Abstract
Pumpkin leaves grown under high light (500-700 micromol of photons m-2.s-1) were illuminated under photon flux densities ranging from 6.5 to 1500 micromol.m-2.s-1 in the presence of lincomycin, an inhibitor of chloroplast protein synthesis. The illumination at all light intensities caused photoinhibition, measured as a decrease in the ratio of variable to maximum fluorescence. Loss of photosystem II (PSII) electron transfer activity correlated with the decrease in the fluorescence ratio. The rate constant of photoinhibition, determined from first-order fits, was directly proportional to photon flux density at all light intensities studied. The fluorescence ratio did not decrease if the leaves were illuminated in low light in the absence of lincomycin or incubated in darkness in the presence of lincomycin. The constancy of the quantum yield of photoinhibition under different photon flux densities strongly suggests that photoinhibition in vivo occurs by one dominant mechanism under all light intensities. This mechanism probably is not the acceptor side mechanism characterized in the anaerobic case in vitro. Furthermore, there was an excellent correlation between the loss of PSII activity and the loss of the D1 protein from thylakoid membranes under low light. At low light, photoinhibition occurs so slowly that inactive PSII centers with the D1 protein waiting to be degraded do not accumulate. The kinetic agreement between D1 protein degradation and the inactivation of PSII indicates that the turnover of the D1 protein depends on photoinhibition under both low and high light.
Full text
PDF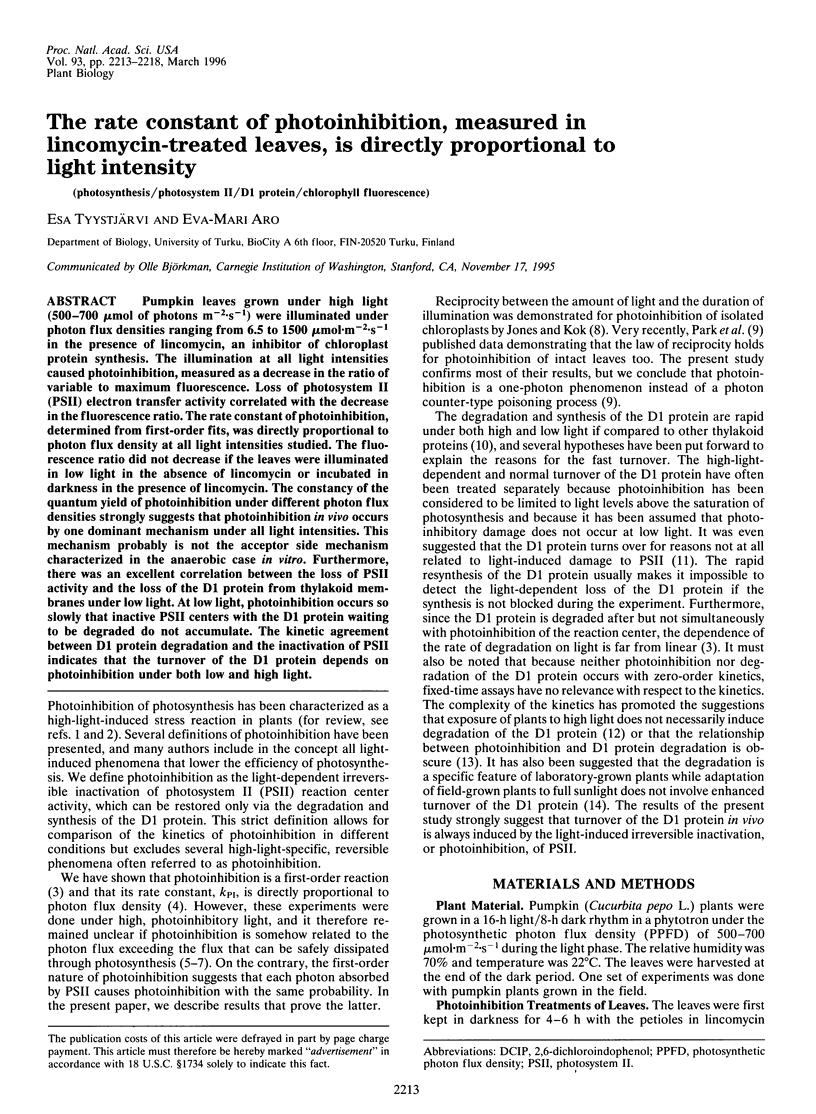
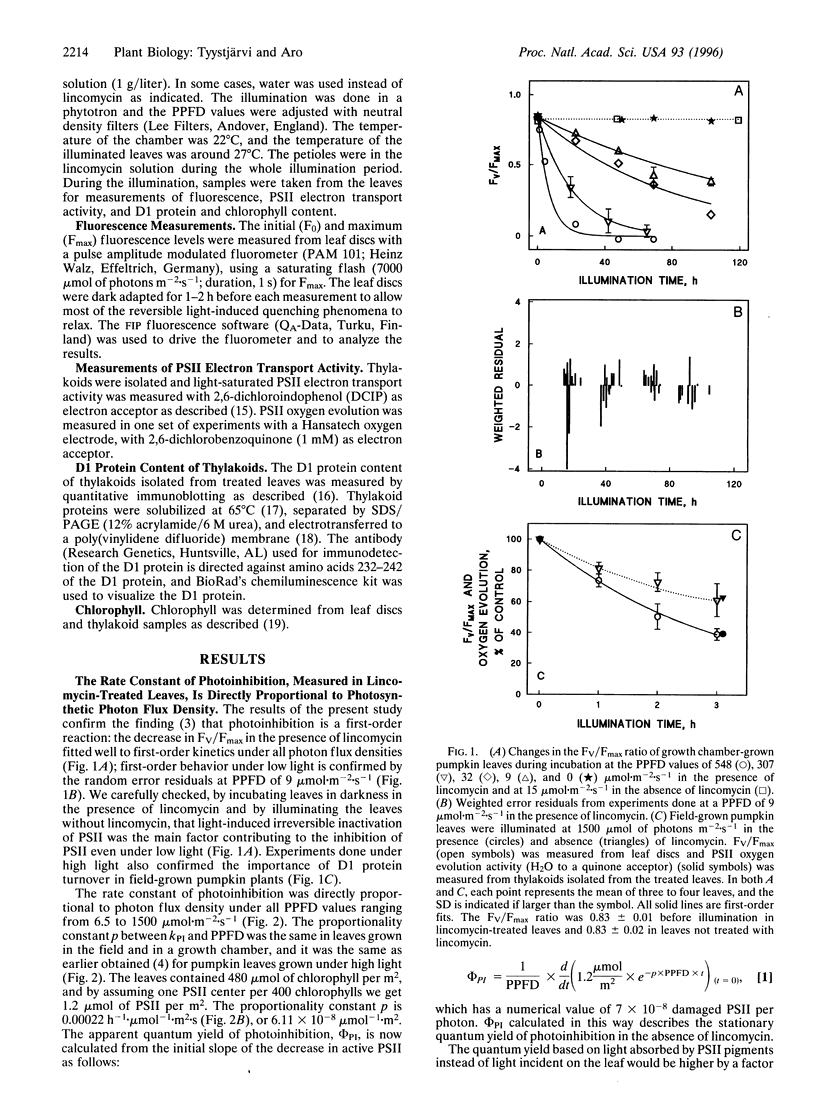
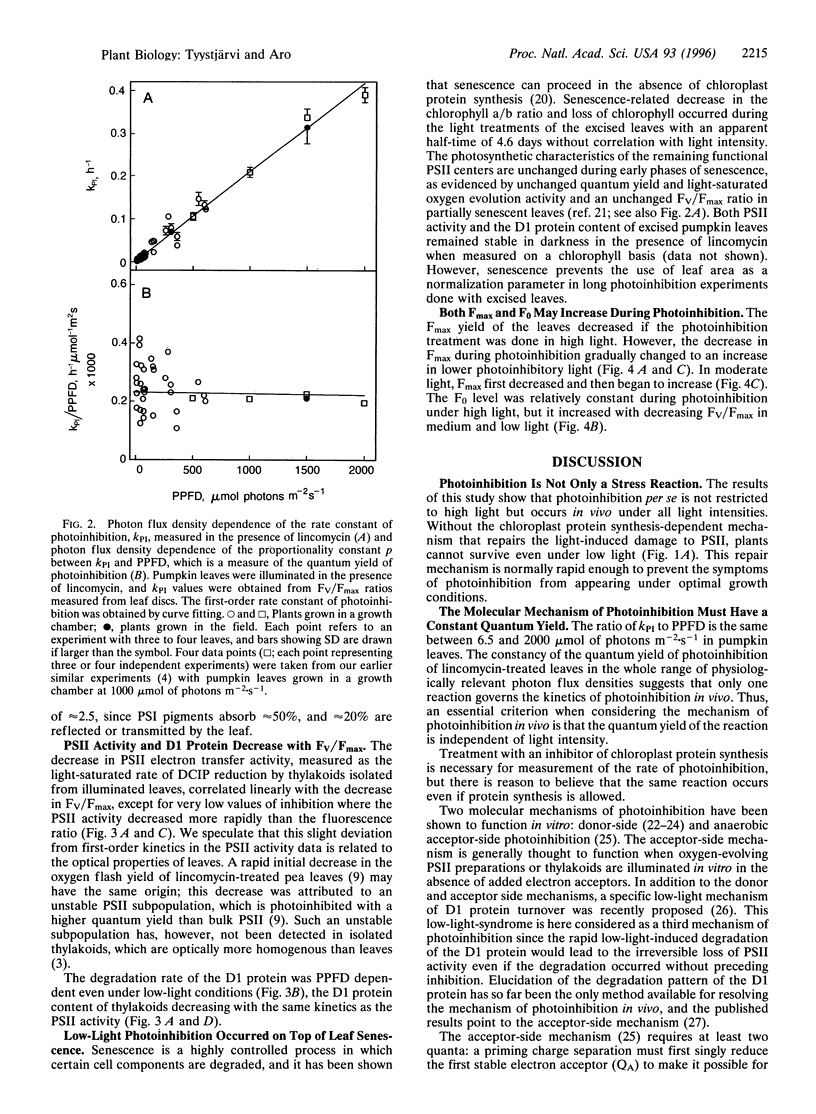
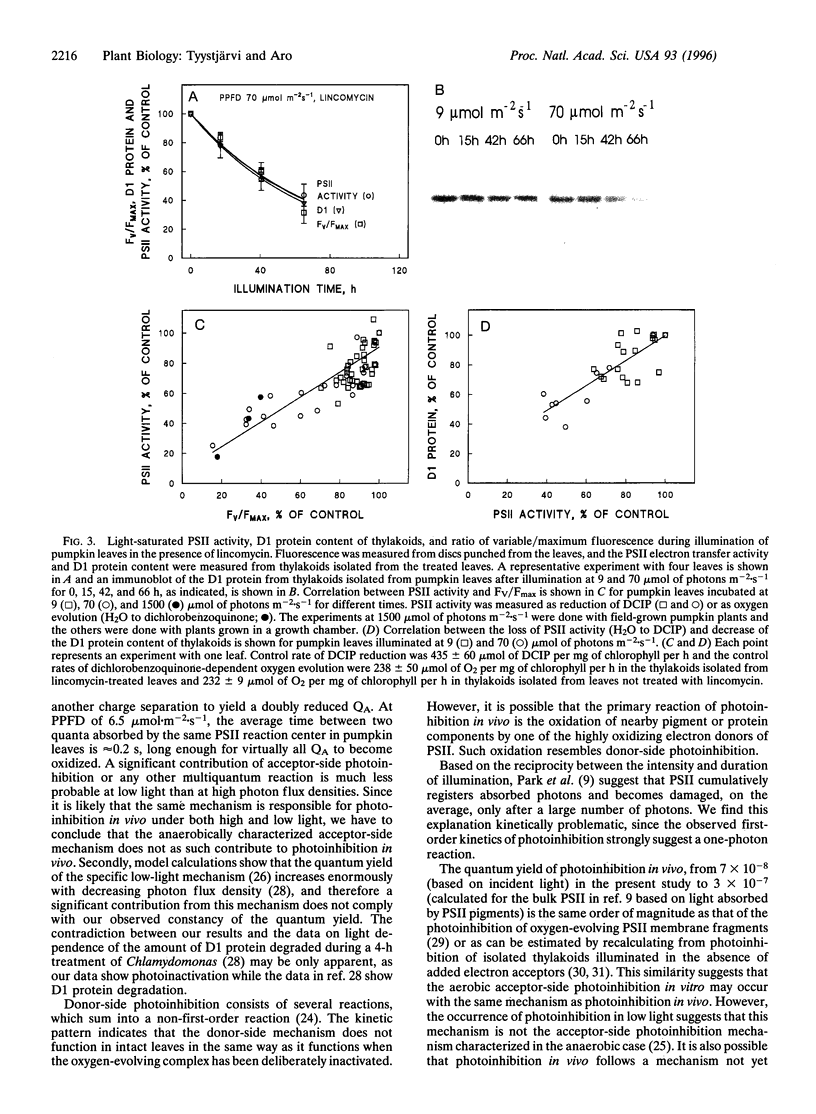
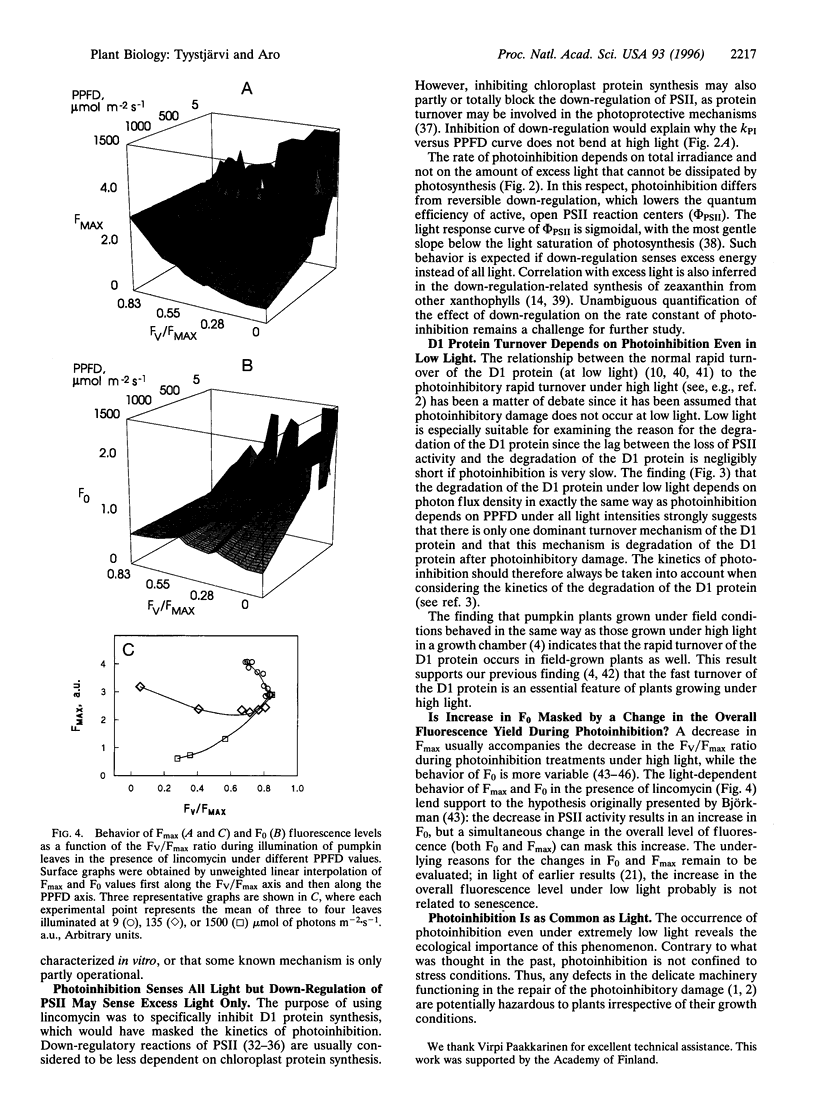
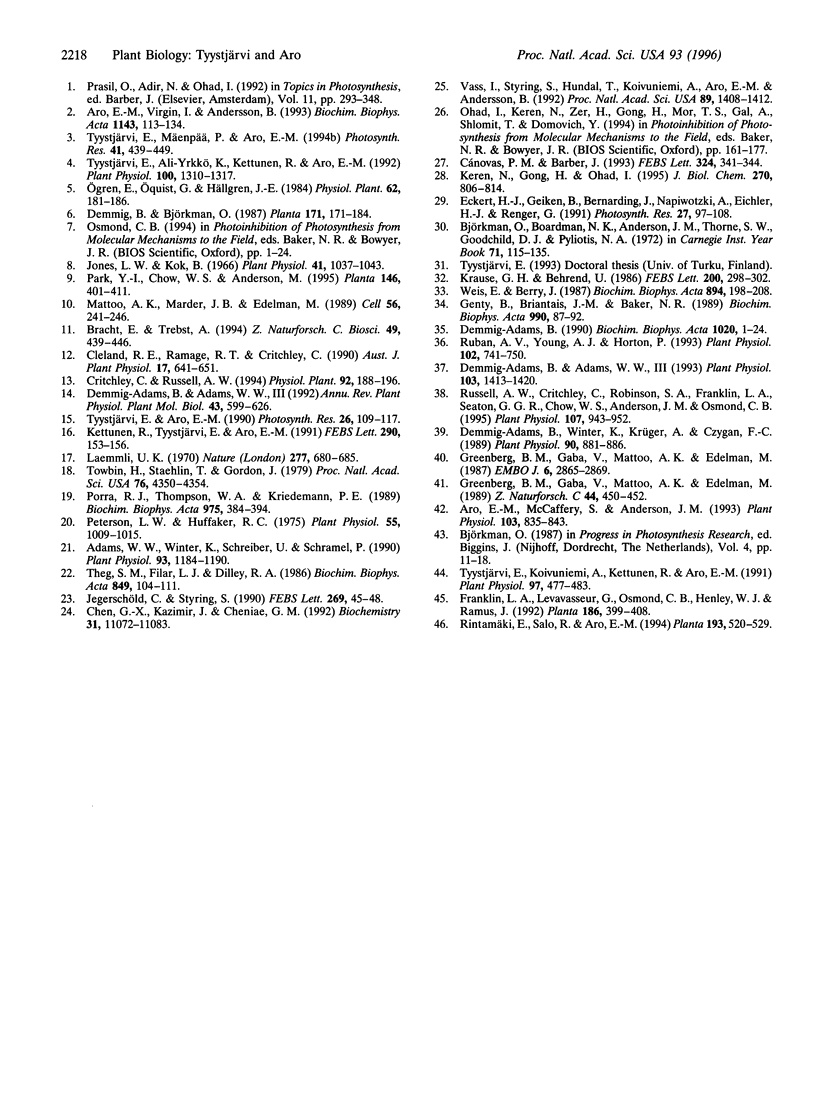
Images in this article
Selected References
These references are in PubMed. This may not be the complete list of references from this article.
- Adams W. W., Winter K., Schreiber U., Schramel P. Photosynthesis and Chlorophyll Fluorescence Characteristics in Relationship to Changes in Pigment and Element Composition of Leaves of Platanus occidentalis L. during Autumnal Leaf Senescence. Plant Physiol. 1990 Apr;92(4):1184–1190. doi: 10.1104/pp.92.4.1184. [DOI] [PMC free article] [PubMed] [Google Scholar]
- Aro E. M., McCaffery S., Anderson J. M. Photoinhibition and D1 Protein Degradation in Peas Acclimated to Different Growth Irradiances. Plant Physiol. 1993 Nov;103(3):835–843. doi: 10.1104/pp.103.3.835. [DOI] [PMC free article] [PubMed] [Google Scholar]
- Aro E. M., Virgin I., Andersson B. Photoinhibition of Photosystem II. Inactivation, protein damage and turnover. Biochim Biophys Acta. 1993 Jul 5;1143(2):113–134. doi: 10.1016/0005-2728(93)90134-2. [DOI] [PubMed] [Google Scholar]
- Chen G. X., Kazimir J., Cheniae G. M. Photoinhibition of hydroxylamine-extracted photosystem II membranes: studies of the mechanism. Biochemistry. 1992 Nov 17;31(45):11072–11083. doi: 10.1021/bi00160a017. [DOI] [PubMed] [Google Scholar]
- Cánovas P. M., Barber J. Detection of a 10 kDa breakdown product containing the C-terminus of the D1-protein in photoinhibited wheat leaves suggests an acceptor side mechanism. FEBS Lett. 1993 Jun 21;324(3):341–344. doi: 10.1016/0014-5793(93)80147-m. [DOI] [PubMed] [Google Scholar]
- Demmig-Adams B., Adams W. W., 3rd The Xanthophyll Cycle, Protein Turnover, and the High Light Tolerance of Sun-Acclimated Leaves. Plant Physiol. 1993 Dec;103(4):1413–1420. doi: 10.1104/pp.103.4.1413. [DOI] [PMC free article] [PubMed] [Google Scholar]
- Demmig-Adams B., Winter K., Krüger A., Czygan F. C. Light Response of CO(2) Assimilation, Dissipation of Excess Excitation Energy, and Zeaxanthin Content of Sun and Shade Leaves. Plant Physiol. 1989 Jul;90(3):881–886. doi: 10.1104/pp.90.3.881. [DOI] [PMC free article] [PubMed] [Google Scholar]
- Greenberg B. M., Gaba V., Mattoo A. K., Edelman M. Identification of a primary in vivo degradation product of the rapidly-turning-over 32 kd protein of photosystem II. EMBO J. 1987 Oct;6(10):2865–2869. doi: 10.1002/j.1460-2075.1987.tb02588.x. [DOI] [PMC free article] [PubMed] [Google Scholar]
- Jones L. W., Kok B. Photoinhibition of chloroplast reactions. I. Kinetics and action spectra. Plant Physiol. 1966 Jun;41(6):1037–1043. doi: 10.1104/pp.41.6.1037. [DOI] [PMC free article] [PubMed] [Google Scholar]
- Keren N., Gong H., Ohad I. Oscillations of reaction center II-D1 protein degradation in vivo induced by repetitive light flashes. Correlation between the level of RCII-QB- and protein degradation in low light. J Biol Chem. 1995 Jan 13;270(2):806–814. doi: 10.1074/jbc.270.2.806. [DOI] [PubMed] [Google Scholar]
- Kettunen R., Tyystjärvi E., Aro E. M. D1 protein degradation during photoinhibition of intact leaves. A modification of the D1 protein precedes degradation. FEBS Lett. 1991 Sep 23;290(1-2):153–156. doi: 10.1016/0014-5793(91)81247-6. [DOI] [PubMed] [Google Scholar]
- Laemmli U. K. Cleavage of structural proteins during the assembly of the head of bacteriophage T4. Nature. 1970 Aug 15;227(5259):680–685. doi: 10.1038/227680a0. [DOI] [PubMed] [Google Scholar]
- Mattoo A. K., Marder J. B., Edelman M. Dynamics of the photosystem II reaction center. Cell. 1989 Jan 27;56(2):241–246. doi: 10.1016/0092-8674(89)90897-0. [DOI] [PubMed] [Google Scholar]
- Peterson L. W., Huffaker R. C. Loss of Ribulose 1,5-Diphosphate Carboxylase and Increase in Proteolytic Activity during Senescence of Detached Primary Barley Leaves. Plant Physiol. 1975 Jun;55(6):1009–1015. doi: 10.1104/pp.55.6.1009. [DOI] [PMC free article] [PubMed] [Google Scholar]
- Ruban A. V., Young A. J., Horton P. Induction of Nonphotochemical Energy Dissipation and Absorbance Changes in Leaves (Evidence for Changes in the State of the Light-Harvesting System of Photosystem II in Vivo). Plant Physiol. 1993 Jul;102(3):741–750. doi: 10.1104/pp.102.3.741. [DOI] [PMC free article] [PubMed] [Google Scholar]
- Russell A. W., Critchley C., Robinson S. A., Franklin L. A., Seaton GGR., Chow W. S., Anderson J. M., Osmond C. B. Photosystem II Regulation and Dynamics of the Chloroplast D1 Protein in Arabidopsis Leaves during Photosynthesis and Photoinhibition. Plant Physiol. 1995 Mar;107(3):943–952. doi: 10.1104/pp.107.3.943. [DOI] [PMC free article] [PubMed] [Google Scholar]
- Towbin H., Staehelin T., Gordon J. Electrophoretic transfer of proteins from polyacrylamide gels to nitrocellulose sheets: procedure and some applications. Proc Natl Acad Sci U S A. 1979 Sep;76(9):4350–4354. doi: 10.1073/pnas.76.9.4350. [DOI] [PMC free article] [PubMed] [Google Scholar]
- Tyystjärvi E., Ali-Yrkkö K., Kettunen R., Aro E. M. Slow degradation of the d1 protein is related to the susceptibility of low-light-grown pumpkin plants to photoinhibition. Plant Physiol. 1992 Nov;100(3):1310–1317. doi: 10.1104/pp.100.3.1310. [DOI] [PMC free article] [PubMed] [Google Scholar]
- Tyystjärvi E., Koivuniemi A., Kettunen R., Aro E. M. Small light-harvesting antenna does not protect from photoinhibition. Plant Physiol. 1991 Oct;97(2):477–483. doi: 10.1104/pp.97.2.477. [DOI] [PMC free article] [PubMed] [Google Scholar]
- Vass I., Styring S., Hundal T., Koivuniemi A., Aro E., Andersson B. Reversible and irreversible intermediates during photoinhibition of photosystem II: stable reduced QA species promote chlorophyll triplet formation. Proc Natl Acad Sci U S A. 1992 Feb 15;89(4):1408–1412. doi: 10.1073/pnas.89.4.1408. [DOI] [PMC free article] [PubMed] [Google Scholar]