Abstract
Physiological recordings and computer simulations of unitary inhibitory postsynaptic potentials in the Mauthner cell of the goldfish central nervous system have been used to estimate the expected size of the postsynaptic receptor matrix at individual junctions. Simultaneous pre- and postsynaptic recordings were used to determine the kinetic parameters of the quantal responses under normal conditions and in the presence of strychnine, a competitive antagonist of glycine, which is the putative transmitter at these synapses. Calculations indicate that if the postsynaptic density, which has a radius of 0.1 micron, were to accommodate the population of channels estimated to be opened during a quantal response, the glycine binding site density in that region would be unrealistically high. Computer simulation of the quantal responses included transmitter diffusion, transmitter-receptor interactions, and channel activation under conditions including both normal and lowered binding site densities, the latter corresponding to the experimental data obtained with strychnine. The data indicate that the synaptic receptors involved in generating unitary responses are widely distributed to include regions located outside the junctional area, which directly faces the presynaptic release sites. We further suggest that the receptor matrix is surrounded by a restricted diffusional space; this geometrical organization may underlie the finding that response rise times are relatively independent of receptor binding site densities.
Full text
PDF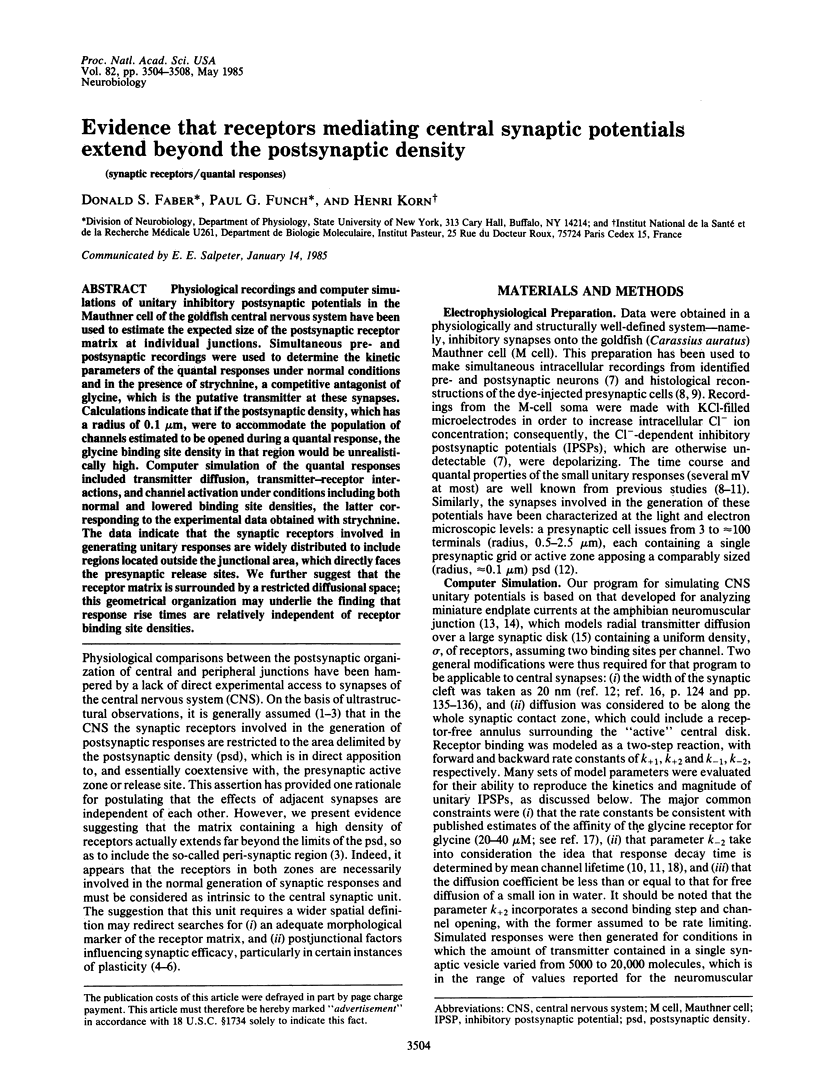
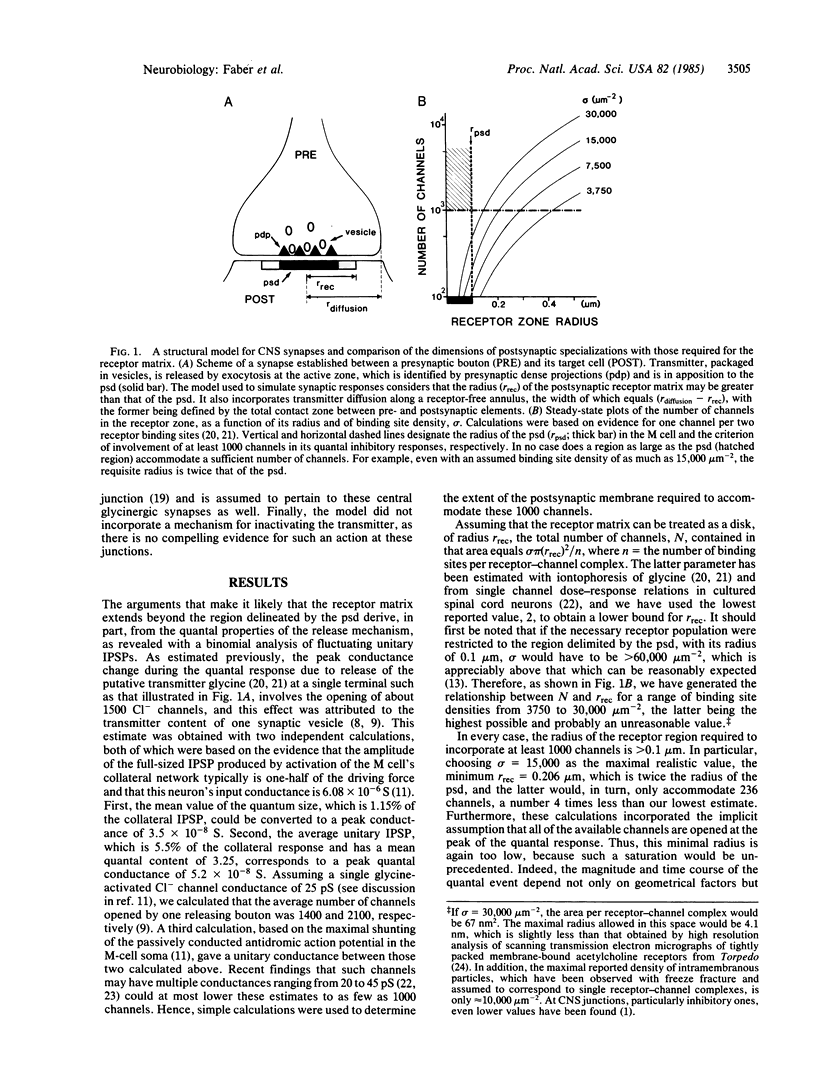
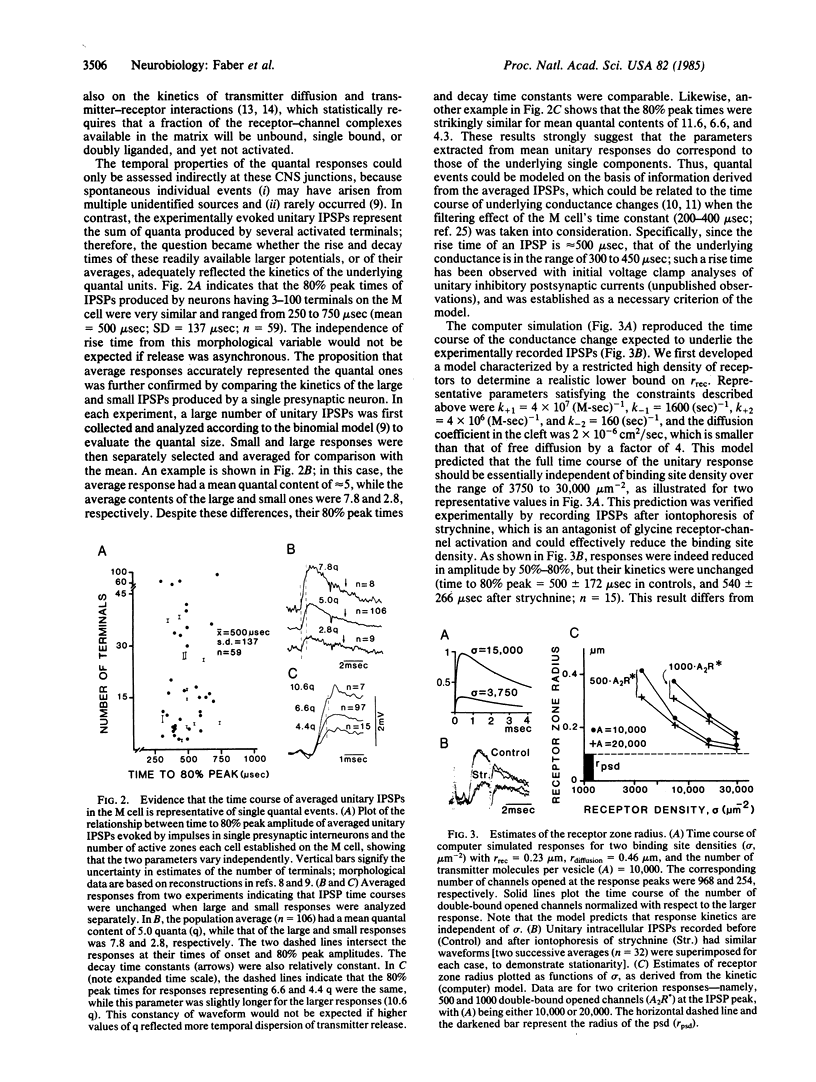
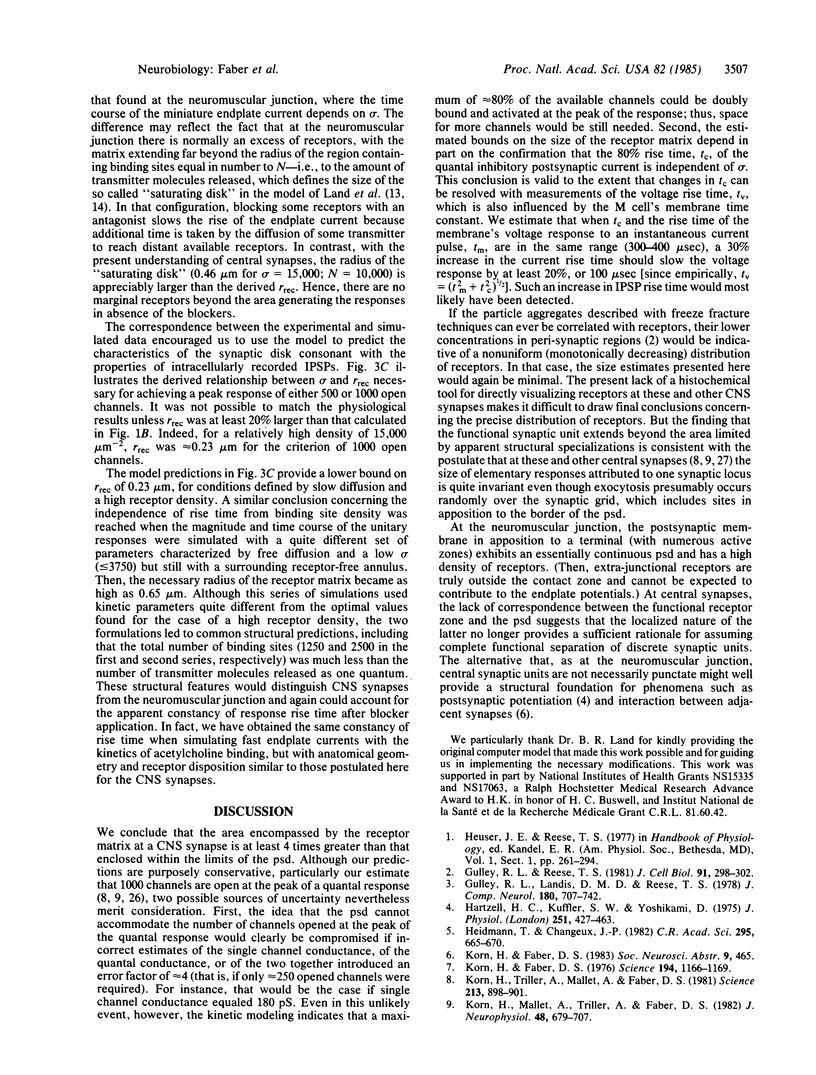
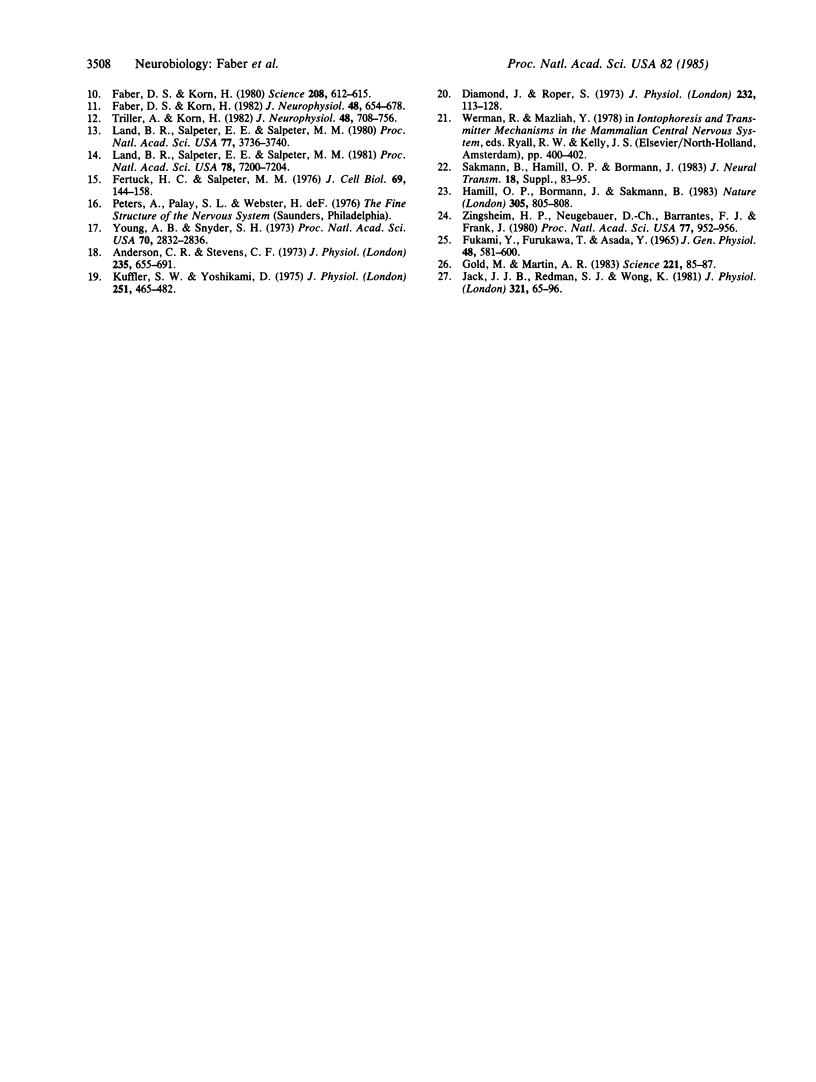
Selected References
These references are in PubMed. This may not be the complete list of references from this article.
- Anderson C. R., Stevens C. F. Voltage clamp analysis of acetylcholine produced end-plate current fluctuations at frog neuromuscular junction. J Physiol. 1973 Dec;235(3):655–691. doi: 10.1113/jphysiol.1973.sp010410. [DOI] [PMC free article] [PubMed] [Google Scholar]
- Diamond J., Roper S. Analysis of Mauthner cell responses to iontophoretically delivered pulses of GABA, glycine and L-glutamate. J Physiol. 1973 Jul;232(1):113–128. doi: 10.1113/jphysiol.1973.sp010259. [DOI] [PMC free article] [PubMed] [Google Scholar]
- FUKAMI Y., FURUKAWA T., ASADA Y. EXCITABILITY CHANGES OF THE MAUTHNER CELL DURING COLLATERAL INHIBITION. J Gen Physiol. 1965 Mar;48:581–600. doi: 10.1085/jgp.48.4.581. [DOI] [PMC free article] [PubMed] [Google Scholar]
- Faber D. S., Korn H. Single-shot channel activation accounts for duration of inhibitory postsynaptic potentials in a central neuron. Science. 1980 May 9;208(4444):612–615. doi: 10.1126/science.6245449. [DOI] [PubMed] [Google Scholar]
- Faber D. S., Korn H. Transmission at a central inhibitory synapse. I. Magnitude of unitary postsynaptic conductance change and kinetics of channel activation. J Neurophysiol. 1982 Sep;48(3):654–678. doi: 10.1152/jn.1982.48.3.654. [DOI] [PubMed] [Google Scholar]
- Fertuck H. C., Salpeter M. M. Quantitation of junctional and extrajunctional acetylcholine receptors by electron microscope autoradiography after 125I-alpha-bungarotoxin binding at mouse neuromuscular junctions. J Cell Biol. 1976 Apr;69(1):144–158. doi: 10.1083/jcb.69.1.144. [DOI] [PMC free article] [PubMed] [Google Scholar]
- Gold M. R., Martin A. R. Inhibitory conductance changes at synapses in the lamprey brainstem. Science. 1983 Jul 1;221(4605):85–87. doi: 10.1126/science.6857271. [DOI] [PubMed] [Google Scholar]
- Gulley R. L., Landis D. M., Reese T. S. Internal organization of membranes at end bulbs of Held in the anteroventral cochlear nucleus. J Comp Neurol. 1978 Aug 15;180(4):707–741. doi: 10.1002/cne.901800405. [DOI] [PubMed] [Google Scholar]
- Gulley R. L., Reese T. S. Cytoskeletal organization at the postsynaptic complex. J Cell Biol. 1981 Oct;91(1):298–302. doi: 10.1083/jcb.91.1.298. [DOI] [PMC free article] [PubMed] [Google Scholar]
- Hamill O. P., Bormann J., Sakmann B. Activation of multiple-conductance state chloride channels in spinal neurones by glycine and GABA. 1983 Oct 27-Nov 2Nature. 305(5937):805–808. doi: 10.1038/305805a0. [DOI] [PubMed] [Google Scholar]
- Hartzell H. C., Kuffler S. W., Yoshikami D. Post-synaptic potentiation: interaction between quanta of acetylcholine at the skeletal neuromuscular synapse. J Physiol. 1975 Oct;251(2):427–463. doi: 10.1113/jphysiol.1975.sp011102. [DOI] [PMC free article] [PubMed] [Google Scholar]
- Heidmann T., Changeux J. P. Un modèle moléculaire de régulation d'efficacité au niveau postsynaptique d'une synapse chimique. C R Seances Acad Sci III. 1982 Dec 6;295(12):665–670. [PubMed] [Google Scholar]
- Jack J. J., Redman S. J., Wong K. The components of synaptic potentials evoked in cat spinal motoneurones by impulses in single group Ia afferents. J Physiol. 1981 Dec;321:65–96. doi: 10.1113/jphysiol.1981.sp013972. [DOI] [PMC free article] [PubMed] [Google Scholar]
- Korn H., Faber D. S. Vertebrate central nervous system: same neurons mediate both electrical and chemical inhibitions. Science. 1976 Dec 10;194(4270):1166–1169. doi: 10.1126/science.186868. [DOI] [PubMed] [Google Scholar]
- Korn H., Mallet A., Triller A., Faber D. S. Transmission at a central inhibitory synapse. II. Quantal description of release, with a physical correlate for binomial n. J Neurophysiol. 1982 Sep;48(3):679–707. doi: 10.1152/jn.1982.48.3.679. [DOI] [PubMed] [Google Scholar]
- Korn H., Triller A., Mallet A., Faber D. S. Fluctuating responses at a central synapse: n of binomial fit predicts number of stained presynaptic boutons. Science. 1981 Aug 21;213(4510):898–901. doi: 10.1126/science.6266015. [DOI] [PubMed] [Google Scholar]
- Kuffler S. W., Yoshikami D. The number of transmitter molecules in a quantum: an estimate from iontophoretic application of acetylcholine at the neuromuscular synapse. J Physiol. 1975 Oct;251(2):465–482. doi: 10.1113/jphysiol.1975.sp011103. [DOI] [PMC free article] [PubMed] [Google Scholar]
- Land B. R., Salpeter E. E., Salpeter M. M. Acetylcholine receptor site density affects the rising phase of miniature endplate currents. Proc Natl Acad Sci U S A. 1980 Jun;77(6):3736–3740. doi: 10.1073/pnas.77.6.3736. [DOI] [PMC free article] [PubMed] [Google Scholar]
- Land B. R., Salpeter E. E., Salpeter M. M. Kinetic parameters for acetylcholine interaction in intact neuromuscular junction. Proc Natl Acad Sci U S A. 1981 Nov;78(11):7200–7204. doi: 10.1073/pnas.78.11.7200. [DOI] [PMC free article] [PubMed] [Google Scholar]
- Sakmann B., Hamill O. P., Bormann J. Patch-clamp measurements of elementary chloride currents activated by the putative inhibitory transmitter GABA and glycine in mammalian spinal neurons. J Neural Transm Suppl. 1983;18:83–95. [PubMed] [Google Scholar]
- Triller A., Korn H. Transmission at a central inhibitory synapse. III. Ultrastructure of physiologically identified and stained terminals. J Neurophysiol. 1982 Sep;48(3):708–736. doi: 10.1152/jn.1982.48.3.708. [DOI] [PubMed] [Google Scholar]
- Young A. B., Snyder S. H. Strychnine binding associated with glycine receptors of the central nervous system. Proc Natl Acad Sci U S A. 1973 Oct;70(10):2832–2836. doi: 10.1073/pnas.70.10.2832. [DOI] [PMC free article] [PubMed] [Google Scholar]
- Zingsheim H. P., Neugebauer D. C., Barrantes F. J., Frank J. Structural details of membrane-bound acetylcholine receptor from Tropedo marmorata. Proc Natl Acad Sci U S A. 1980 Feb;77(2):952–956. doi: 10.1073/pnas.77.2.952. [DOI] [PMC free article] [PubMed] [Google Scholar]