Abstract
The insulin responsiveness of the membrane transport system for glucose (2-deoxy-D-glucose) in diaphragm was measured during postnatal development of the rat. At birth, the basal rate of 2-deoxy-D-glucose transport is 3 nmol/min X g and it gradually decreases to 1 nmol/min X g over a period of 40 days. On the other hand, the insulin-stimulated rate of transport is 6 nmol/min X g at birth, it increases to 9 nmol/min X g in 16- to 20-day-old rats, and it decreases again to approximately 4 nmol/min X g in the 40-day-old rats. The stimulation of 2-deoxy-D-glucose transport by insulin is 2-fold at birth and increases to 4- to 5-fold 20 days after birth. The number of insulin receptors in the plasma membrane and the number of intracellular glucose transporters was also measured as a function of age to determine if there might be a correlation between these components of the insulin responsive system and the development of the increased insulin stimulation of 2-deoxy-D-glucose transport. The number of insulin receptors per g of wet weight decreased continuously with increasing age; the diaphragm of 40-day-old rats had about 50% of the receptors present in the diaphragm of the newborn rat. Similarly, the number of intracellular D-glucose transporters per g of wet weight decreased with increasing age; for adult rats, the number of transporters per g of diaphragm was 60% of that of newborn rats. The results indicate that the extent of insulin stimulation of glucose (2-deoxy-D-glucose) transport in the diaphragm during the first 20 days of life is not directly or simply related to the number of insulin receptors or the number of intracellular glucose transporters. The extent of the insulin response depends on some other factor that activates or is part of the machinery for translocation of the transporter.
Full text
PDF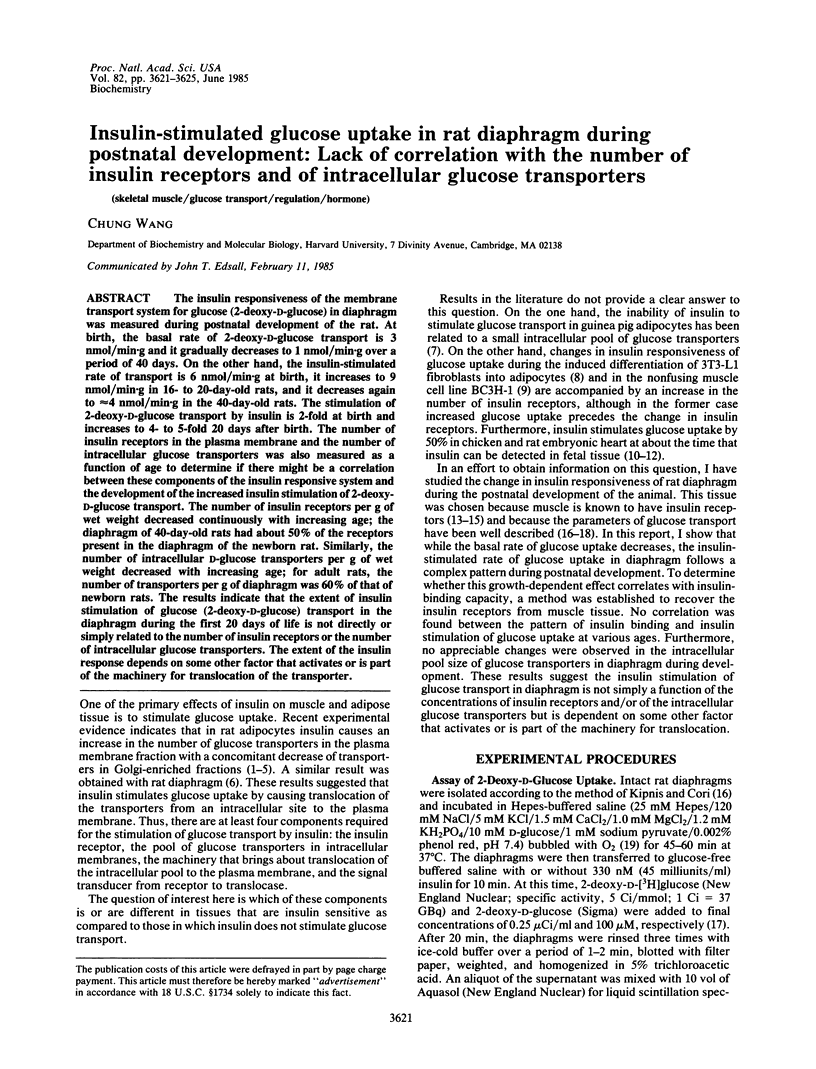
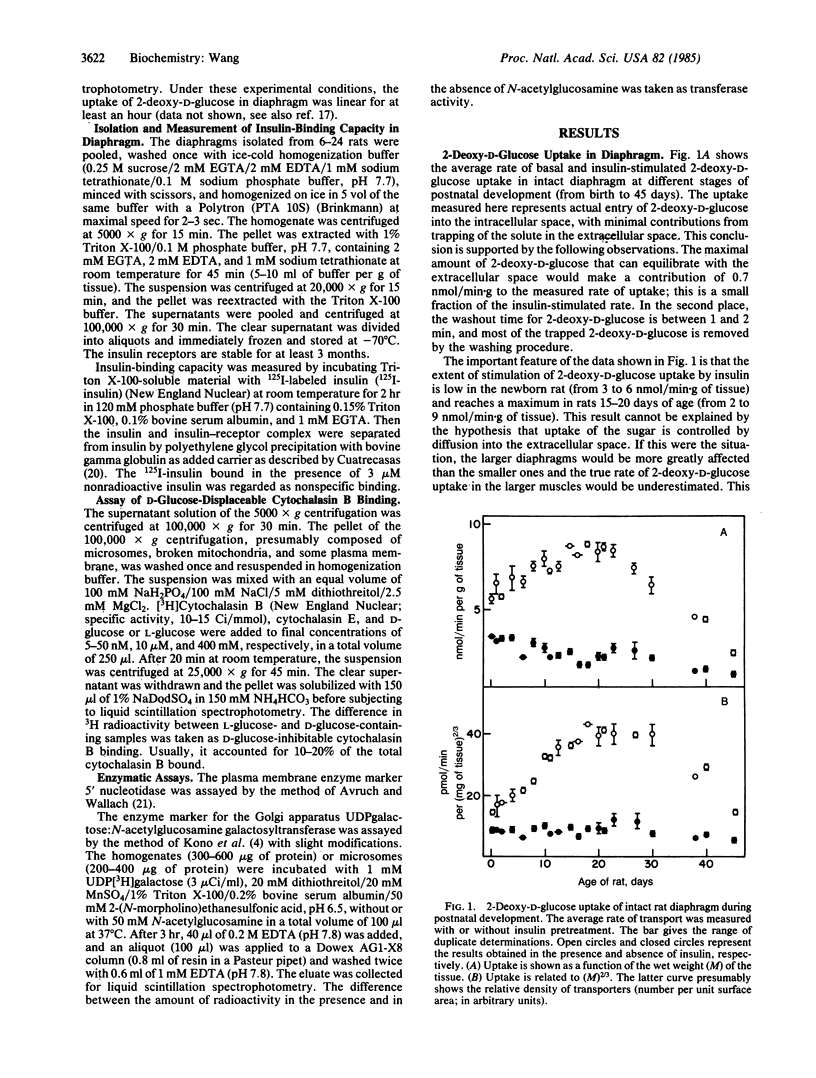
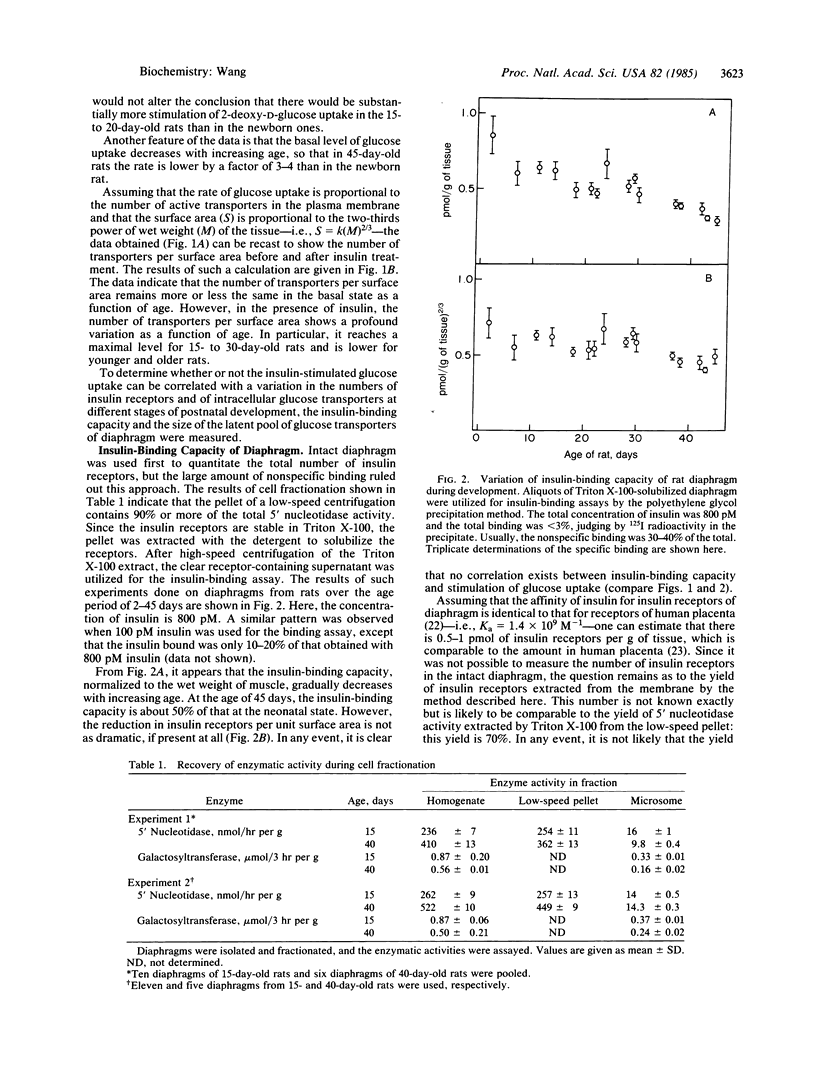
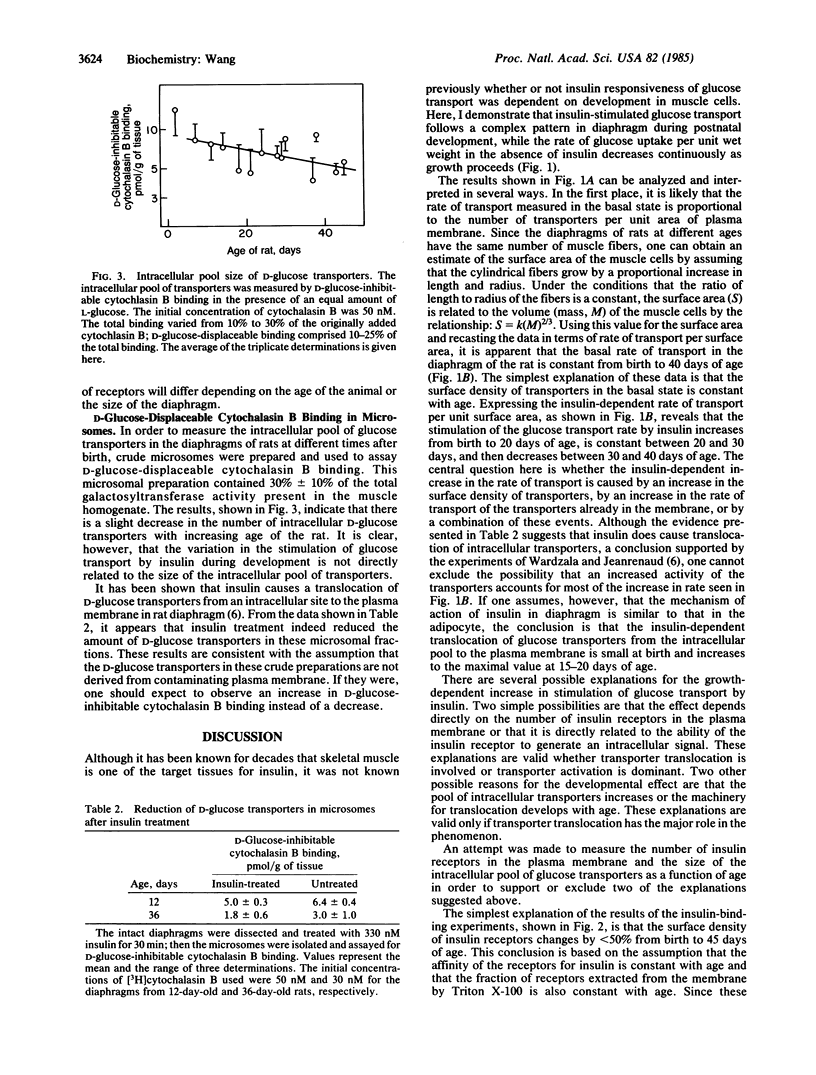
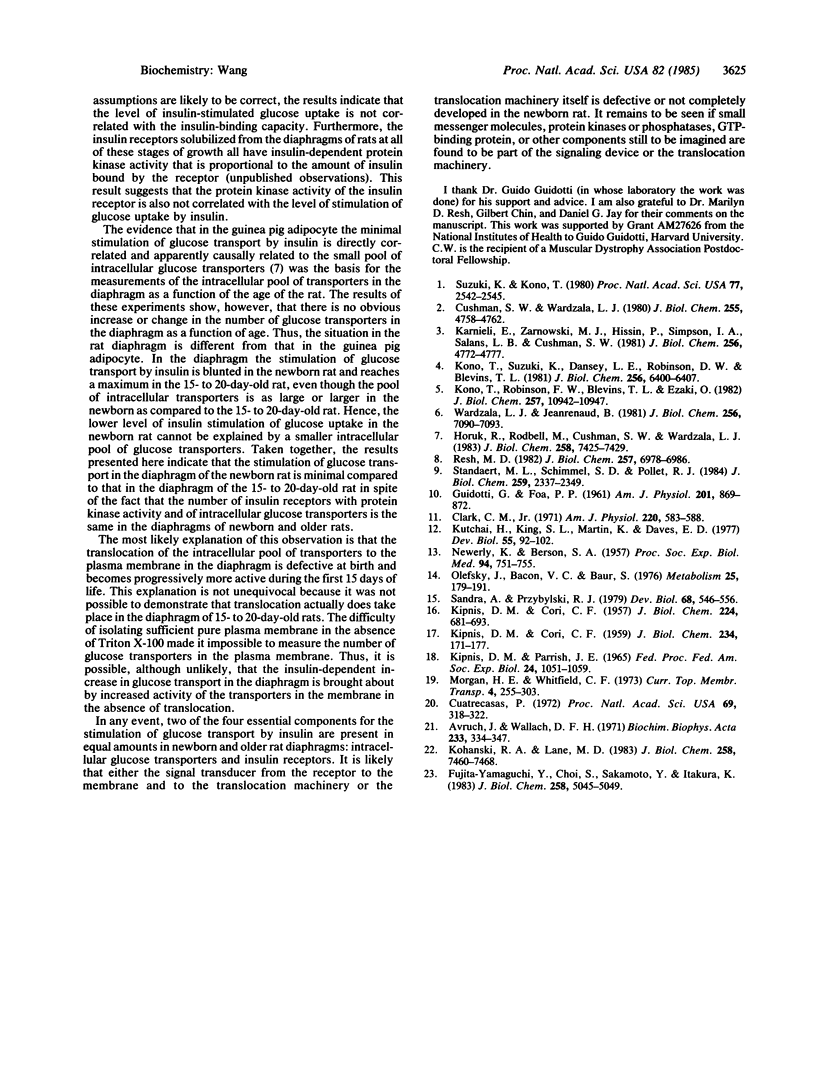
Selected References
These references are in PubMed. This may not be the complete list of references from this article.
- Avruch J., Wallach D. F. Preparation and properties of plasma membrane and endoplasmic reticulum fragments from isolated rat fat cells. Biochim Biophys Acta. 1971 Apr 13;233(2):334–347. doi: 10.1016/0005-2736(71)90331-2. [DOI] [PubMed] [Google Scholar]
- Clark C. M., Jr Carbohydrate metabolism in the isolated fetal rat heart. Am J Physiol. 1971 Mar;220(3):583–588. doi: 10.1152/ajplegacy.1971.220.3.583. [DOI] [PubMed] [Google Scholar]
- Cuatrecasas P. Isolation of the insulin receptor of liver and fat-cell membranes (detergent-solubilized-( 125 I)insulin-polyethylene glycol precipitation-sephadex). Proc Natl Acad Sci U S A. 1972 Feb;69(2):318–322. doi: 10.1073/pnas.69.2.318. [DOI] [PMC free article] [PubMed] [Google Scholar]
- Cushman S. W., Wardzala L. J. Potential mechanism of insulin action on glucose transport in the isolated rat adipose cell. Apparent translocation of intracellular transport systems to the plasma membrane. J Biol Chem. 1980 May 25;255(10):4758–4762. [PubMed] [Google Scholar]
- Fujita-Yamaguchi Y., Choi S., Sakamoto Y., Itakura K. Purification of insulin receptor with full binding activity. J Biol Chem. 1983 Apr 25;258(8):5045–5049. [PubMed] [Google Scholar]
- Horuk R., Rodbell M., Cushman S. W., Wardzala L. J. Proposed mechanism of insulin-resistant glucose transport in the isolated guinea pig adipocyte. Small intracellular pool of glucose transporters. J Biol Chem. 1983 Jun 25;258(12):7425–7429. [PubMed] [Google Scholar]
- KIPNIS D. M., CORI C. F. Studies of tissue permeability. III. The effect of insulin on pentose uptake by the diaphragm. J Biol Chem. 1957 Feb;224(2):681–693. [PubMed] [Google Scholar]
- KIPNIS D. M., CORI C. F. Studies of tissue permeability. V. The penetration and phosphorylation of 2-deoxyglucose in the rat diaphragm. J Biol Chem. 1959 Jan;234(1):171–177. [PubMed] [Google Scholar]
- Karnieli E., Zarnowski M. J., Hissin P. J., Simpson I. A., Salans L. B., Cushman S. W. Insulin-stimulated translocation of glucose transport systems in the isolated rat adipose cell. Time course, reversal, insulin concentration dependency, and relationship to glucose transport activity. J Biol Chem. 1981 May 25;256(10):4772–4777. [PubMed] [Google Scholar]
- Kipnis D. M., Parrish J. E. Role of Na+ and K+ on sugar (2-deoxyglucose) and amino acid (alpha-aminoisobutyric acid) transport in striated muscle. Fed Proc. 1965 Sep-Oct;24(5):1051–1059. [PubMed] [Google Scholar]
- Kohanski R. A., Lane M. D. Binding of insulin to solubilized insulin receptor from human placenta. Evidence for a single class of noninteracting binding sites. J Biol Chem. 1983 Jun 25;258(12):7460–7468. [PubMed] [Google Scholar]
- Kono T., Robinson F. W., Blevins T. L., Ezaki O. Evidence that translocation of the glucose transport activity is the major mechanism of insulin action on glucose transport in fat cells. J Biol Chem. 1982 Sep 25;257(18):10942–10947. [PubMed] [Google Scholar]
- Kono T., Suzuki K., Dansey L. E., Robinson F. W., Blevins T. L. Energy-dependent and protein synthesis-independent recycling of the insulin-sensitive glucose transport mechanism in fat cells. J Biol Chem. 1981 Jun 25;256(12):6400–6407. [PubMed] [Google Scholar]
- Kutchai H., King S. L., Martin M., Daves E. D. Glucose uptake by chicken embryo hearts at various stages of development. Dev Biol. 1977 Jan;55(1):92–102. doi: 10.1016/0012-1606(77)90322-0. [DOI] [PubMed] [Google Scholar]
- NEWERLY K., BERSON S. A. Lack of specificity of insulin-I 131-binding by isolated rat diaphragm. Proc Soc Exp Biol Med. 1957 Apr;94(4):751–755. doi: 10.3181/00379727-94-23075. [DOI] [PubMed] [Google Scholar]
- Olefsky J., Bacon V. C., Baur S. Insulin receptors of skeletal muscle: specific insulin binding sites and demonstration of decreased numbers of sites in obese rats. Metabolism. 1976 Feb;25(2):179–191. doi: 10.1016/0026-0495(76)90048-2. [DOI] [PubMed] [Google Scholar]
- Resh M. D. Development of insulin responsiveness of the glucose transporter and the (Na+,K+)-adenosine triphosphatase during in vitro adipocyte differentiation. J Biol Chem. 1982 Jun 25;257(12):6978–6986. [PubMed] [Google Scholar]
- Sandra A., Przybylski R. J. Ontogeny of insulin binding during chick skeletal myogenesis in vitro. Dev Biol. 1979 Feb;68(2):546–556. doi: 10.1016/0012-1606(79)90225-2. [DOI] [PubMed] [Google Scholar]
- Standaert M. L., Schimmel S. D., Pollet R. J. The development of insulin receptors and responses in the differentiating nonfusing muscle cell line BC3H-1. J Biol Chem. 1984 Feb 25;259(4):2337–2345. [PubMed] [Google Scholar]
- Suzuki K., Kono T. Evidence that insulin causes translocation of glucose transport activity to the plasma membrane from an intracellular storage site. Proc Natl Acad Sci U S A. 1980 May;77(5):2542–2545. doi: 10.1073/pnas.77.5.2542. [DOI] [PMC free article] [PubMed] [Google Scholar]
- Wardzala L. J., Jeanrenaud B. Potential mechanism of insulin action on glucose transport in the isolated rat diaphragm. Apparent translocation of intracellular transport units to the plasma membrane. J Biol Chem. 1981 Jul 25;256(14):7090–7093. [PubMed] [Google Scholar]