Abstract
Engineering site-specific amino acid substitutions into the protein-tyrosine phosphatase (PTPase) PTP1 and the dual-specific vaccinia H1-related phosphatase (VHR), has kinetically isolated the two chemical steps of the reaction and provided a rare opportunity for examining transition states and directly observing the phosphoenzyme intermediate. Changing serine to alanine in the active-site sequence motif HCXXGXXRS shifted the rate-limiting step from intermediate formation to intermediate hydrolysis. Using phosphorus 31P NMR, the covalent thiol-phosphate intermediate was directly observed during catalytic turnover. The importance of the conserved aspartic acid (D92 in VHR and D181 in PTP1) in both chemical steps was established. Kinetic analysis of D92N and D181N mutants indicated that aspartic acid acts as a general acid by protonating the leaving-group phenolic oxygen. Structure-reactivity experiments with native and aspartate mutant enzymes established that proton transfer is concomitant with P-O cleavage, such that no charge develops on the phenolic oxygen. Steady- and presteady-state kinetics, as well as NMR analysis of the double mutant D92N/S131A (VHR), suggested that the conserved aspartic acid functions as a general base during intermediate hydrolysis. As a general base, aspartate would activate a water molecule to facilitate nucleophilic attack. The amino acids involved in transition-state stabilization for cysteinylphosphate hydrolysis were confirmed by the x-ray structure of the Yersinia PTPase complexed with vanadate, a transition-state mimic that binds covalently to the active-site cysteine. Consistent with the NMR, x-ray, biochemical, and kinetic data, a unifying mechanism for catalysis is proposed.
Full text
PDF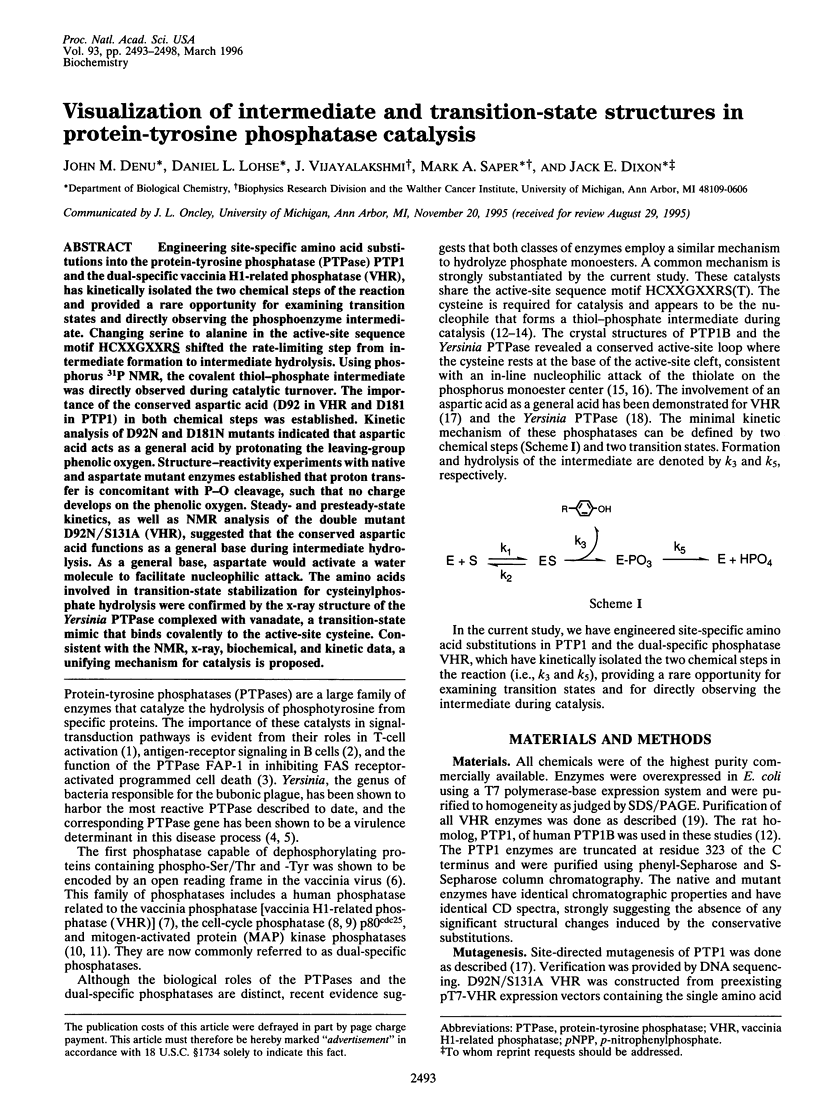
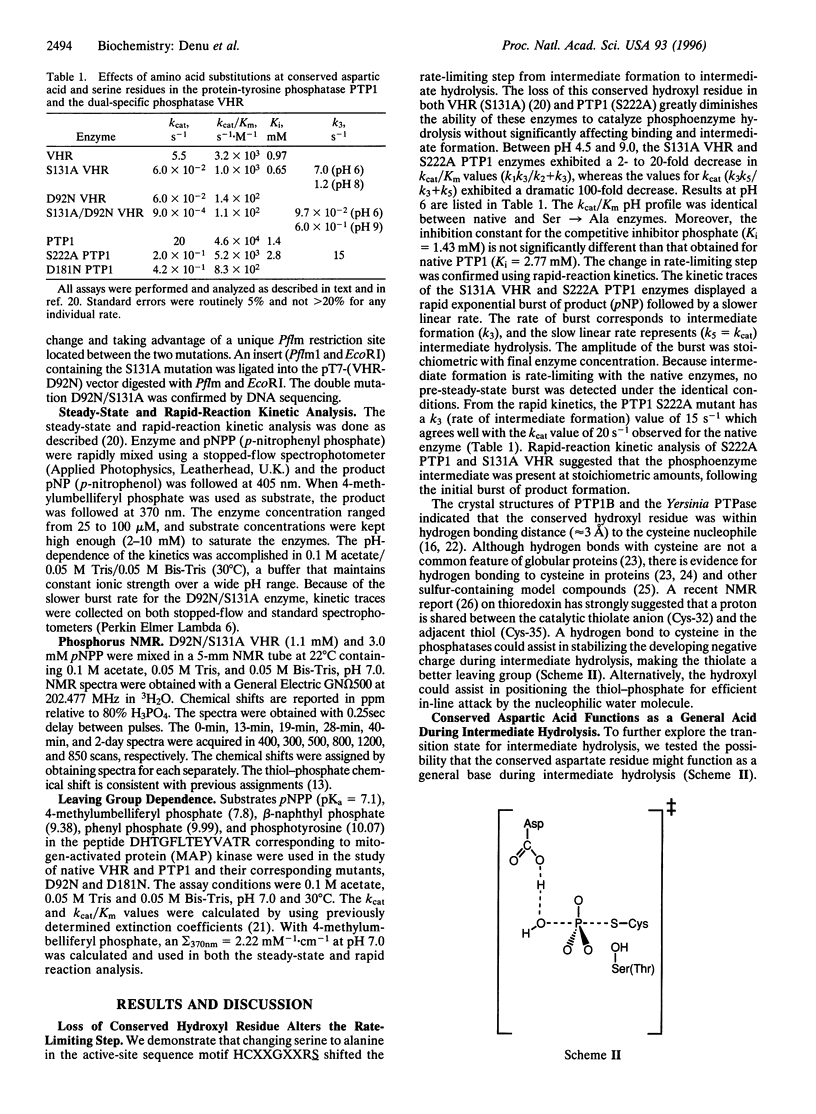
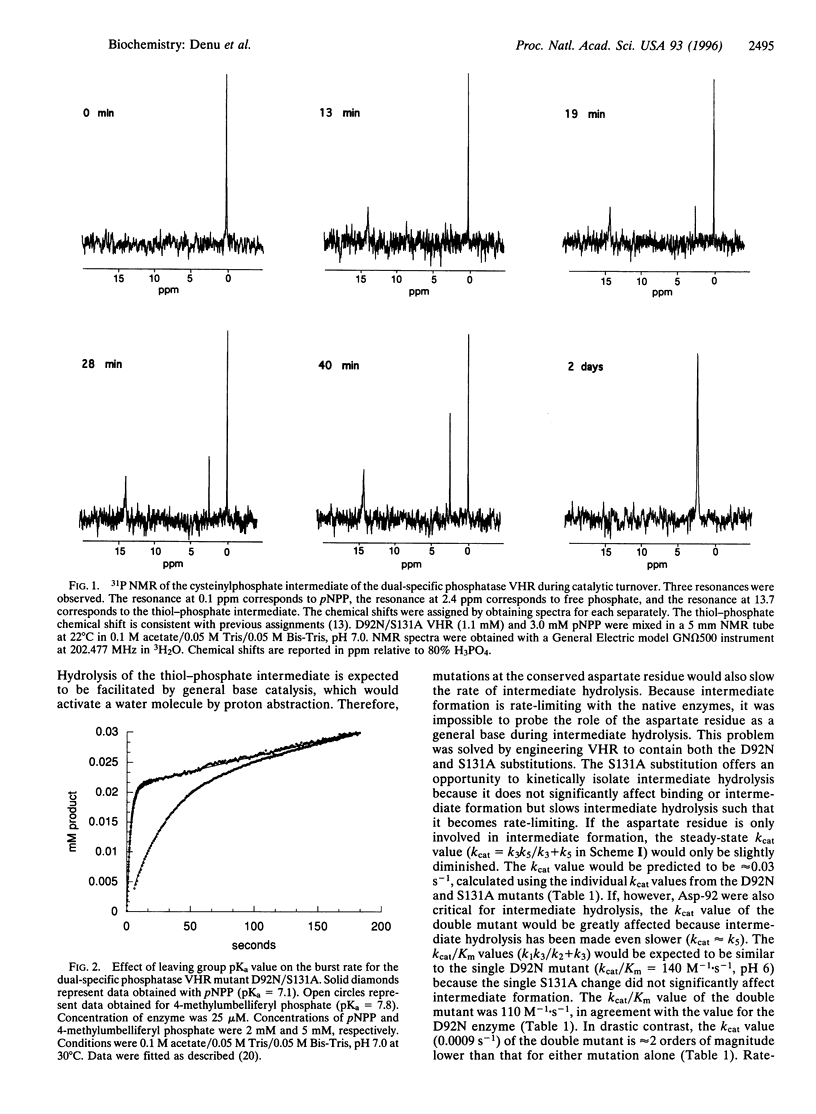
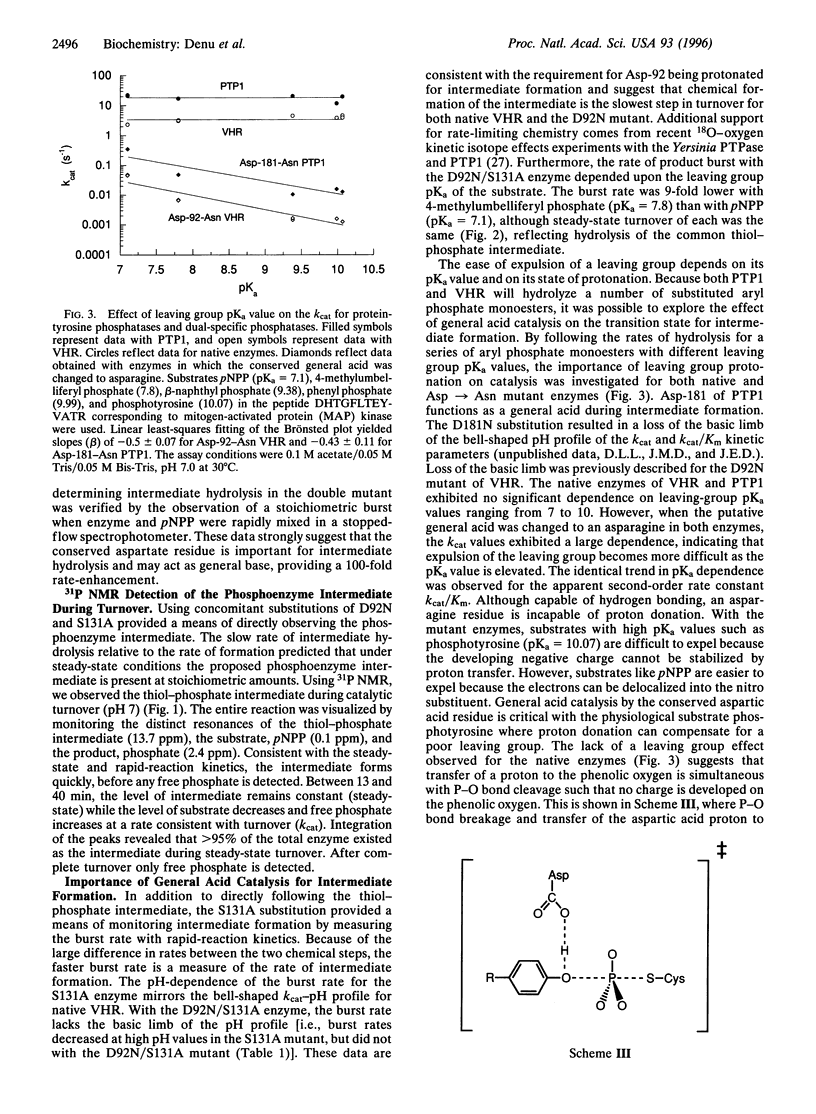
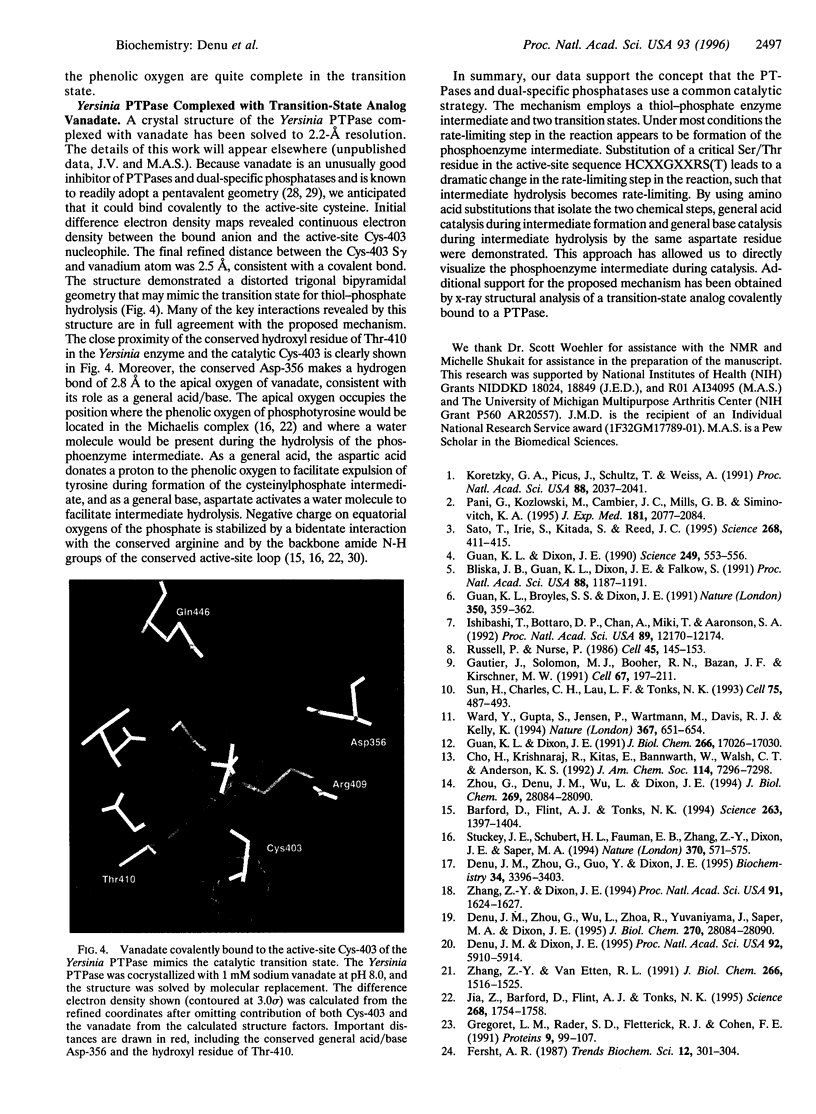
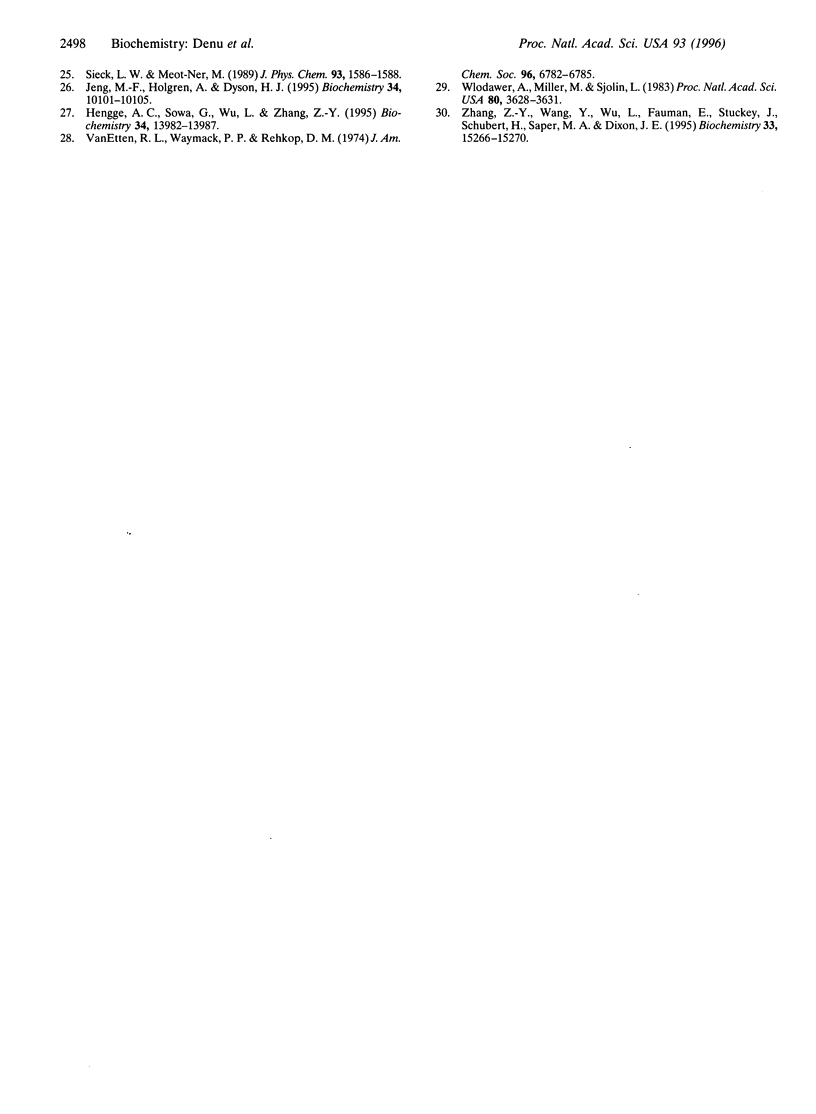
Images in this article
Selected References
These references are in PubMed. This may not be the complete list of references from this article.
- Barford D., Flint A. J., Tonks N. K. Crystal structure of human protein tyrosine phosphatase 1B. Science. 1994 Mar 11;263(5152):1397–1404. [PubMed] [Google Scholar]
- Bliska J. B., Guan K. L., Dixon J. E., Falkow S. Tyrosine phosphate hydrolysis of host proteins by an essential Yersinia virulence determinant. Proc Natl Acad Sci U S A. 1991 Feb 15;88(4):1187–1191. doi: 10.1073/pnas.88.4.1187. [DOI] [PMC free article] [PubMed] [Google Scholar]
- Denu J. M., Dixon J. E. A catalytic mechanism for the dual-specific phosphatases. Proc Natl Acad Sci U S A. 1995 Jun 20;92(13):5910–5914. doi: 10.1073/pnas.92.13.5910. [DOI] [PMC free article] [PubMed] [Google Scholar]
- Denu J. M., Zhou G., Guo Y., Dixon J. E. The catalytic role of aspartic acid-92 in a human dual-specific protein-tyrosine-phosphatase. Biochemistry. 1995 Mar 14;34(10):3396–3403. doi: 10.1021/bi00010a031. [DOI] [PubMed] [Google Scholar]
- Gautier J., Solomon M. J., Booher R. N., Bazan J. F., Kirschner M. W. cdc25 is a specific tyrosine phosphatase that directly activates p34cdc2. Cell. 1991 Oct 4;67(1):197–211. doi: 10.1016/0092-8674(91)90583-k. [DOI] [PubMed] [Google Scholar]
- Gregoret L. M., Rader S. D., Fletterick R. J., Cohen F. E. Hydrogen bonds involving sulfur atoms in proteins. Proteins. 1991;9(2):99–107. doi: 10.1002/prot.340090204. [DOI] [PubMed] [Google Scholar]
- Guan K. L., Broyles S. S., Dixon J. E. A Tyr/Ser protein phosphatase encoded by vaccinia virus. Nature. 1991 Mar 28;350(6316):359–362. doi: 10.1038/350359a0. [DOI] [PubMed] [Google Scholar]
- Guan K. L., Dixon J. E. Evidence for protein-tyrosine-phosphatase catalysis proceeding via a cysteine-phosphate intermediate. J Biol Chem. 1991 Sep 15;266(26):17026–17030. [PubMed] [Google Scholar]
- Guan K. L., Dixon J. E. Protein tyrosine phosphatase activity of an essential virulence determinant in Yersinia. Science. 1990 Aug 3;249(4968):553–556. doi: 10.1126/science.2166336. [DOI] [PubMed] [Google Scholar]
- Hengge A. C., Sowa G. A., Wu L., Zhang Z. Y. Nature of the transition state of the protein-tyrosine phosphatase-catalyzed reaction. Biochemistry. 1995 Oct 31;34(43):13982–13987. doi: 10.1021/bi00043a003. [DOI] [PubMed] [Google Scholar]
- Ishibashi T., Bottaro D. P., Chan A., Miki T., Aaronson S. A. Expression cloning of a human dual-specificity phosphatase. Proc Natl Acad Sci U S A. 1992 Dec 15;89(24):12170–12174. doi: 10.1073/pnas.89.24.12170. [DOI] [PMC free article] [PubMed] [Google Scholar]
- Jeng M. F., Holmgren A., Dyson H. J. Proton sharing between cysteine thiols in Escherichia coli thioredoxin: implications for the mechanism of protein disulfide reduction. Biochemistry. 1995 Aug 15;34(32):10101–10105. doi: 10.1021/bi00032a001. [DOI] [PubMed] [Google Scholar]
- Jia Z., Barford D., Flint A. J., Tonks N. K. Structural basis for phosphotyrosine peptide recognition by protein tyrosine phosphatase 1B. Science. 1995 Jun 23;268(5218):1754–1758. doi: 10.1126/science.7540771. [DOI] [PubMed] [Google Scholar]
- Koretzky G. A., Picus J., Schultz T., Weiss A. Tyrosine phosphatase CD45 is required for T-cell antigen receptor and CD2-mediated activation of a protein tyrosine kinase and interleukin 2 production. Proc Natl Acad Sci U S A. 1991 Mar 15;88(6):2037–2041. doi: 10.1073/pnas.88.6.2037. [DOI] [PMC free article] [PubMed] [Google Scholar]
- Pani G., Kozlowski M., Cambier J. C., Mills G. B., Siminovitch K. A. Identification of the tyrosine phosphatase PTP1C as a B cell antigen receptor-associated protein involved in the regulation of B cell signaling. J Exp Med. 1995 Jun 1;181(6):2077–2084. doi: 10.1084/jem.181.6.2077. [DOI] [PMC free article] [PubMed] [Google Scholar]
- Russell P., Nurse P. cdc25+ functions as an inducer in the mitotic control of fission yeast. Cell. 1986 Apr 11;45(1):145–153. doi: 10.1016/0092-8674(86)90546-5. [DOI] [PubMed] [Google Scholar]
- Sato T., Irie S., Kitada S., Reed J. C. FAP-1: a protein tyrosine phosphatase that associates with Fas. Science. 1995 Apr 21;268(5209):411–415. doi: 10.1126/science.7536343. [DOI] [PubMed] [Google Scholar]
- Stuckey J. A., Schubert H. L., Fauman E. B., Zhang Z. Y., Dixon J. E., Saper M. A. Crystal structure of Yersinia protein tyrosine phosphatase at 2.5 A and the complex with tungstate. Nature. 1994 Aug 18;370(6490):571–575. doi: 10.1038/370571a0. [DOI] [PubMed] [Google Scholar]
- Sun H., Charles C. H., Lau L. F., Tonks N. K. MKP-1 (3CH134), an immediate early gene product, is a dual specificity phosphatase that dephosphorylates MAP kinase in vivo. Cell. 1993 Nov 5;75(3):487–493. doi: 10.1016/0092-8674(93)90383-2. [DOI] [PubMed] [Google Scholar]
- Ward Y., Gupta S., Jensen P., Wartmann M., Davis R. J., Kelly K. Control of MAP kinase activation by the mitogen-induced threonine/tyrosine phosphatase PAC1. Nature. 1994 Feb 17;367(6464):651–654. doi: 10.1038/367651a0. [DOI] [PubMed] [Google Scholar]
- Wlodawer A., Miller M., Sjölin L. Active site of RNase: neutron diffraction study of a complex with uridine vanadate, a transition-state analog. Proc Natl Acad Sci U S A. 1983 Jun;80(12):3628–3631. doi: 10.1073/pnas.80.12.3628. [DOI] [PMC free article] [PubMed] [Google Scholar]
- Xie J., Drumm M. L., Ma J., Davis P. B. Intracellular loop between transmembrane segments IV and V of cystic fibrosis transmembrane conductance regulator is involved in regulation of chloride channel conductance state. J Biol Chem. 1995 Nov 24;270(47):28084–28091. doi: 10.1074/jbc.270.47.28084. [DOI] [PubMed] [Google Scholar]
- Zhang Z. Y., VanEtten R. L. Pre-steady-state and steady-state kinetic analysis of the low molecular weight phosphotyrosyl protein phosphatase from bovine heart. J Biol Chem. 1991 Jan 25;266(3):1516–1525. [PubMed] [Google Scholar]
- Zhang Z. Y., Wang Y., Dixon J. E. Dissecting the catalytic mechanism of protein-tyrosine phosphatases. Proc Natl Acad Sci U S A. 1994 Mar 1;91(5):1624–1627. doi: 10.1073/pnas.91.5.1624. [DOI] [PMC free article] [PubMed] [Google Scholar]
- Zhang Z. Y., Wang Y., Wu L., Fauman E. B., Stuckey J. A., Schubert H. L., Saper M. A., Dixon J. E. The Cys(X)5Arg catalytic motif in phosphoester hydrolysis. Biochemistry. 1994 Dec 27;33(51):15266–15270. doi: 10.1021/bi00255a007. [DOI] [PubMed] [Google Scholar]
- Zhou G., Denu J. M., Wu L., Dixon J. E. The catalytic role of Cys124 in the dual specificity phosphatase VHR. J Biol Chem. 1994 Nov 11;269(45):28084–28090. [PubMed] [Google Scholar]