Abstract
Treatment of Escherichia coli glutamine synthetase (GS) with peroxynitrite leads to nitration of some tyrosine residues and conversion of some methionine residues to methionine sulfoxide (MSOX) residues. Nitration, but not MSOX formation, is stimulated by Fe-EDTA. In the absence of Fe-EDTA, nitration of only one tyrosine residue per subunit of unadenylylated GS leads to changes in divalent cation requirement, pH-activity profile, affinity for ADP, and susceptibility to feedback inhibition by end products (tryptophan, AMP, CTP), whereas nitration of one tyrosine residue per subunit in the adenylylated GS leads to complete loss of catalytic activity. In the presence of Fe-EDTA, nitration is a more random process: nitration of five to six tyrosine residues per subunit is needed to convert unadenylylated GS to the adenylylated configuration. These results and the fact that nitration of tyrosine residues is an irreversible process serve notice that the regulatory function of proteins that undergo phosphorylation or adenylylation in signal transduction cascades might be seriously compromised by peroxynitrite-promoted nitration.
Full text
PDF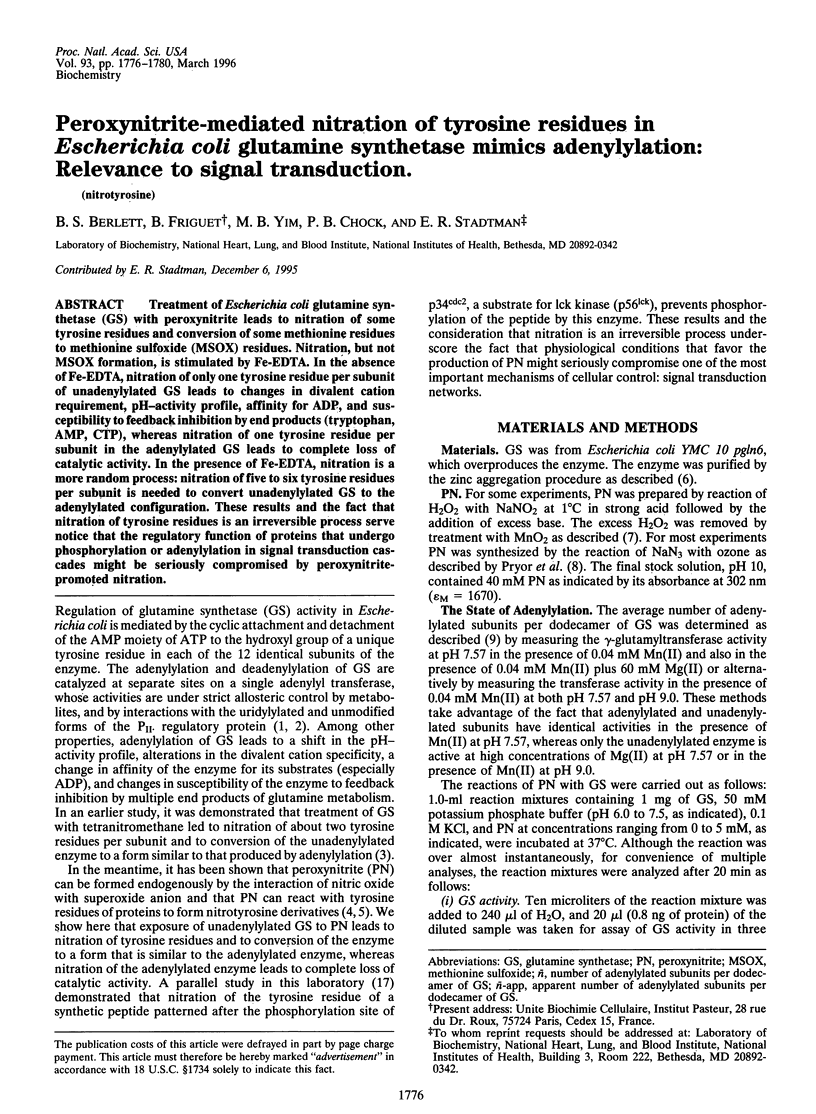
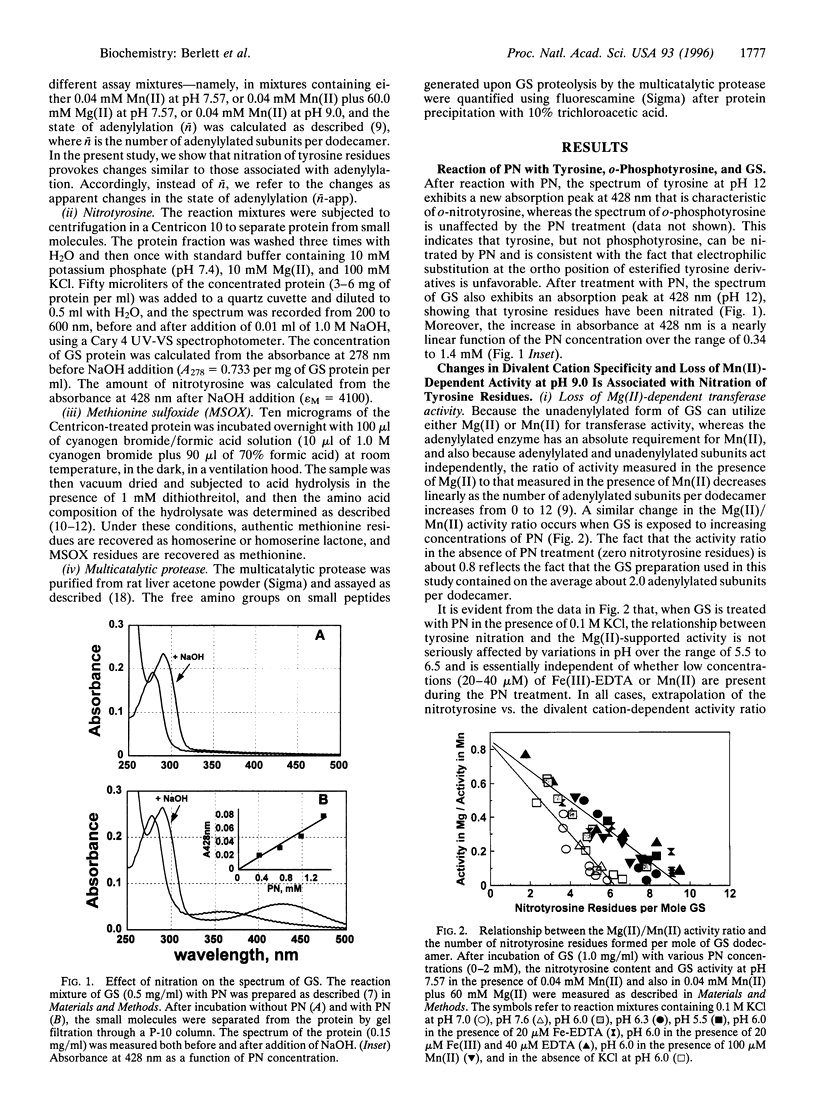
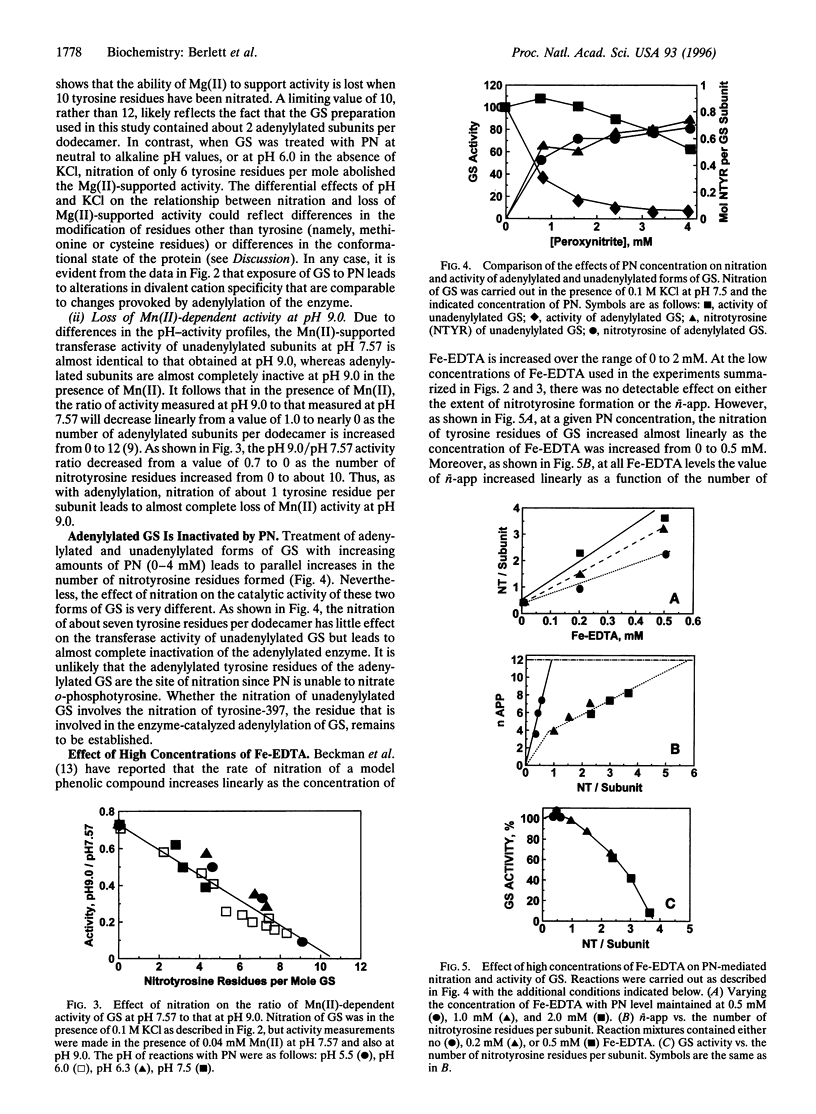
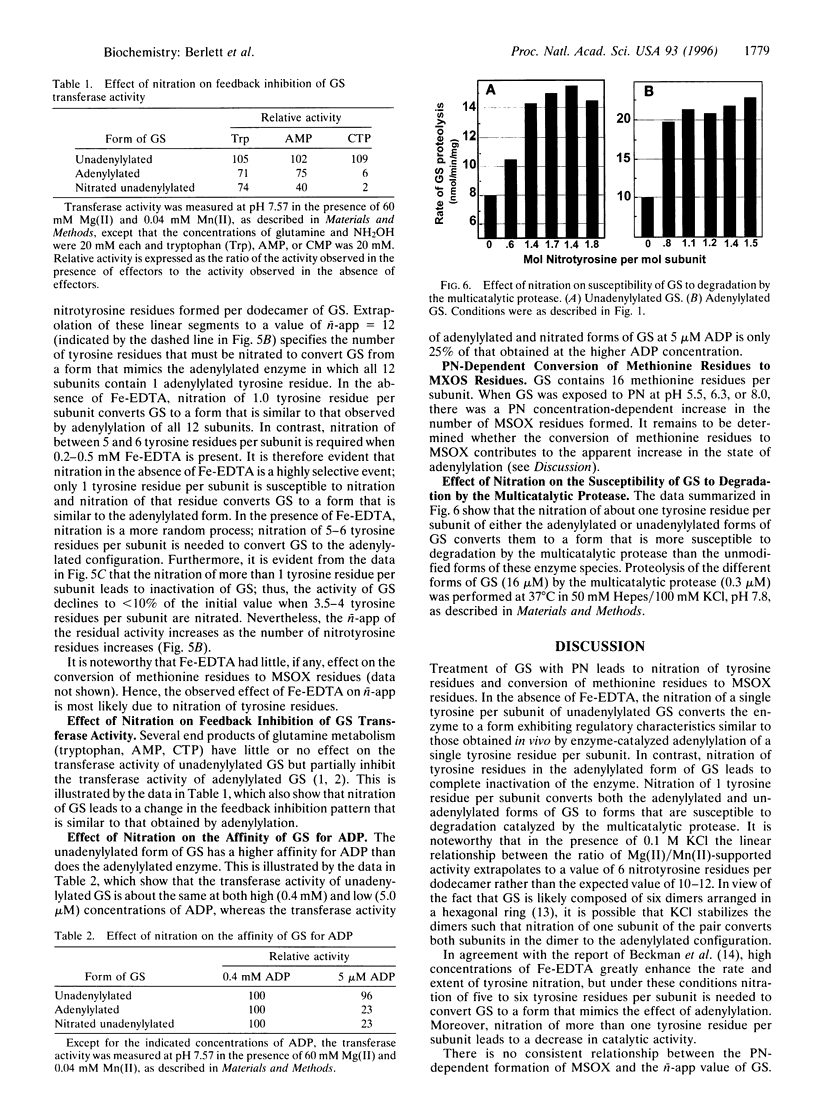
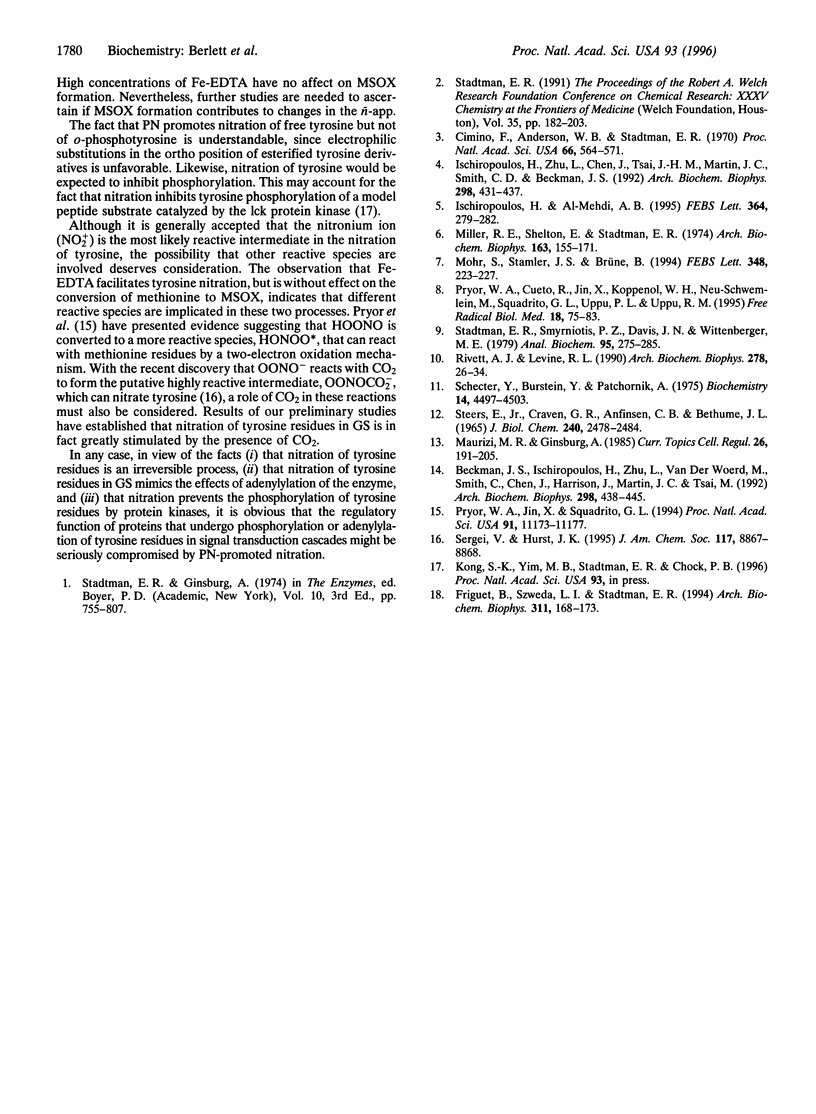
Images in this article
Selected References
These references are in PubMed. This may not be the complete list of references from this article.
- Beckman J. S., Ischiropoulos H., Zhu L., van der Woerd M., Smith C., Chen J., Harrison J., Martin J. C., Tsai M. Kinetics of superoxide dismutase- and iron-catalyzed nitration of phenolics by peroxynitrite. Arch Biochem Biophys. 1992 Nov 1;298(2):438–445. doi: 10.1016/0003-9861(92)90432-v. [DOI] [PubMed] [Google Scholar]
- Cimino F., Anderson W. B., Stadtman E. R. Ability of nonenzymic nitration or acetylation of E. coli glutamine synthetase to produce effects analogous to enzymic adenylylation. Proc Natl Acad Sci U S A. 1970 Jun;66(2):564–571. doi: 10.1073/pnas.66.2.564. [DOI] [PMC free article] [PubMed] [Google Scholar]
- Friguet B., Szweda L. I., Stadtman E. R. Susceptibility of glucose-6-phosphate dehydrogenase modified by 4-hydroxy-2-nonenal and metal-catalyzed oxidation to proteolysis by the multicatalytic protease. Arch Biochem Biophys. 1994 May 15;311(1):168–173. doi: 10.1006/abbi.1994.1222. [DOI] [PubMed] [Google Scholar]
- Ischiropoulos H., Zhu L., Chen J., Tsai M., Martin J. C., Smith C. D., Beckman J. S. Peroxynitrite-mediated tyrosine nitration catalyzed by superoxide dismutase. Arch Biochem Biophys. 1992 Nov 1;298(2):431–437. doi: 10.1016/0003-9861(92)90431-u. [DOI] [PubMed] [Google Scholar]
- Ischiropoulos H., al-Mehdi A. B. Peroxynitrite-mediated oxidative protein modifications. FEBS Lett. 1995 May 15;364(3):279–282. doi: 10.1016/0014-5793(95)00307-u. [DOI] [PubMed] [Google Scholar]
- Maurizi M. R., Ginsburg A. Active-site ligand binding and subunit interactions in glutamine synthetase from Escherichia coli. Curr Top Cell Regul. 1985;26:191–206. doi: 10.1016/b978-0-12-152826-3.50022-x. [DOI] [PubMed] [Google Scholar]
- Miller R. E., Shelton E., Stadtman E. R. Zinc-induced paracrystalline aggregation of glutamine synthetase. Arch Biochem Biophys. 1974 Jul;163(1):155–171. doi: 10.1016/0003-9861(74)90465-2. [DOI] [PubMed] [Google Scholar]
- Mohr S., Stamler J. S., Brüne B. Mechanism of covalent modification of glyceraldehyde-3-phosphate dehydrogenase at its active site thiol by nitric oxide, peroxynitrite and related nitrosating agents. FEBS Lett. 1994 Jul 18;348(3):223–227. doi: 10.1016/0014-5793(94)00596-6. [DOI] [PubMed] [Google Scholar]
- Pryor W. A., Cueto R., Jin X., Koppenol W. H., Ngu-Schwemlein M., Squadrito G. L., Uppu P. L., Uppu R. M. A practical method for preparing peroxynitrite solutions of low ionic strength and free of hydrogen peroxide. Free Radic Biol Med. 1995 Jan;18(1):75–83. doi: 10.1016/0891-5849(94)00105-s. [DOI] [PubMed] [Google Scholar]
- Pryor W. A., Jin X., Squadrito G. L. One- and two-electron oxidations of methionine by peroxynitrite. Proc Natl Acad Sci U S A. 1994 Nov 8;91(23):11173–11177. doi: 10.1073/pnas.91.23.11173. [DOI] [PMC free article] [PubMed] [Google Scholar]
- Rivett A. J., Levine R. L. Metal-catalyzed oxidation of Escherichia coli glutamine synthetase: correlation of structural and functional changes. Arch Biochem Biophys. 1990 Apr;278(1):26–34. doi: 10.1016/0003-9861(90)90226-o. [DOI] [PubMed] [Google Scholar]
- STEERS E., Jr, CRAVEN G. R., ANFINSEN C. B., BETHUNE J. L. EVIDENCE FOR NONIDENTICAL CHAINS IN THE BETA-GALACTOSIDASE OF ESCHERICHIA COLI K12. J Biol Chem. 1965 Jun;240:2478–2484. [PubMed] [Google Scholar]
- Shechter Y., Burstein Y., Patchornik A. Selective oxidation of methionine residues in proteins. Biochemistry. 1975 Oct 7;14(20):4497–4503. doi: 10.1021/bi00691a025. [DOI] [PubMed] [Google Scholar]
- Stadtman E. R., Smyrniotis P. Z., Davis J. N., Wittenberger M. E. Enzymic procedures for determining the average state of adenylylation of Escherichia coli glutamine synthetase. Anal Biochem. 1979 May;95(1):275–285. doi: 10.1016/0003-2697(79)90217-3. [DOI] [PubMed] [Google Scholar]