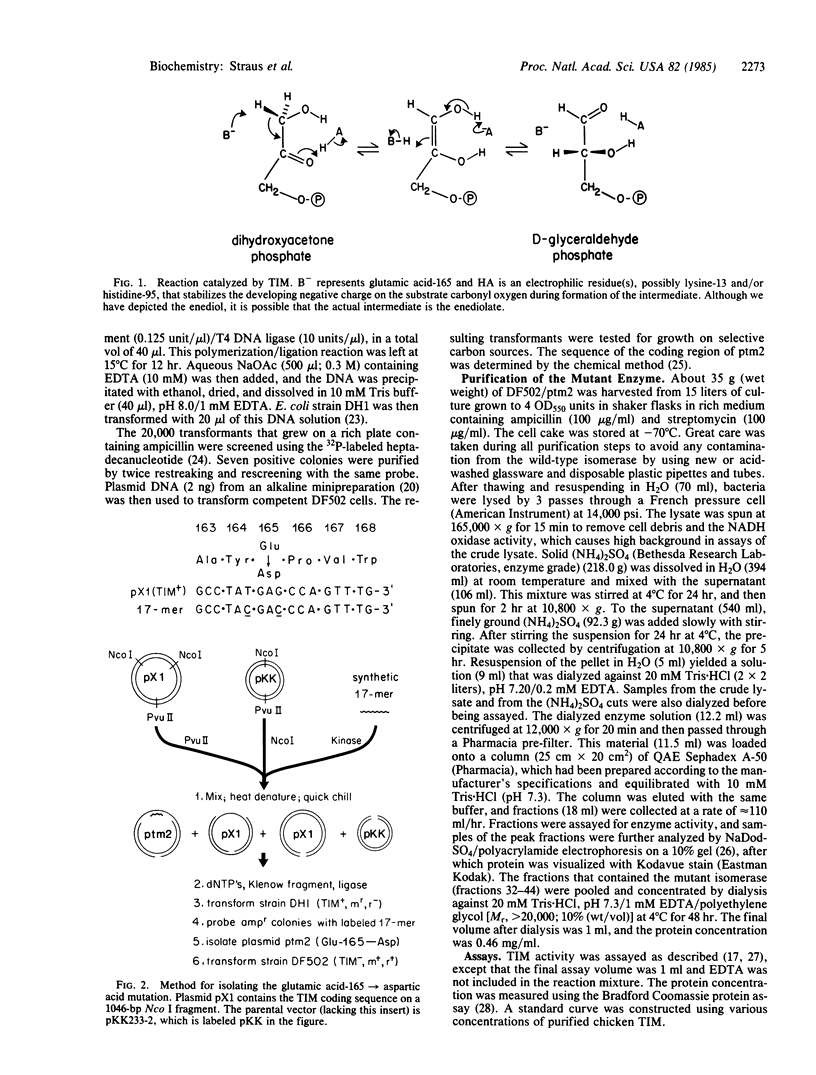
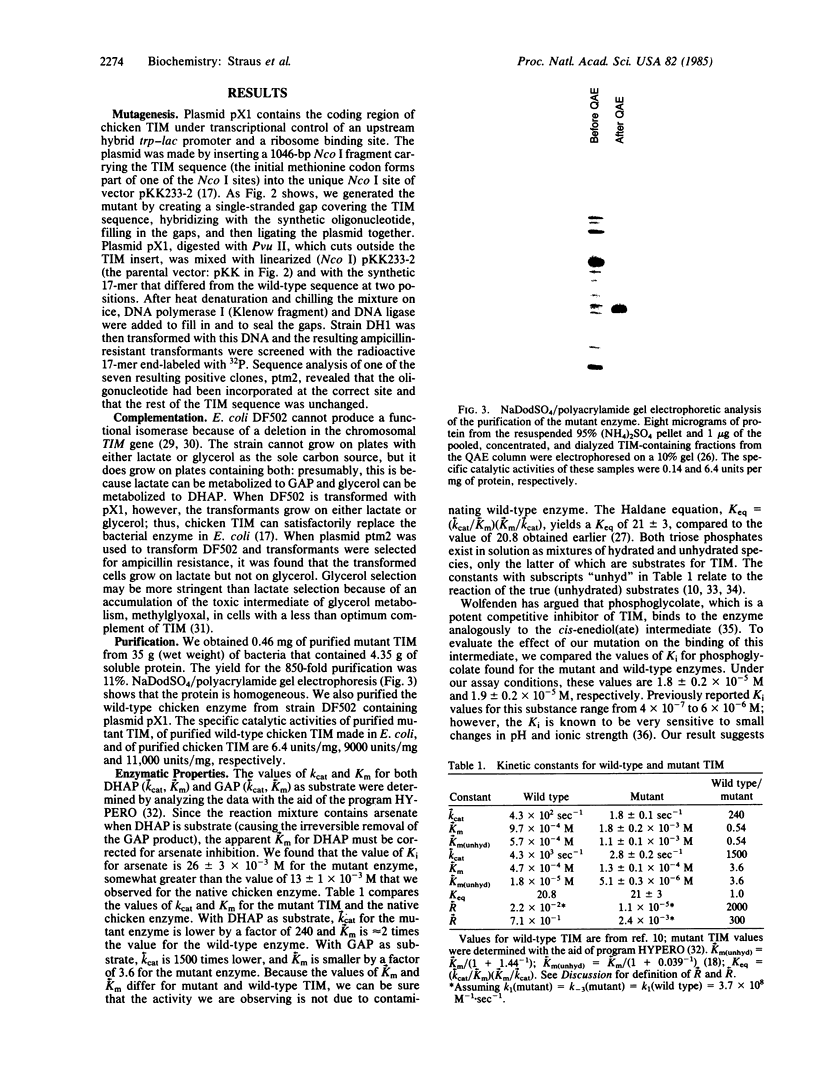
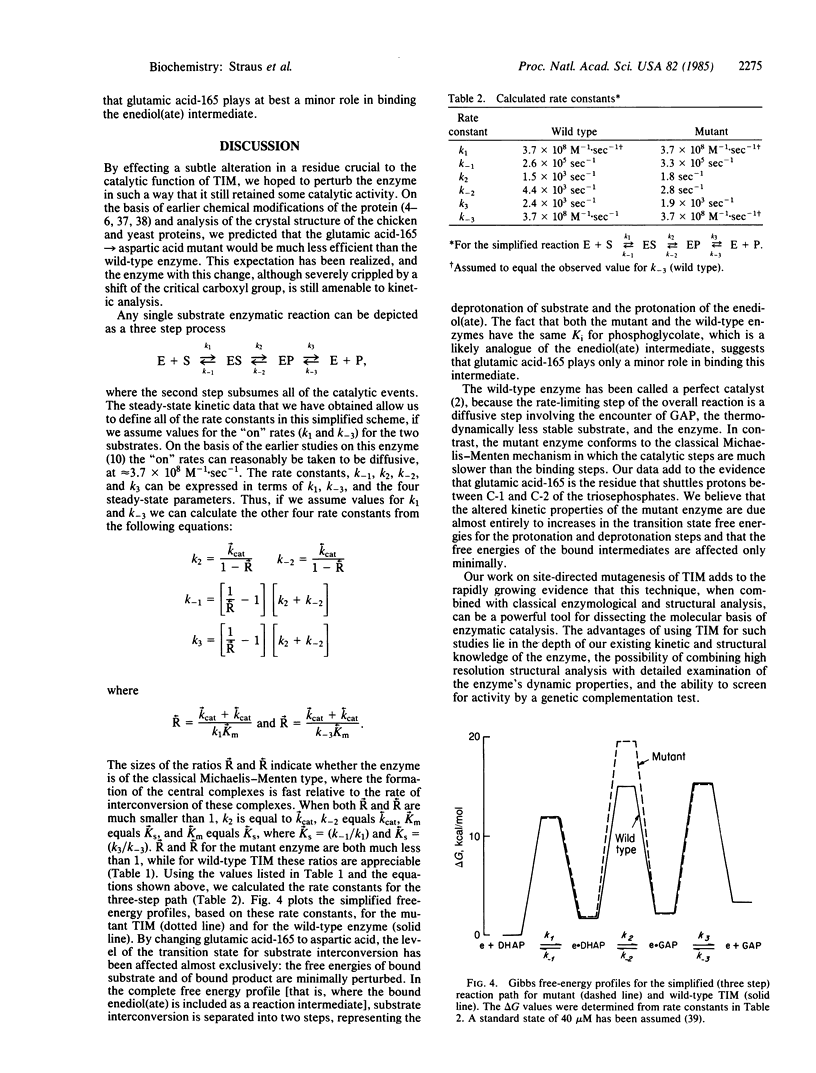
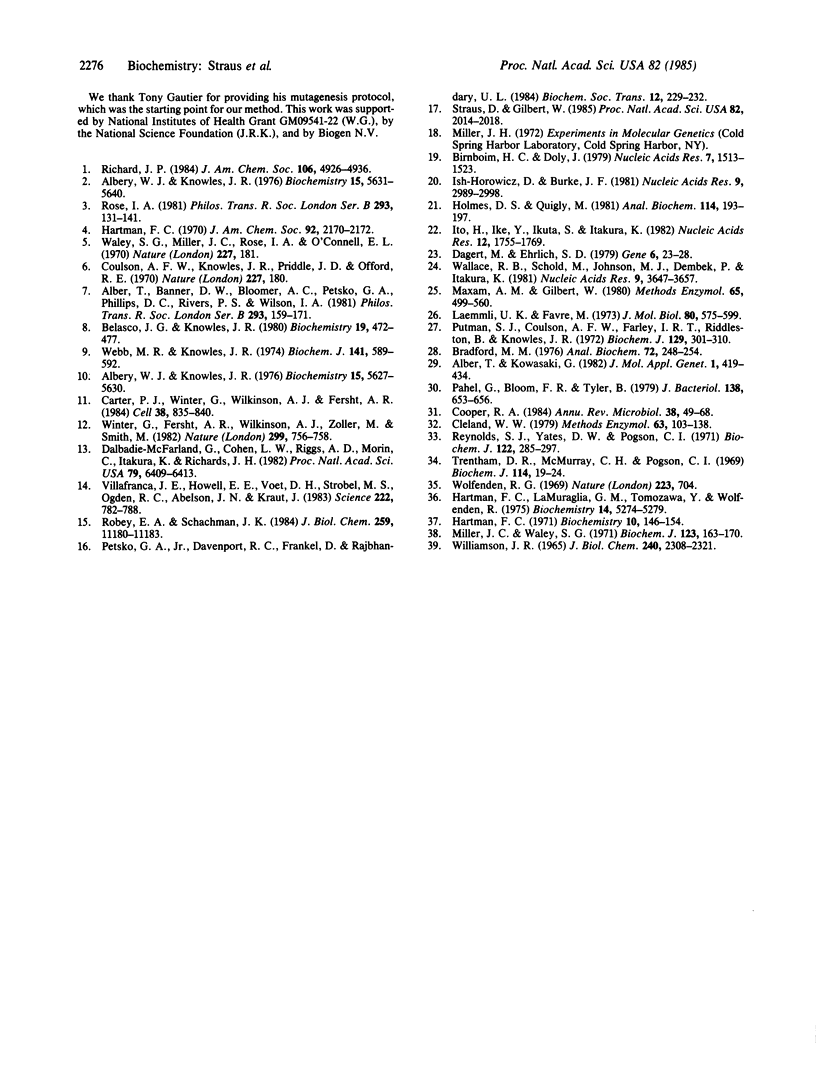
Images in this article
Selected References
These references are in PubMed. This may not be the complete list of references from this article.
- Alber T., Banner D. W., Bloomer A. C., Petsko G. A., Phillips D., Rivers P. S., Wilson I. A. On the three-dimensional structure and catalytic mechanism of triose phosphate isomerase. Philos Trans R Soc Lond B Biol Sci. 1981 Jun 26;293(1063):159–171. doi: 10.1098/rstb.1981.0069. [DOI] [PubMed] [Google Scholar]
- Alber T., Kawasaki G. Nucleotide sequence of the triose phosphate isomerase gene of Saccharomyces cerevisiae. J Mol Appl Genet. 1982;1(5):419–434. [PubMed] [Google Scholar]
- Albery W. J., Knowles J. R. Evolution of enzyme function and the development of catalytic efficiency. Biochemistry. 1976 Dec 14;15(25):5631–5640. doi: 10.1021/bi00670a032. [DOI] [PubMed] [Google Scholar]
- Albery W. J., Knowles J. R. Free-energy profile of the reaction catalyzed by triosephosphate isomerase. Biochemistry. 1976 Dec 14;15(25):5627–5631. doi: 10.1021/bi00670a031. [DOI] [PubMed] [Google Scholar]
- Belasco J. G., Knowles J. R. Direct observation of substrate distortion by triosephosphate isomerase using Fourier transform infrared spectroscopy. Biochemistry. 1980 Feb 5;19(3):472–477. doi: 10.1021/bi00544a012. [DOI] [PubMed] [Google Scholar]
- Birnboim H. C., Doly J. A rapid alkaline extraction procedure for screening recombinant plasmid DNA. Nucleic Acids Res. 1979 Nov 24;7(6):1513–1523. doi: 10.1093/nar/7.6.1513. [DOI] [PMC free article] [PubMed] [Google Scholar]
- Bradford M. M. A rapid and sensitive method for the quantitation of microgram quantities of protein utilizing the principle of protein-dye binding. Anal Biochem. 1976 May 7;72:248–254. doi: 10.1006/abio.1976.9999. [DOI] [PubMed] [Google Scholar]
- Carter P. J., Winter G., Wilkinson A. J., Fersht A. R. The use of double mutants to detect structural changes in the active site of the tyrosyl-tRNA synthetase (Bacillus stearothermophilus). Cell. 1984 Oct;38(3):835–840. doi: 10.1016/0092-8674(84)90278-2. [DOI] [PubMed] [Google Scholar]
- Cleland W. W. Statistical analysis of enzyme kinetic data. Methods Enzymol. 1979;63:103–138. doi: 10.1016/0076-6879(79)63008-2. [DOI] [PubMed] [Google Scholar]
- Cooper R. A. Metabolism of methylglyoxal in microorganisms. Annu Rev Microbiol. 1984;38:49–68. doi: 10.1146/annurev.mi.38.100184.000405. [DOI] [PubMed] [Google Scholar]
- Coulson A. F., Knowles J. R., Priddle J. D., Offord R. E. Uniquely labelled active site sequence in chicken muscle triose phosphate isomerase. Nature. 1970 Jul 11;227(5254):180–181. doi: 10.1038/227180a0. [DOI] [PubMed] [Google Scholar]
- Dagert M., Ehrlich S. D. Prolonged incubation in calcium chloride improves the competence of Escherichia coli cells. Gene. 1979 May;6(1):23–28. doi: 10.1016/0378-1119(79)90082-9. [DOI] [PubMed] [Google Scholar]
- Dalbadie-McFarland G., Cohen L. W., Riggs A. D., Morin C., Itakura K., Richards J. H. Oligonucleotide-directed mutagenesis as a general and powerful method for studies of protein function. Proc Natl Acad Sci U S A. 1982 Nov;79(21):6409–6413. doi: 10.1073/pnas.79.21.6409. [DOI] [PMC free article] [PubMed] [Google Scholar]
- Hartman F. C. Haloacetol phosphates. Characterization of the active site of rabbit muscle triose phosphate isomerase. Biochemistry. 1971 Jan 5;10(1):146–154. doi: 10.1021/bi00777a021. [DOI] [PubMed] [Google Scholar]
- Hartman F. C. Isolation and characterization of an active-site peptide from triose phosphate isomerase. J Am Chem Soc. 1970 Apr 8;92(7):2170–2172. doi: 10.1021/ja00710a082. [DOI] [PubMed] [Google Scholar]
- Hartman F. C., LaMuraglia G. M., Tomozawa Y., Wolfenden R. The influence of pH on the interaction of inhibitors with triosephosphate isomerase and determination of the pKa of the active-site carboxyl group. Biochemistry. 1975 Dec 2;14(24):5274–5279. doi: 10.1021/bi00695a007. [DOI] [PubMed] [Google Scholar]
- Holmes D. S., Quigley M. A rapid boiling method for the preparation of bacterial plasmids. Anal Biochem. 1981 Jun;114(1):193–197. doi: 10.1016/0003-2697(81)90473-5. [DOI] [PubMed] [Google Scholar]
- Ish-Horowicz D., Burke J. F. Rapid and efficient cosmid cloning. Nucleic Acids Res. 1981 Jul 10;9(13):2989–2998. doi: 10.1093/nar/9.13.2989. [DOI] [PMC free article] [PubMed] [Google Scholar]
- Ito H., Ike Y., Ikuta S., Itakura K. Solid phase synthesis of polynucleotides. VI. Further studies on polystyrene copolymers for the solid support. Nucleic Acids Res. 1982 Mar 11;10(5):1755–1769. doi: 10.1093/nar/10.5.1755. [DOI] [PMC free article] [PubMed] [Google Scholar]
- Laemmli U. K., Favre M. Maturation of the head of bacteriophage T4. I. DNA packaging events. J Mol Biol. 1973 Nov 15;80(4):575–599. doi: 10.1016/0022-2836(73)90198-8. [DOI] [PubMed] [Google Scholar]
- Maxam A. M., Gilbert W. Sequencing end-labeled DNA with base-specific chemical cleavages. Methods Enzymol. 1980;65(1):499–560. doi: 10.1016/s0076-6879(80)65059-9. [DOI] [PubMed] [Google Scholar]
- Miller J. C., Waley S. G. The active centre of rabbit muscle triose phosphate isomerase. The site that is labelled by glycidol phosphate. Biochem J. 1971 Jun;123(2):163–170. doi: 10.1042/bj1230163. [DOI] [PMC free article] [PubMed] [Google Scholar]
- Pahel G., Bloom F. R., Tyler B. Deletion mapping of the polA-metB region of the Escherichia coli chromosome. J Bacteriol. 1979 May;138(2):653–656. doi: 10.1128/jb.138.2.653-656.1979. [DOI] [PMC free article] [PubMed] [Google Scholar]
- Petsko G. A., Davenport R. C., Jr, Frankel D., RaiBhandary U. L. Probing the catalytic mechanism of yeast triose phosphate isomerase by site-specific mutagenesis. Biochem Soc Trans. 1984 Apr;12(2):229–232. doi: 10.1042/bst0120229. [DOI] [PubMed] [Google Scholar]
- Putman S. J., Coulson A. F., Farley I. R., Riddleston B., Knowles J. R. Specificity and kinetics of triose phosphate isomerase from chicken muscle. Biochem J. 1972 Sep;129(2):301–310. doi: 10.1042/bj1290301. [DOI] [PMC free article] [PubMed] [Google Scholar]
- Reynolds S. J., Yates D. W., Pogson C. I. Dihydroxyacetone phosphate. Its structure and reactivity with -glycerophosphate dehydrogenase, aldolase and triose phosphate isomerase and some possible metabolic implications. Biochem J. 1971 Apr;122(3):285–297. doi: 10.1042/bj1220285. [DOI] [PMC free article] [PubMed] [Google Scholar]
- Robey E. A., Schachman H. K. Site-specific mutagenesis of aspartate transcarbamoylase. Replacement of tyrosine 165 in the catalytic chain by serine reduces enzymatic activity. J Biol Chem. 1984 Sep 25;259(18):11180–11183. [PubMed] [Google Scholar]
- Straus D., Gilbert W. Chicken triosephosphate isomerase complements an Escherichia coli deficiency. Proc Natl Acad Sci U S A. 1985 Apr;82(7):2014–2018. doi: 10.1073/pnas.82.7.2014. [DOI] [PMC free article] [PubMed] [Google Scholar]
- Trentham D. R., McMurray C. H., Pogson C. I. The active chemical state of D-glyceraldehyde 3-phosphate in its reactions with D-glyceraldehyde 3-phosphate dehydrogenase, aldolase and triose phosphate isomerase. Biochem J. 1969 Aug;114(1):19–24. doi: 10.1042/bj1140019. [DOI] [PMC free article] [PubMed] [Google Scholar]
- Villafranca J. E., Howell E. E., Voet D. H., Strobel M. S., Ogden R. C., Abelson J. N., Kraut J. Directed mutagenesis of dihydrofolate reductase. Science. 1983 Nov 18;222(4625):782–788. doi: 10.1126/science.6356360. [DOI] [PubMed] [Google Scholar]
- WILLIAMSON J. R. GLYCOLYTIC CONTROL MECHANISMS. I. INHIBITION OF GLYCOLYSIS BY ACETATE AND PYRUVATE IN THE ISOLATED, PERFUSED RAT HEART. J Biol Chem. 1965 Jun;240:2308–2321. [PubMed] [Google Scholar]
- Waley S. G., Miller J. C., Rose I. A., O'Connell E. L. Identification of site in triose phosphate isomerase labelled by glycidol phosphate. Nature. 1970 Jul 11;227(5254):181–181. doi: 10.1038/227181a0. [DOI] [PubMed] [Google Scholar]
- Wallace R. B., Schold M., Johnson M. J., Dembek P., Itakura K. Oligonucleotide directed mutagenesis of the human beta-globin gene: a general method for producing specific point mutations in cloned DNA. Nucleic Acids Res. 1981 Aug 11;9(15):3647–3656. doi: 10.1093/nar/9.15.3647. [DOI] [PMC free article] [PubMed] [Google Scholar]
- Webb M. R., Knowles J. R. The existence of an electrophilic component in the reaction catalysed by triose phosphate isomerase. Biochem J. 1974 Aug;141(2):589–592. doi: 10.1042/bj1410589. [DOI] [PMC free article] [PubMed] [Google Scholar]
- Winter G., Fersht A. R., Wilkinson A. J., Zoller M., Smith M. Redesigning enzyme structure by site-directed mutagenesis: tyrosyl tRNA synthetase and ATP binding. Nature. 1982 Oct 21;299(5885):756–758. doi: 10.1038/299756a0. [DOI] [PubMed] [Google Scholar]
- Wolfenden R. Transition state analogues for enzyme catalysis. Nature. 1969 Aug 16;223(5207):704–705. doi: 10.1038/223704a0. [DOI] [PubMed] [Google Scholar]