Abstract
Of all humans thus far studied, Sherpas are considered by many high-altitude biomedical scientists as most exquisitely adapted for life under continuous hypobaric hypoxia. However, little is known about how the heart is protected in hypoxia. Hypoxia defense mechanisms in the Sherpa heart were explored by in vivo, noninvasive 31P magnetic resonance spectroscopy. Six Sherpas were examined under two experimental conditions [normoxic (21% FiO2) and hypoxic (11% FiO2) and in two adaptational states--the acclimated state (on arrival at low-altitude study sites) and the deacclimating state (4 weeks of ongoing exposure to low altitude). Four lowland subjects were used for comparison. We found that the concentration ratios of phosphocreatine (PCr)/adenosine triphosphate (ATP) were maintained at steady-state normoxic values (0.96, SEM = 0.22) that were about half those found in normoxic lowlanders (1.76, SEM = 0.03) monitored the same way at the same time. These differences in heart energetic status between Sherpas and lowlanders compared under normoxic conditions remained highly significant (P < 0.02) even after 4 weeks of deacclimation at low altitudes. In Sherpas under acute hypoxia, the heart rate increased by 20 beats per min from resting values of about 70 beats per min, and the percent saturation of hemoglobin decreased to about 75%. However, these perturbations did not alter the PCr/ATP concentration ratios, which remained at about 50% of the values expected in healthy lowlanders. Because the creatine phosphokinase reaction functions close to equilibrium, these steady-state PCr/ATP ratios presumably coincided with about 3-fold higher free adenosine diphosphate (ADP) concentrations. Higher ADP concentrations (i.e., lower [PCr]/[ATP] ratios) were interpreted to correlate with the Km values for ADP-requiring kinases of glycolysis and to reflect elevated carbohydrate contributions to heart energy needs. This metabolic organization is postulated as advantageous in hypobaria because the ATP yield per O2 molecule is 25-60% higher with glucose than with free fatty acids (the usual fuels utilized in the human heart in postfasting conditions).
Full text
PDF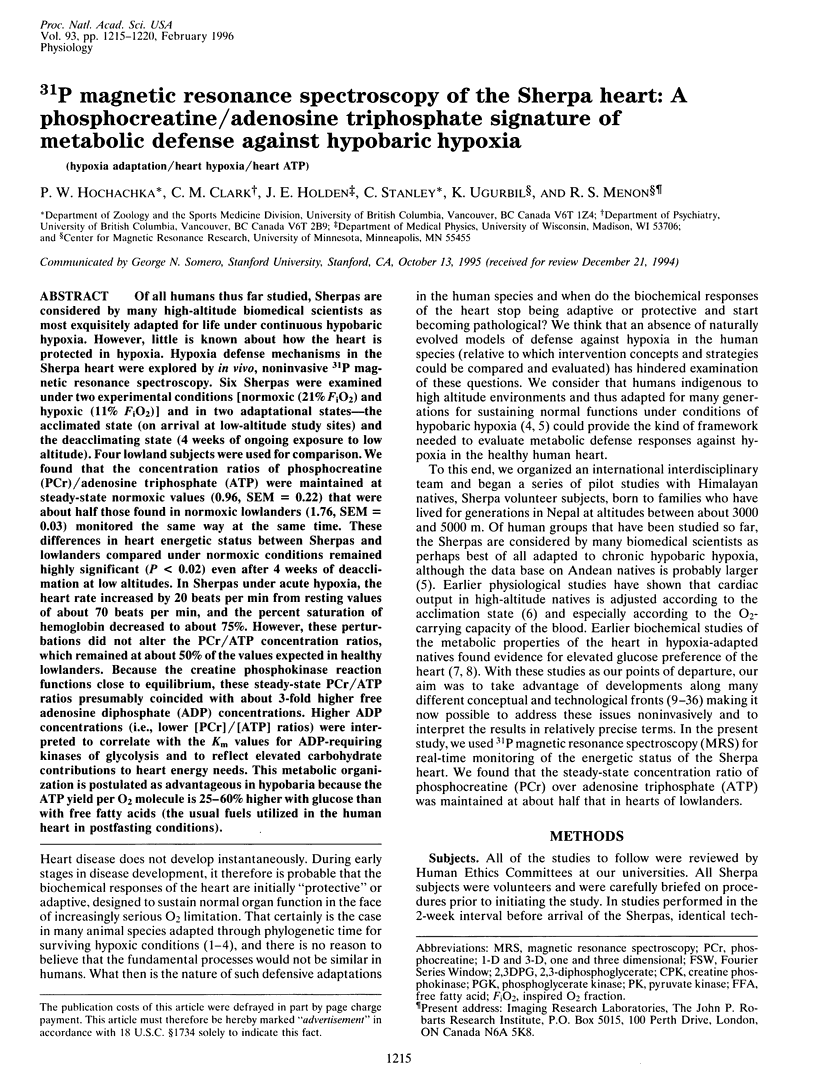
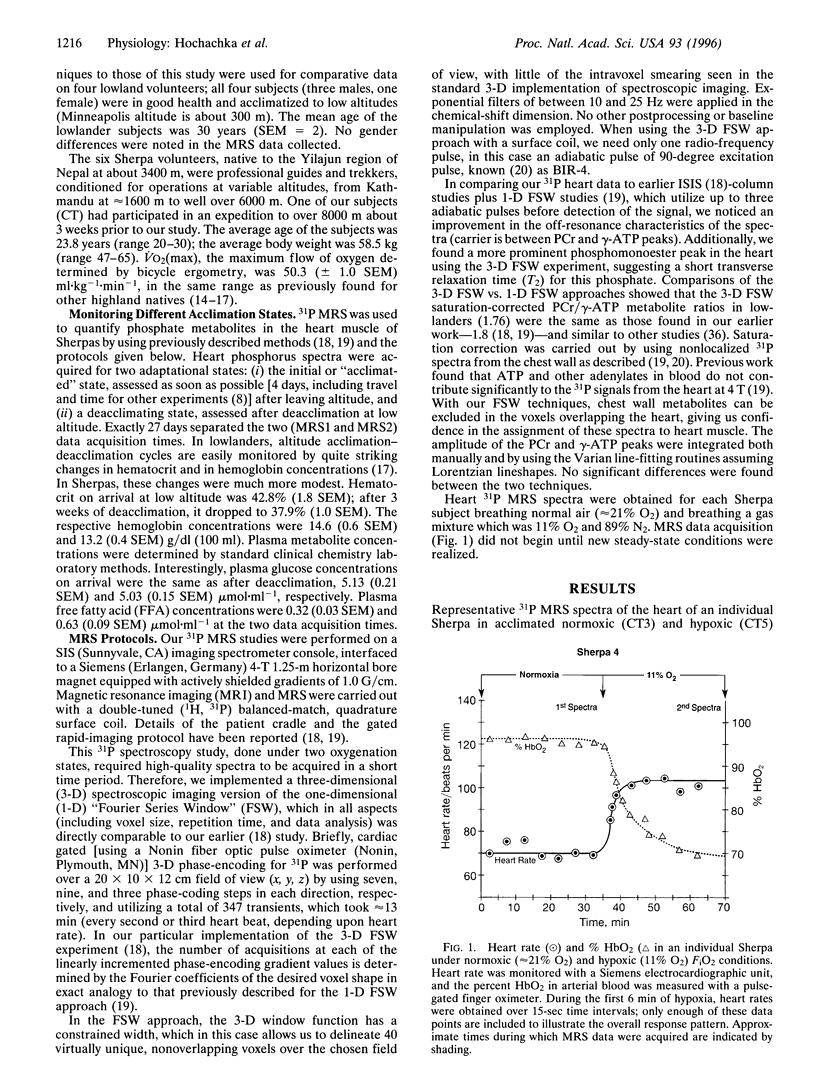
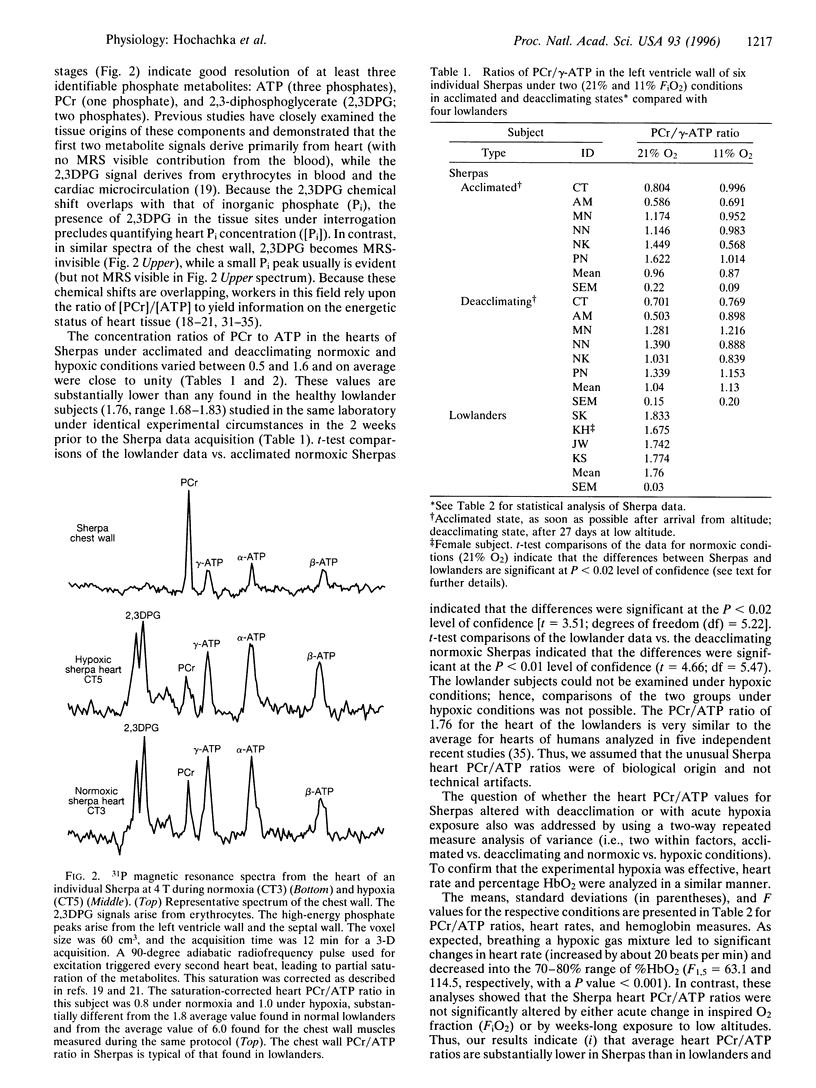
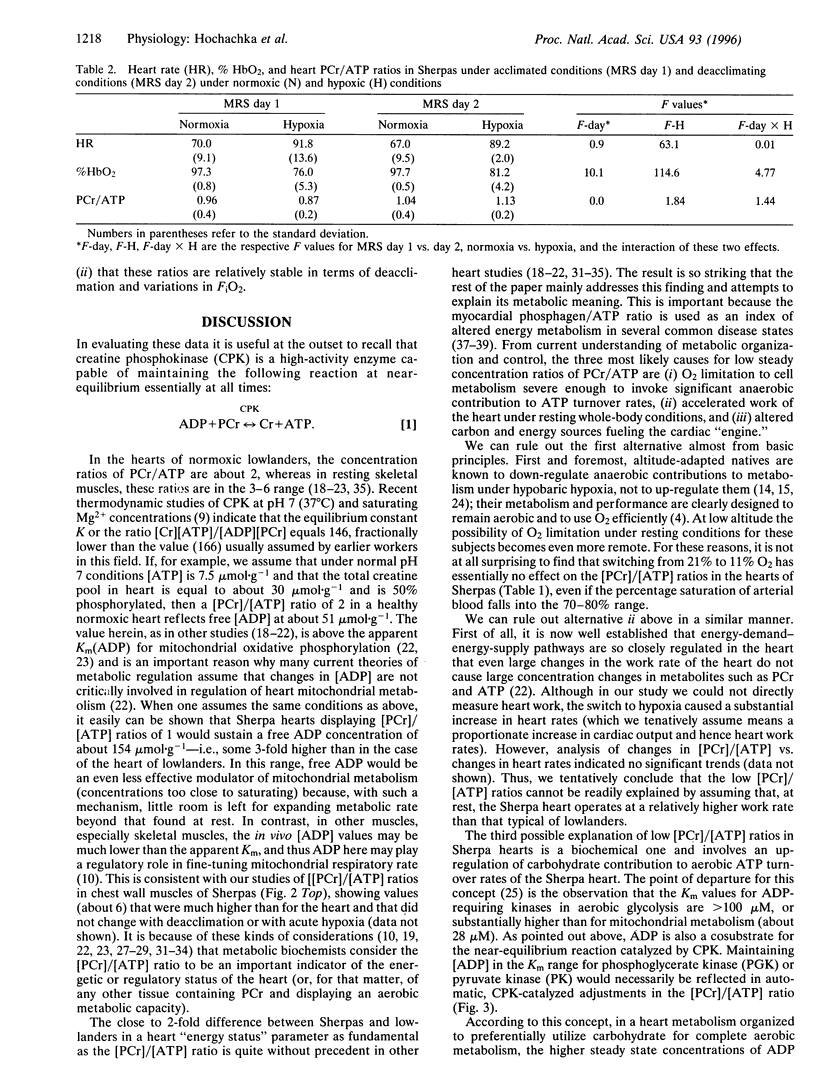
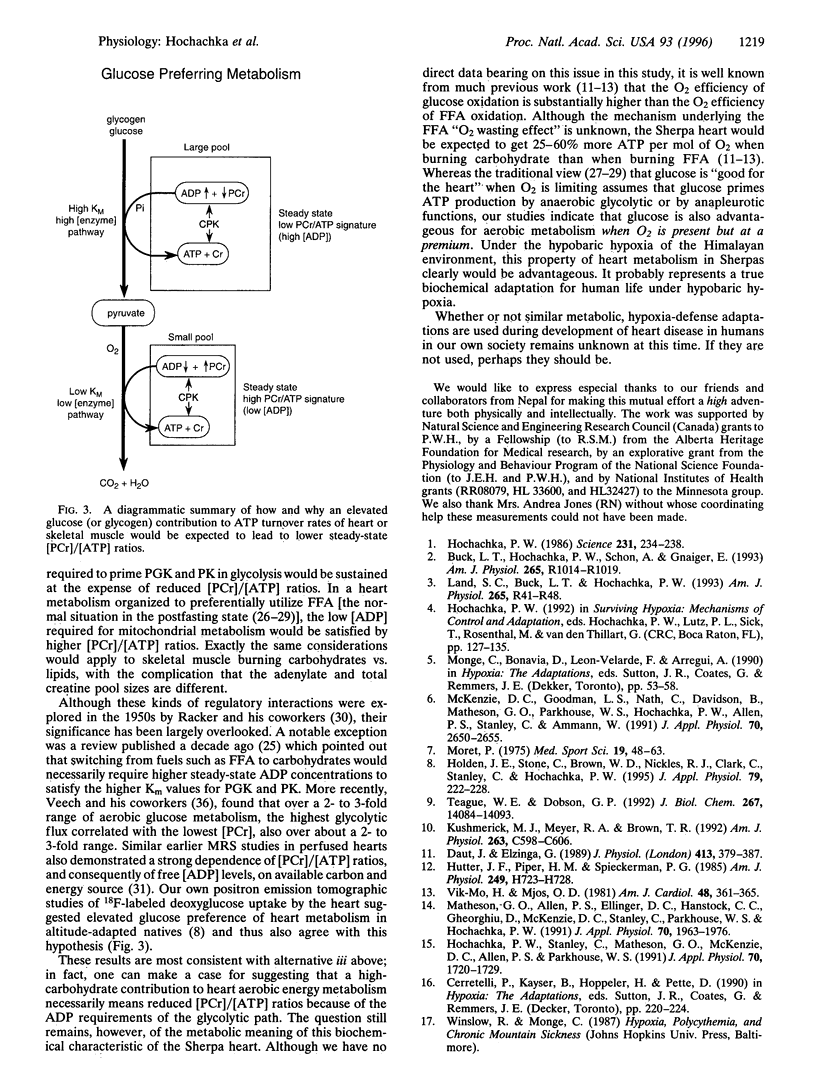
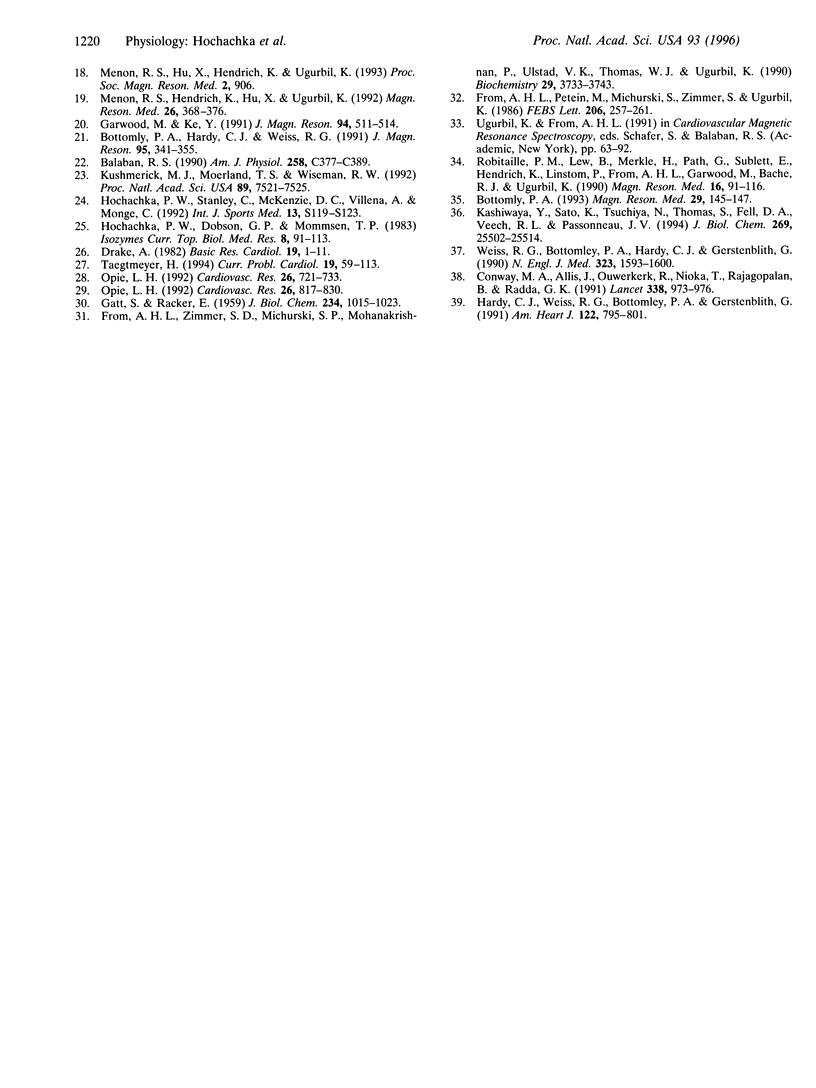
Images in this article
Selected References
These references are in PubMed. This may not be the complete list of references from this article.
- Balaban R. S. Regulation of oxidative phosphorylation in the mammalian cell. Am J Physiol. 1990 Mar;258(3 Pt 1):C377–C389. doi: 10.1152/ajpcell.1990.258.3.C377. [DOI] [PubMed] [Google Scholar]
- Bottomley P. A. The true T1 values of myocardial high-energy phosphates? Magn Reson Med. 1993 Jan;29(1):145–147. doi: 10.1002/mrm.1910290127. [DOI] [PubMed] [Google Scholar]
- Buck L. T., Hochachka P. W., Schön A., Gnaiger E. Microcalorimetric measurement of reversible metabolic suppression induced by anoxia in isolated hepatocytes. Am J Physiol. 1993 Nov;265(5 Pt 2):R1014–R1019. doi: 10.1152/ajpregu.1993.265.5.R1014. [DOI] [PubMed] [Google Scholar]
- Conway M. A., Allis J., Ouwerkerk R., Niioka T., Rajagopalan B., Radda G. K. Detection of low phosphocreatine to ATP ratio in failing hypertrophied human myocardium by 31P magnetic resonance spectroscopy. Lancet. 1991 Oct 19;338(8773):973–976. doi: 10.1016/0140-6736(91)91838-l. [DOI] [PubMed] [Google Scholar]
- Daut J., Elzinga G. Substrate dependence of energy metabolism in isolated guinea-pig cardiac muscle: a microcalorimetric study. J Physiol. 1989 Jun;413:379–397. doi: 10.1113/jphysiol.1989.sp017659. [DOI] [PMC free article] [PubMed] [Google Scholar]
- Drake A. J. Substrate utilization in the myocardium. Basic Res Cardiol. 1982 Jan-Feb;77(1):1–11. doi: 10.1007/BF01908126. [DOI] [PubMed] [Google Scholar]
- From A. H., Petein M. A., Michurski S. P., Zimmer S. D., Uğurbil K. 31P-NMR studies of respiratory regulation in the intact myocardium. FEBS Lett. 1986 Oct 6;206(2):257–261. doi: 10.1016/0014-5793(86)80992-9. [DOI] [PubMed] [Google Scholar]
- From A. H., Zimmer S. D., Michurski S. P., Mohanakrishnan P., Ulstad V. K., Thoma W. J., Uğurbil K. Regulation of the oxidative phosphorylation rate in the intact cell. Biochemistry. 1990 Apr 17;29(15):3731–3743. doi: 10.1021/bi00467a020. [DOI] [PubMed] [Google Scholar]
- GATT S., RACKER E. Regulatory mechanisms in carbohydrate metabolism. I. Crabtree effect in reconstructed systems. J Biol Chem. 1959 May;234(5):1015–1023. [PubMed] [Google Scholar]
- Hardy C. J., Weiss R. G., Bottomley P. A., Gerstenblith G. Altered myocardial high-energy phosphate metabolites in patients with dilated cardiomyopathy. Am Heart J. 1991 Sep;122(3 Pt 1):795–801. doi: 10.1016/0002-8703(91)90527-o. [DOI] [PubMed] [Google Scholar]
- Hochachka P. W. Defense strategies against hypoxia and hypothermia. Science. 1986 Jan 17;231(4735):234–241. doi: 10.1126/science.2417316. [DOI] [PubMed] [Google Scholar]
- Hochachka P. W., Dobson G. P., Mommsen T. P. Role of isozymes in metabolic regulation during exercise: insights from comparative studies. Isozymes Curr Top Biol Med Res. 1983;8:91–113. [PubMed] [Google Scholar]
- Hochachka P. W., Stanley C., Matheson G. O., McKenzie D. C., Allen P. S., Parkhouse W. S. Metabolic and work efficiencies during exercise in Andean natives. J Appl Physiol (1985) 1991 Apr;70(4):1720–1730. doi: 10.1152/jappl.1991.70.4.1720. [DOI] [PubMed] [Google Scholar]
- Hochachka P. W., Stanley C., McKenzie D. C., Villena A., Monge C. Enzyme mechanisms for pyruvate-to-lactate flux attenuation: a study of Sherpas, Quechuas, and hummingbirds. Int J Sports Med. 1992 Oct;13 (Suppl 1):S119–S122. doi: 10.1055/s-2007-1024613. [DOI] [PubMed] [Google Scholar]
- Holden J. E., Stone C. K., Clark C. M., Brown W. D., Nickles R. J., Stanley C., Hochachka P. W. Enhanced cardiac metabolism of plasma glucose in high-altitude natives: adaptation against chronic hypoxia. J Appl Physiol (1985) 1995 Jul;79(1):222–228. doi: 10.1152/jappl.1995.79.1.222. [DOI] [PubMed] [Google Scholar]
- Hütter J. F., Piper H. M., Spieckerman P. G. Effect of fatty acid oxidation on efficiency of energy production in rat heart. Am J Physiol. 1985 Oct;249(4 Pt 2):H723–H728. doi: 10.1152/ajpheart.1985.249.4.H723. [DOI] [PubMed] [Google Scholar]
- Kashiwaya Y., Sato K., Tsuchiya N., Thomas S., Fell D. A., Veech R. L., Passonneau J. V. Control of glucose utilization in working perfused rat heart. J Biol Chem. 1994 Oct 14;269(41):25502–25514. [PubMed] [Google Scholar]
- Kushmerick M. J., Meyer R. A., Brown T. R. Regulation of oxygen consumption in fast- and slow-twitch muscle. Am J Physiol. 1992 Sep;263(3 Pt 1):C598–C606. doi: 10.1152/ajpcell.1992.263.3.C598. [DOI] [PubMed] [Google Scholar]
- Kushmerick M. J., Moerland T. S., Wiseman R. W. Mammalian skeletal muscle fibers distinguished by contents of phosphocreatine, ATP, and Pi. Proc Natl Acad Sci U S A. 1992 Aug 15;89(16):7521–7525. doi: 10.1073/pnas.89.16.7521. [DOI] [PMC free article] [PubMed] [Google Scholar]
- Land S. C., Buck L. T., Hochachka P. W. Response of protein synthesis to anoxia and recovery in anoxia-tolerant hepatocytes. Am J Physiol. 1993 Jul;265(1 Pt 2):R41–R48. doi: 10.1152/ajpregu.1993.265.1.R41. [DOI] [PubMed] [Google Scholar]
- Matheson G. O., Allen P. S., Ellinger D. C., Hanstock C. C., Gheorghiu D., McKenzie D. C., Stanley C., Parkhouse W. S., Hochachka P. W. Skeletal muscle metabolism and work capacity: a 31P-NMR study of Andean natives and lowlanders. J Appl Physiol (1985) 1991 May;70(5):1963–1976. doi: 10.1152/jappl.1991.70.5.1963. [DOI] [PubMed] [Google Scholar]
- McKenzie D. C., Goodman L. S., Nath C., Davidson B., Matheson G. O., Parkhouse W. S., Hochachka P. W., Allen P. S., Stanley C., Ammann W. Cardiovascular adaptations in Andean natives after 6 wk of exposure to sea level. J Appl Physiol (1985) 1991 Jun;70(6):2650–2655. doi: 10.1152/jappl.1991.70.6.2650. [DOI] [PubMed] [Google Scholar]
- Menon R. S., Hendrich K., Hu X., Uğurbil K. 31P NMR spectroscopy of the human heart at 4 T: detection of substantially uncontaminated cardiac spectra and differentiation of subepicardium and subendocardium. Magn Reson Med. 1992 Aug;26(2):368–376. doi: 10.1002/mrm.1910260216. [DOI] [PubMed] [Google Scholar]
- Opie L. H. Cardiac metabolism--emergence, decline, and resurgence. Part I. Cardiovasc Res. 1992 Aug;26(8):721–733. doi: 10.1093/cvr/26.8.721. [DOI] [PubMed] [Google Scholar]
- Opie L. H. Cardiac metabolism--emergence, decline, and resurgence. Part II. Cardiovasc Res. 1992 Sep;26(9):817–830. doi: 10.1093/cvr/26.9.817. [DOI] [PubMed] [Google Scholar]
- Robitaille P. M., Merkle H., Lew B., Path G., Hendrich K., Lindstrom P., From A. H., Garwood M., Bache R. J., Uğurbil K. Transmural high energy phosphate distribution and response to alterations in workload in the normal canine myocardium as studied with spatially localized 31P NMR spectroscopy. Magn Reson Med. 1990 Oct;16(1):91–116. doi: 10.1002/mrm.1910160110. [DOI] [PubMed] [Google Scholar]
- Taegtmeyer H. Energy metabolism of the heart: from basic concepts to clinical applications. Curr Probl Cardiol. 1994 Feb;19(2):59–113. doi: 10.1016/0146-2806(94)90008-6. [DOI] [PubMed] [Google Scholar]
- Teague W. E., Jr, Dobson G. P. Effect of temperature on the creatine kinase equilibrium. J Biol Chem. 1992 Jul 15;267(20):14084–14093. [PubMed] [Google Scholar]
- Vik-Mo H., Mjøs O. D. Influence of free fatty acids on myocardial oxygen consumption and ischemic injury. Am J Cardiol. 1981 Aug;48(2):361–365. doi: 10.1016/0002-9149(81)90621-4. [DOI] [PubMed] [Google Scholar]
- Weiss R. G., Bottomley P. A., Hardy C. J., Gerstenblith G. Regional myocardial metabolism of high-energy phosphates during isometric exercise in patients with coronary artery disease. N Engl J Med. 1990 Dec 6;323(23):1593–1600. doi: 10.1056/NEJM199012063232304. [DOI] [PubMed] [Google Scholar]