Abstract
Competing hypotheses seek to explain the evolution of oxygenic and anoxygenic processes of photosynthesis. Since chlorophyll is less reduced and precedes bacteriochlorophyll on the modern biosynthetic pathway, it has been proposed that chlorophyll preceded bacteriochlorophyll in its evolution. However, recent analyses of nucleotide sequences that encode chlorophyll and bacteriochlorophyll biosynthetic enzymes appear to provide support for an alternative hypothesis. This is that the evolution of bacteriochlorophyll occurred earlier than the evolution of chlorophyll. Here we demonstrate that the presence of invariant sites in sequence datasets leads to inconsistency in tree building (including maximum-likelihood methods). Homologous sequences with different biological functions often share invariant sites at the same nucleotide positions. However, different constraints can also result in additional invariant sites unique to the genes, which have specific and different biological functions. Consequently, the distribution of these sites can be uneven between the different types of homologous genes. The presence of invariant sites, shared by related biosynthetic genes as well as those unique to only some of these genes, has misled the recent evolutionary analysis of oxygenic and anoxygenic photosynthetic pigments. We evaluate an alternative scheme for the evolution of chlorophyll and bacteriochlorophyll.
Full text
PDF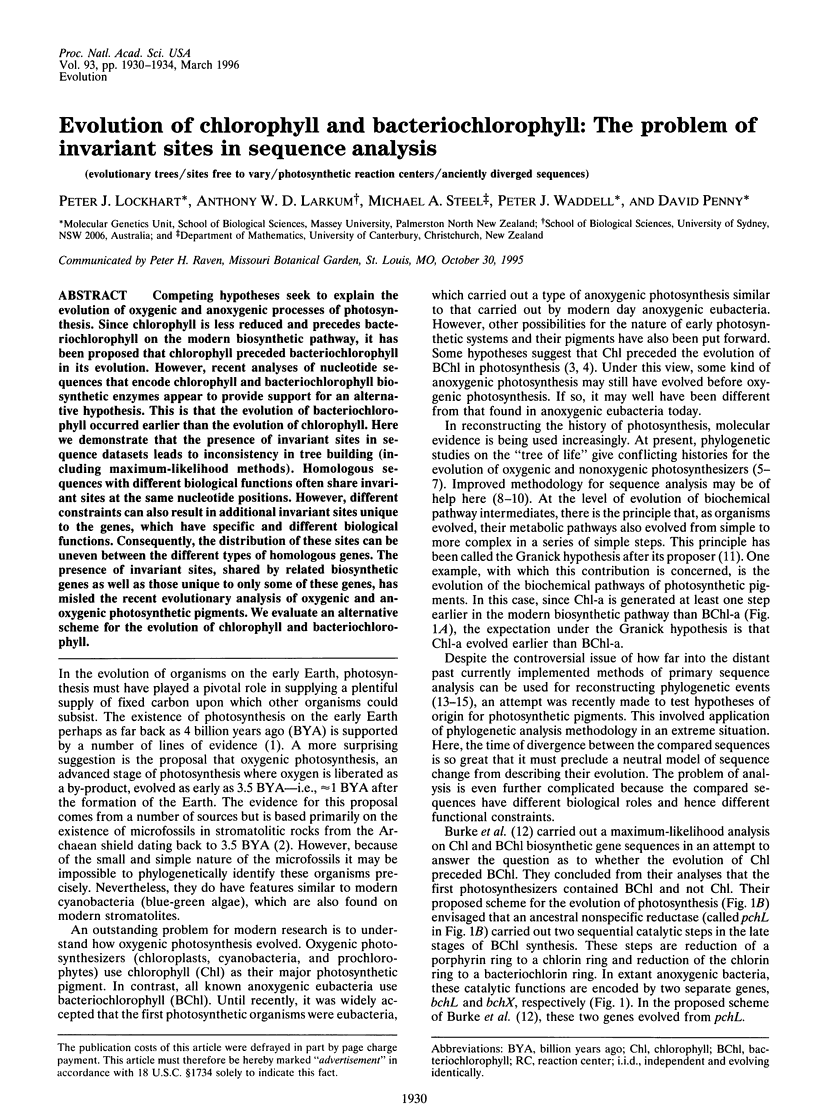
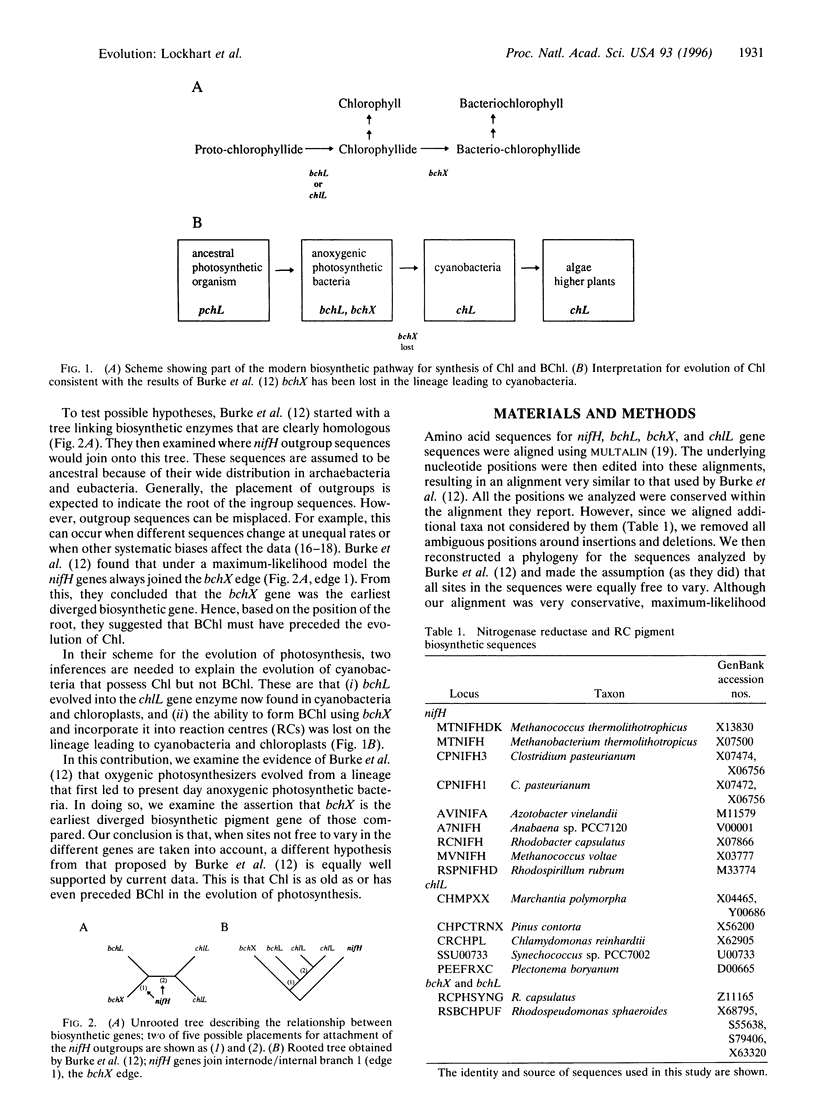
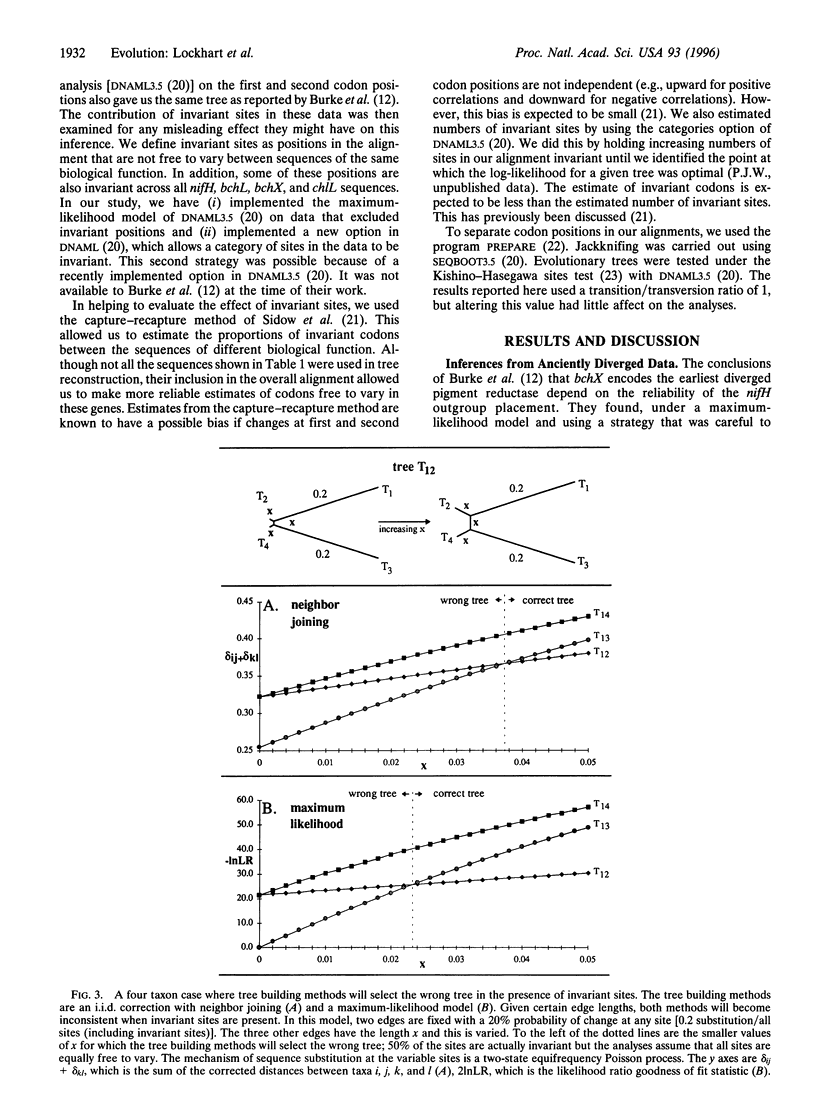
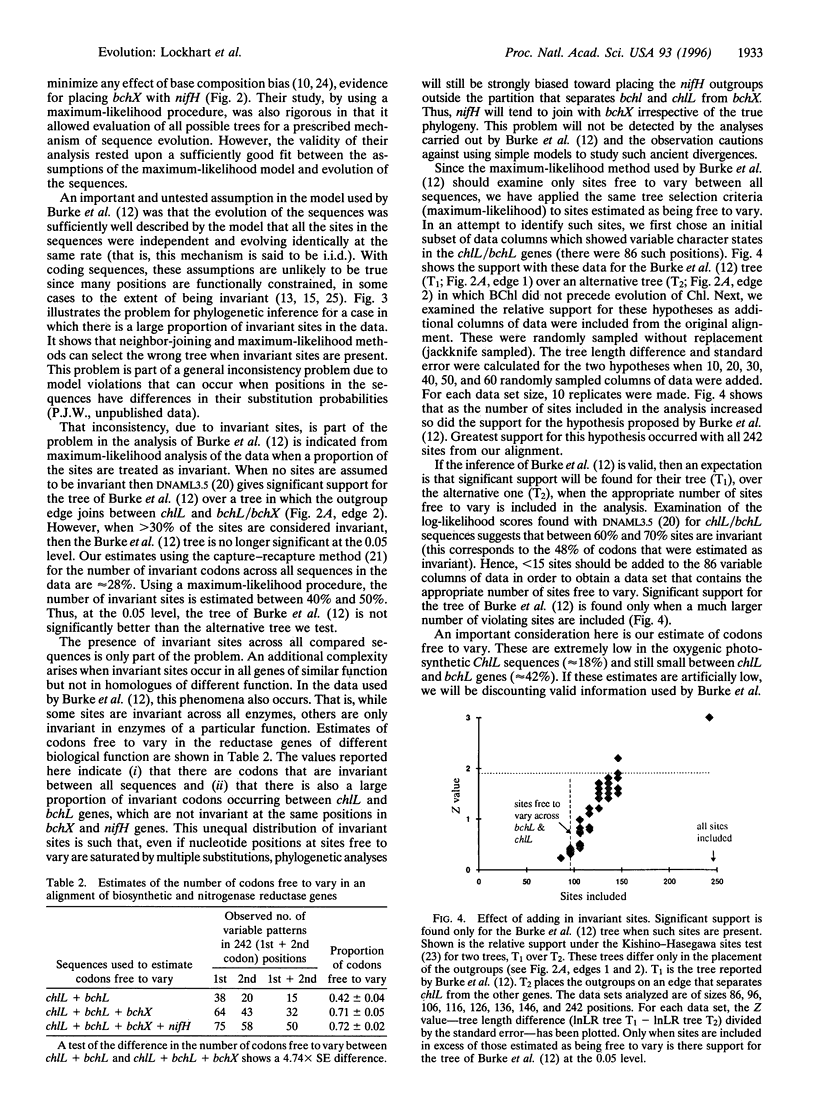
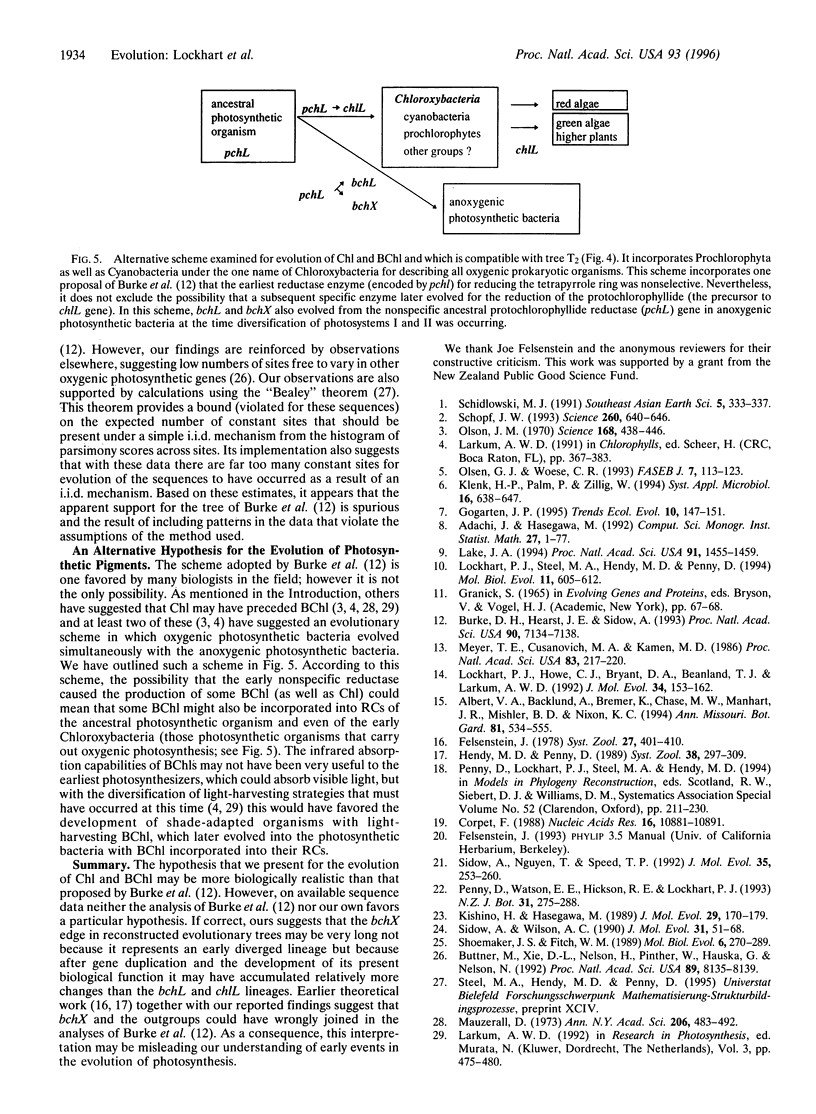
Selected References
These references are in PubMed. This may not be the complete list of references from this article.
- Burke D. H., Hearst J. E., Sidow A. Early evolution of photosynthesis: clues from nitrogenase and chlorophyll iron proteins. Proc Natl Acad Sci U S A. 1993 Aug 1;90(15):7134–7138. doi: 10.1073/pnas.90.15.7134. [DOI] [PMC free article] [PubMed] [Google Scholar]
- Büttner M., Xie D. L., Nelson H., Pinther W., Hauska G., Nelson N. Photosynthetic reaction center genes in green sulfur bacteria and in photosystem 1 are related. Proc Natl Acad Sci U S A. 1992 Sep 1;89(17):8135–8139. doi: 10.1073/pnas.89.17.8135. [DOI] [PMC free article] [PubMed] [Google Scholar]
- Corpet F. Multiple sequence alignment with hierarchical clustering. Nucleic Acids Res. 1988 Nov 25;16(22):10881–10890. doi: 10.1093/nar/16.22.10881. [DOI] [PMC free article] [PubMed] [Google Scholar]
- Kishino H., Hasegawa M. Evaluation of the maximum likelihood estimate of the evolutionary tree topologies from DNA sequence data, and the branching order in hominoidea. J Mol Evol. 1989 Aug;29(2):170–179. doi: 10.1007/BF02100115. [DOI] [PubMed] [Google Scholar]
- Lake J. A. Reconstructing evolutionary trees from DNA and protein sequences: paralinear distances. Proc Natl Acad Sci U S A. 1994 Feb 15;91(4):1455–1459. doi: 10.1073/pnas.91.4.1455. [DOI] [PMC free article] [PubMed] [Google Scholar]
- Lockhart P. J., Howe C. J., Bryant D. A., Beanland T. J., Larkum A. W. Substitutional bias confounds inference of cyanelle origins from sequence data. J Mol Evol. 1992 Feb;34(2):153–162. doi: 10.1007/BF00182392. [DOI] [PubMed] [Google Scholar]
- Mauzerall D. Why chlorophyll? Ann N Y Acad Sci. 1973;206:483–494. doi: 10.1111/j.1749-6632.1973.tb43231.x. [DOI] [PubMed] [Google Scholar]
- Meyer T. E., Cusanovich M. A., Kamen M. D. Evidence against use of bacterial amino acid sequence data for construction of all-inclusive phylogenetic trees. Proc Natl Acad Sci U S A. 1986 Jan;83(2):217–220. doi: 10.1073/pnas.83.2.217. [DOI] [PMC free article] [PubMed] [Google Scholar]
- Olsen G. J., Woese C. R. Ribosomal RNA: a key to phylogeny. FASEB J. 1993 Jan;7(1):113–123. doi: 10.1096/fasebj.7.1.8422957. [DOI] [PubMed] [Google Scholar]
- Olson J. M. The evolution of photosynthesis. Science. 1970 Apr 24;168(3930):438–446. doi: 10.1126/science.168.3930.438. [DOI] [PubMed] [Google Scholar]
- Schopf J. W. Microfossils of the Early Archean Apex chert: new evidence of the antiquity of life. Science. 1993 Apr 30;260:640–646. doi: 10.1126/science.260.5108.640. [DOI] [PubMed] [Google Scholar]
- Shoemaker J. S., Fitch W. M. Evidence from nuclear sequences that invariable sites should be considered when sequence divergence is calculated. Mol Biol Evol. 1989 May;6(3):270–289. doi: 10.1093/oxfordjournals.molbev.a040550. [DOI] [PubMed] [Google Scholar]
- Sidow A., Nguyen T., Speed T. P. Estimating the fraction of invariable codons with a capture-recapture method. J Mol Evol. 1992 Sep;35(3):253–260. doi: 10.1007/BF00178601. [DOI] [PubMed] [Google Scholar]
- Sidow A., Wilson A. C. Compositional statistics: an improvement of evolutionary parsimony and its application to deep branches in the tree of life. J Mol Evol. 1990 Jul;31(1):51–68. doi: 10.1007/BF02101792. [DOI] [PubMed] [Google Scholar]