Abstract
We propose a role for GTP hydrolysis in microtubule assembly in which the GTPase reaction serves to stabilize tubulin subunits in the microtubule. The GTPase reaction in tubulin subunits containing GTP at microtubule ends is presumed to occur predominately in subunits at one of the interfaces between a cap of GTP-containing tubulin subunit and a core of GDP-containing tubulin subunit in the microtubule, resulting in elongation of the core. The proposed model interprets the effects of GDP on microtubule assembly, using a reaction scheme in which GDP-containing tubulin subunits are able to add to microtubule ends. The model can account for the GTP requirement for microtubule assembly, the GDP inhibition of the rate for microtubule elongation, and the fact that a metastable state exists after the enzymic conversion of GTP to GDP, with microtubules which are at steady state. To account for the fact that the microtubule assembly and disassembly rates are nonlinearly dependent upon the tubulin subunit concentration and for the effects of GDP-containing tubulin subunits on the kinetic properties of microtubules, our scheme includes nonproductive as well as productive binding of GTP- and GDP-containing tubulin subunits. We compare our model with an alternative scheme [Hill, T. L. & Carlier, M. F. (1983) Proc. Natl. Acad. Sci. USA 80, 7234-7238], which interprets the effects of GDP on microtubule assembly using a reaction scheme in which GDP is able to exchange with GTP in GTP-containing tubulin subunits in the microtubule and in which the principal GTPase occurs in GTP-containing tubulin subunits at the microtubule/solution interface.
Full text
PDF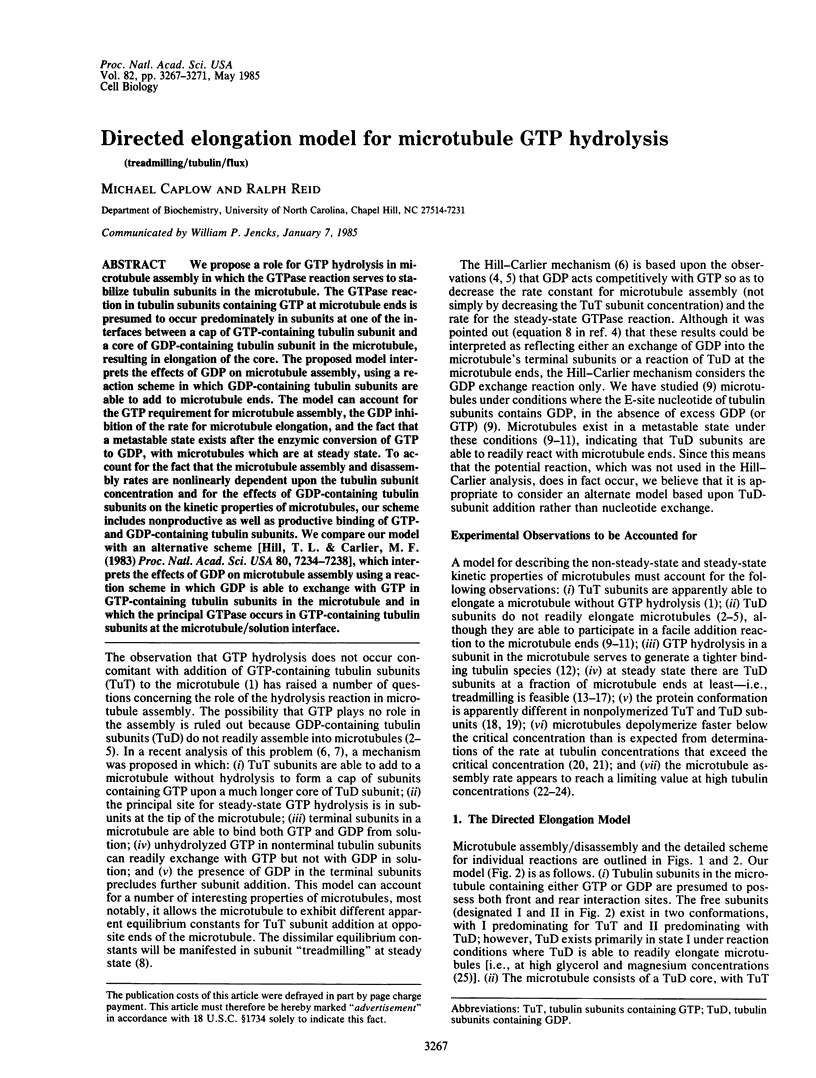
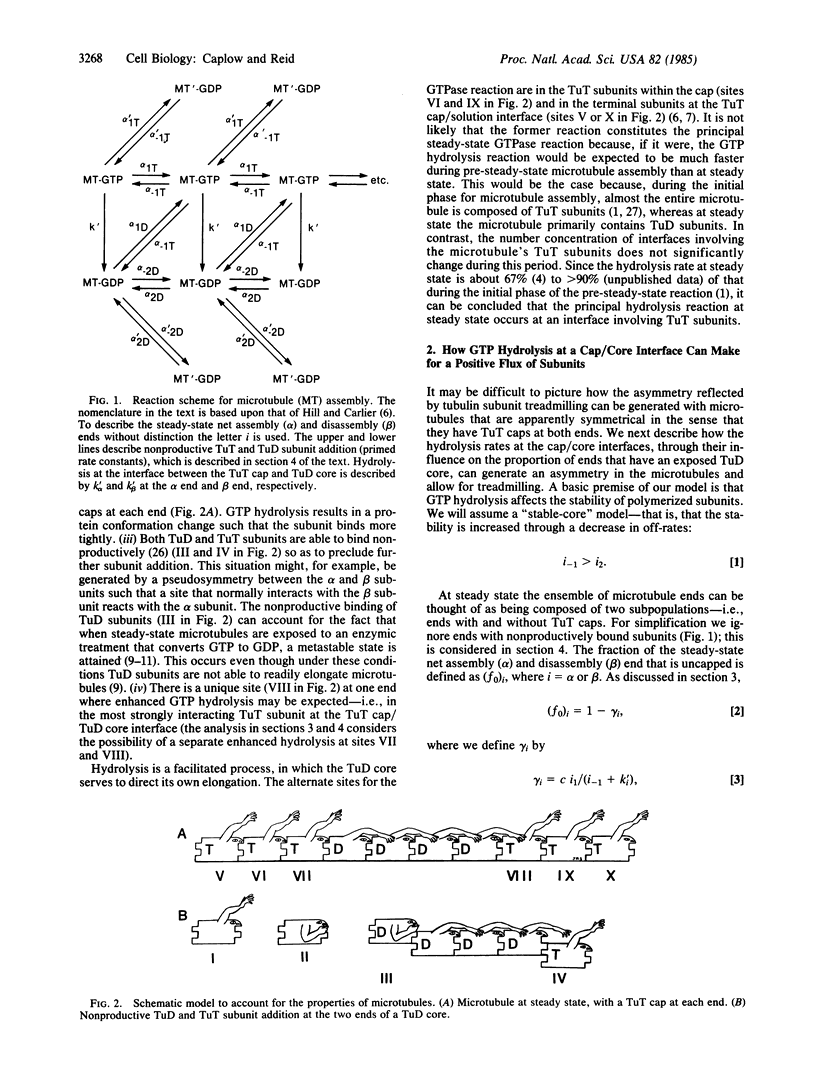
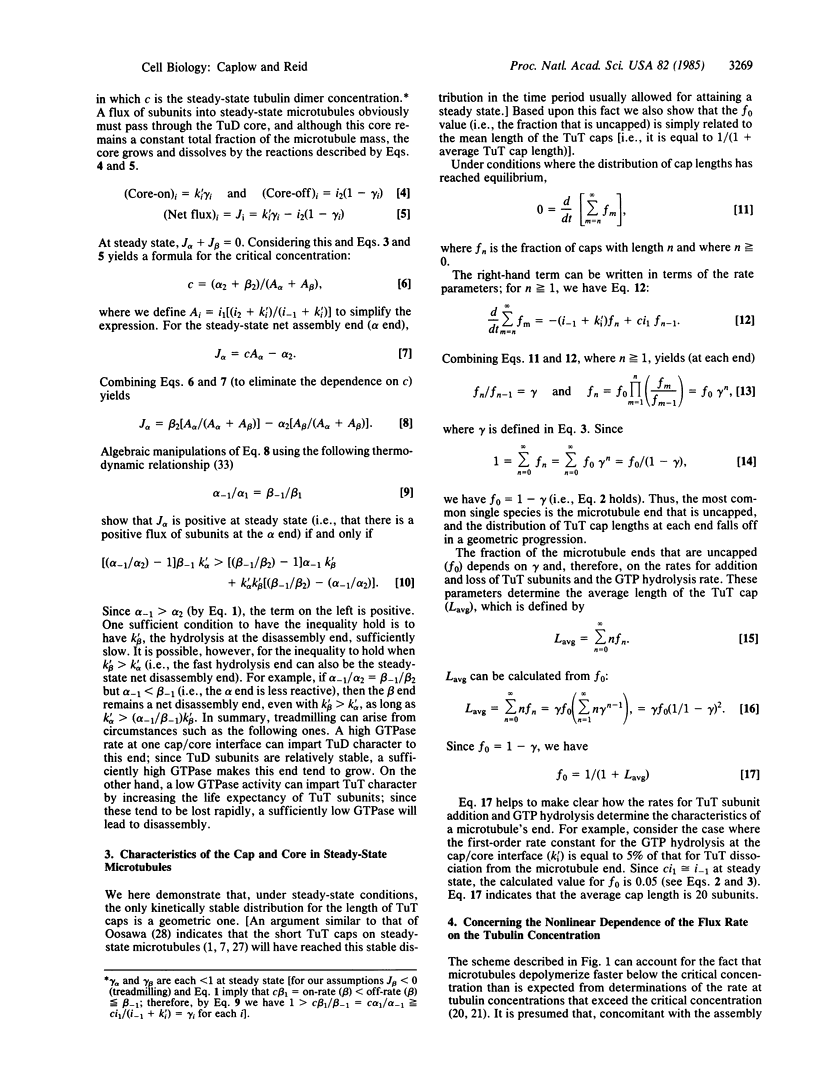
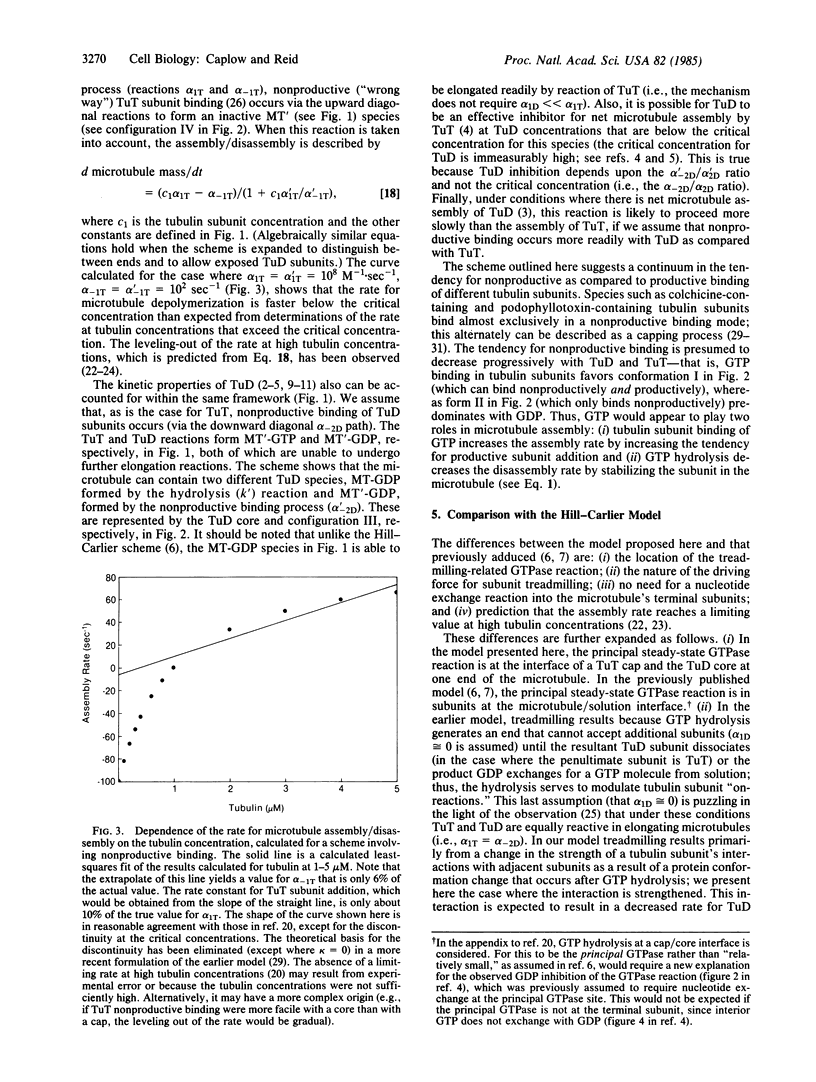
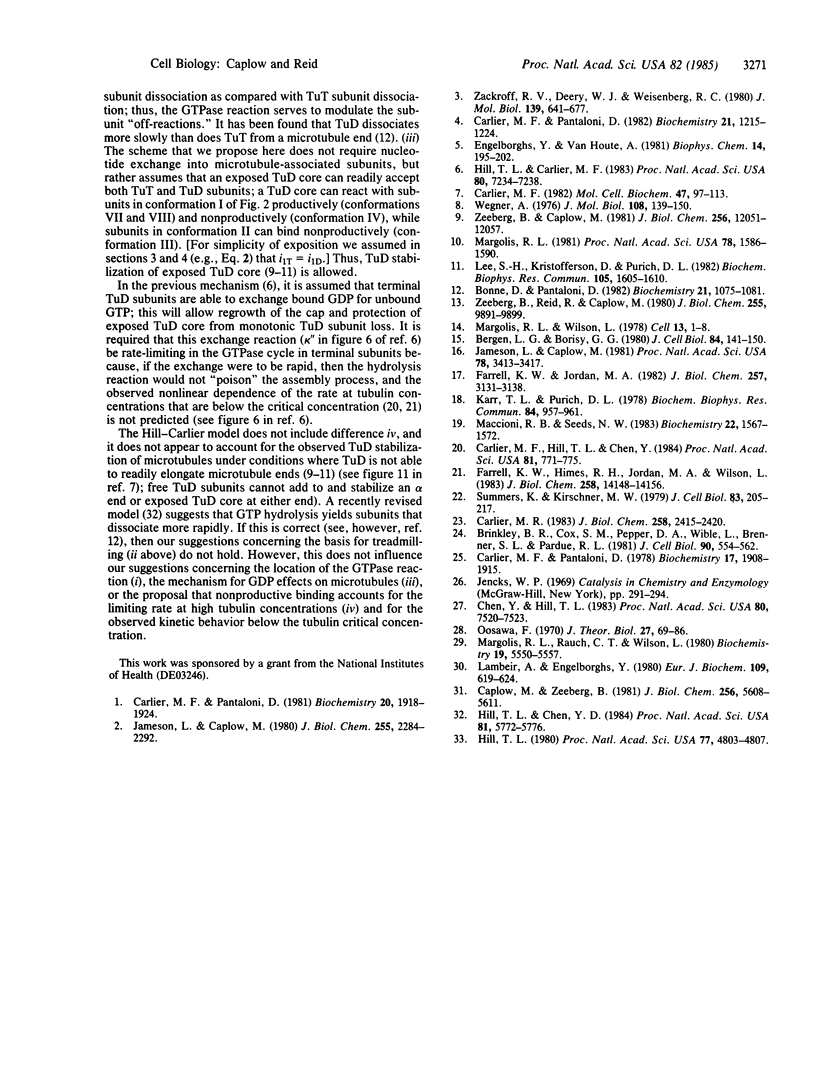
Selected References
These references are in PubMed. This may not be the complete list of references from this article.
- Bergen L. G., Borisy G. G. Head-to-tail polymerization of microtubules in vitro. Electron microscope analysis of seeded assembly. J Cell Biol. 1980 Jan;84(1):141–150. doi: 10.1083/jcb.84.1.141. [DOI] [PMC free article] [PubMed] [Google Scholar]
- Bonne D., Pantaloni D. Mechanism of tubulin assembly: guanosine 5'-triphosphate hydrolysis decreases the rate of microtubule depolymerization. Biochemistry. 1982 Mar 2;21(5):1075–1081. doi: 10.1021/bi00534a039. [DOI] [PubMed] [Google Scholar]
- Brinkley B. R., Cox S. M., Pepper D. A., Wible L., Brenner S. L., Pardue R. L. Tubulin assembly sites and the organization of cytoplasmic microtubules in cultured mammalian cells. J Cell Biol. 1981 Sep;90(3):554–562. doi: 10.1083/jcb.90.3.554. [DOI] [PMC free article] [PubMed] [Google Scholar]
- Caplow M., Zeeberg B. Incorporation of radioactive tubulin into microtubules at steady state. Experimental and theoretical analysis of the effect of podophyllotoxin. J Biol Chem. 1981 Jun 10;256(11):5608–5611. [PubMed] [Google Scholar]
- Carlier M. F. Guanosine-5'-triphosphate hydrolysis and tubulin polymerization. Review article. Mol Cell Biochem. 1982 Sep 3;47(2):97–113. doi: 10.1007/BF00234410. [DOI] [PubMed] [Google Scholar]
- Carlier M. F., Hill T. L., Chen Y. Interference of GTP hydrolysis in the mechanism of microtubule assembly: an experimental study. Proc Natl Acad Sci U S A. 1984 Feb;81(3):771–775. doi: 10.1073/pnas.81.3.771. [DOI] [PMC free article] [PubMed] [Google Scholar]
- Carlier M. F. Kinetic evidence for a conformation change of tubulin preceding microtubule assembly. J Biol Chem. 1983 Feb 25;258(4):2415–2420. [PubMed] [Google Scholar]
- Carlier M. F., Pantaloni D. Assembly of microtubule protein: role of guanosine di- and triphosphate nucleotides. Biochemistry. 1982 Mar 16;21(6):1215–1224. doi: 10.1021/bi00535a017. [DOI] [PubMed] [Google Scholar]
- Carlier M. F., Pantaloni D. Kinetic analysis of cooperativity in tubulin polymerization in the presence of guanosine di- or triphosphate nucleotides. Biochemistry. 1978 May 16;17(10):1908–1915. doi: 10.1021/bi00603a017. [DOI] [PubMed] [Google Scholar]
- Carlier M. F., Pantaloni D. Kinetic analysis of guanosine 5'-triphosphate hydrolysis associated with tubulin polymerization. Biochemistry. 1981 Mar 31;20(7):1918–1924. doi: 10.1021/bi00510a030. [DOI] [PubMed] [Google Scholar]
- Chen Y., Hill T. L. Use of Monte Carlo calculations in the study of microtubule subunit kinetics. Proc Natl Acad Sci U S A. 1983 Dec;80(24):7520–7523. doi: 10.1073/pnas.80.24.7520. [DOI] [PMC free article] [PubMed] [Google Scholar]
- Engelborghs Y., Van Houtte A. Temperature jump relaxation study of microtubule elongation in the presence of GTP/GDP mixtures. Biophys Chem. 1981 Oct;14(2):195–202. doi: 10.1016/0301-4622(81)85019-3. [DOI] [PubMed] [Google Scholar]
- Farrell K. W., Himes R. H., Jordan M. A., Wilson L. On the nonlinear relationship between the initial rates of dilution-induced microtubule disassembly and the initial free subunit concentration. J Biol Chem. 1983 Dec 10;258(23):14148–14156. [PubMed] [Google Scholar]
- Farrell K. W., Jordan M. A. A kinetic analysis of assembly-disassembly at opposite microtubule ends. J Biol Chem. 1982 Mar 25;257(6):3131–3138. [PubMed] [Google Scholar]
- Hill T. L. Bioenergetic aspects and polymer length distribution in steady-state head-to-tail polymerization of actin or microtubules. Proc Natl Acad Sci U S A. 1980 Aug;77(8):4803–4807. doi: 10.1073/pnas.77.8.4803. [DOI] [PMC free article] [PubMed] [Google Scholar]
- Hill T. L., Carlier M. F. Steady-state theory of the interference of GTP hydrolysis in the mechanism of microtubule assembly. Proc Natl Acad Sci U S A. 1983 Dec;80(23):7234–7238. doi: 10.1073/pnas.80.23.7234. [DOI] [PMC free article] [PubMed] [Google Scholar]
- Hill T. L., Chen Y. Phase changes at the end of a microtubule with a GTP cap. Proc Natl Acad Sci U S A. 1984 Sep;81(18):5772–5776. doi: 10.1073/pnas.81.18.5772. [DOI] [PMC free article] [PubMed] [Google Scholar]
- Jameson L., Caplow M. Effect of guanosine diphosphate on microtubule assembly and stability. J Biol Chem. 1980 Mar 25;255(6):2284–2292. [PubMed] [Google Scholar]
- Jameson L., Caplow M. Modification of microtubule steady-state dynamics by phosphorylation of the microtubule-associated proteins. Proc Natl Acad Sci U S A. 1981 Jun;78(6):3413–3417. doi: 10.1073/pnas.78.6.3413. [DOI] [PMC free article] [PubMed] [Google Scholar]
- Karr T. L., Purich D. L. Examination of tubulin-nucleotide interactions by protein fluorescence quenching measurements. Biochem Biophys Res Commun. 1978 Oct 30;84(4):957–961. doi: 10.1016/0006-291x(78)91676-5. [DOI] [PubMed] [Google Scholar]
- Lambier A., Engelborghs Y. A quantitative analysis of tubulin-colchicine binding to microtubules. Eur J Biochem. 1980 Aug;109(2):619–624. doi: 10.1111/j.1432-1033.1980.tb04835.x. [DOI] [PubMed] [Google Scholar]
- Lee S. H., Kristofferson D., Purich D. L. Microtubule interactions with GDP provide evidence that assembly-disassembly properties depend on the method of brain microtubule protein isolation. Biochem Biophys Res Commun. 1982 Apr 29;105(4):1605–1610. doi: 10.1016/0006-291x(82)90972-x. [DOI] [PubMed] [Google Scholar]
- Maccioni R. B., Seeds N. W. Limited proteolysis of tubulin: nucleotide stabilizes an active conformation. Biochemistry. 1983 Mar 29;22(7):1567–1572. doi: 10.1021/bi00276a007. [DOI] [PubMed] [Google Scholar]
- Margolis R. L., Rauch C. T., Wilson L. Mechanism of colchicine-dimer addition to microtubule ends: implications for the microtubule polymerization mechanism. Biochemistry. 1980 Nov 25;19(24):5550–5557. doi: 10.1021/bi00565a014. [DOI] [PubMed] [Google Scholar]
- Margolis R. L. Role of GTP hydrolysis in microtubule treadmilling and assembly. Proc Natl Acad Sci U S A. 1981 Mar;78(3):1586–1590. doi: 10.1073/pnas.78.3.1586. [DOI] [PMC free article] [PubMed] [Google Scholar]
- Margolis R. L., Wilson L. Opposite end assembly and disassembly of microtubules at steady state in vitro. Cell. 1978 Jan;13(1):1–8. doi: 10.1016/0092-8674(78)90132-0. [DOI] [PubMed] [Google Scholar]
- Oosawa F. Size distribution of protein polymers. J Theor Biol. 1970 Apr;27(1):69–86. doi: 10.1016/0022-5193(70)90129-3. [DOI] [PubMed] [Google Scholar]
- Summers K., Kirschner M. W. Characteristics of the polar assembly and disassembly of microtubules observed in vitro by darkfield light microscopy. J Cell Biol. 1979 Oct;83(1):205–217. doi: 10.1083/jcb.83.1.205. [DOI] [PMC free article] [PubMed] [Google Scholar]
- Wegner A. Head to tail polymerization of actin. J Mol Biol. 1976 Nov;108(1):139–150. doi: 10.1016/s0022-2836(76)80100-3. [DOI] [PubMed] [Google Scholar]
- Zackroff R. V., Weisenberg R. C., Deery W. J. Equilibrium and kinetic analysis of microtubule assembly in the presence of guanosine diphosphate. J Mol Biol. 1980 Jun 5;139(4):641–659. doi: 10.1016/0022-2836(80)90053-4. [DOI] [PubMed] [Google Scholar]
- Zeeberg B., Caplow M. An isoenergetic exchange mechanism which accounts for tubulin-GDP stabilization of microtubules. J Biol Chem. 1981 Dec 10;256(23):12051–12057. [PubMed] [Google Scholar]
- Zeeberg B., Reid R., Caplow M. Incorporation of radioactive tubulin into microtubules at steady state. Experimental and theoretical analyses of diffusional and directional flux. J Biol Chem. 1980 Oct 25;255(20):9891–9899. [PubMed] [Google Scholar]