Abstract
Recent studies have elucidated how the absorption of a photon in a rod or cone cell leads to the generation of the amplified neural signal that is transmitted to higher-order visual neurons. Photoexcited visual pigment activates the GTP-binding protein transducin, which in turn stimulates cGMP phosphodiesterase. This enzyme hydrolyzes cGMP, allowing cGMP-gated cationic channels in the surface membrane to close, hyperpolarize the cell, and modulate transmitter release at the synaptic terminal. The kinetics of reactions in the cGMP cascade limit the temporal resolution of the visual system as a whole, while statistical fluctuations in the reactions limit the reliability of detection of dim light. Much interest now focuses on the processes that terminate the light response and dynamically regulate amplification in the cascade, causing the single photon response to be reproducible and allowing the cell to adapt in background light. A light-induced fall in the internal free Ca2+ concentration coordinates negative feedback control of amplification. The fall in Ca2+ stimulates resynthesis of cGMP, antagonizes rhodopsin's catalytic activity, and increases the affinity of the light-regulated cationic channel for cGMP. We are using physiological methods to study the molecular mechanisms that terminate the flash response and mediate adaptation. One approach is to observe transduction in truncated, dialyzed photoreceptor cells whose internal Ca2+ and nucleotide concentrations are under experimental control and to which exogenous proteins can be added. Another approach is to observe transduction in transgenic mouse rods in which specific proteins within the cascade are altered or deleted.
Full text
PDF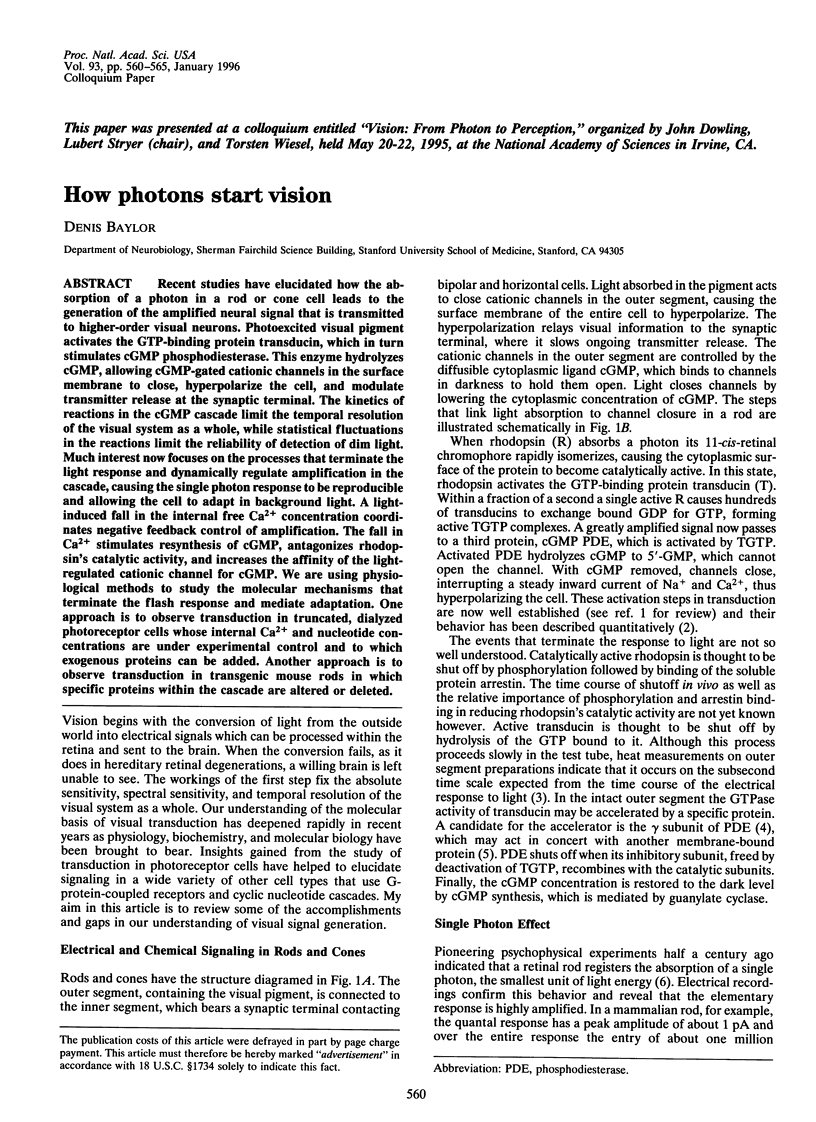
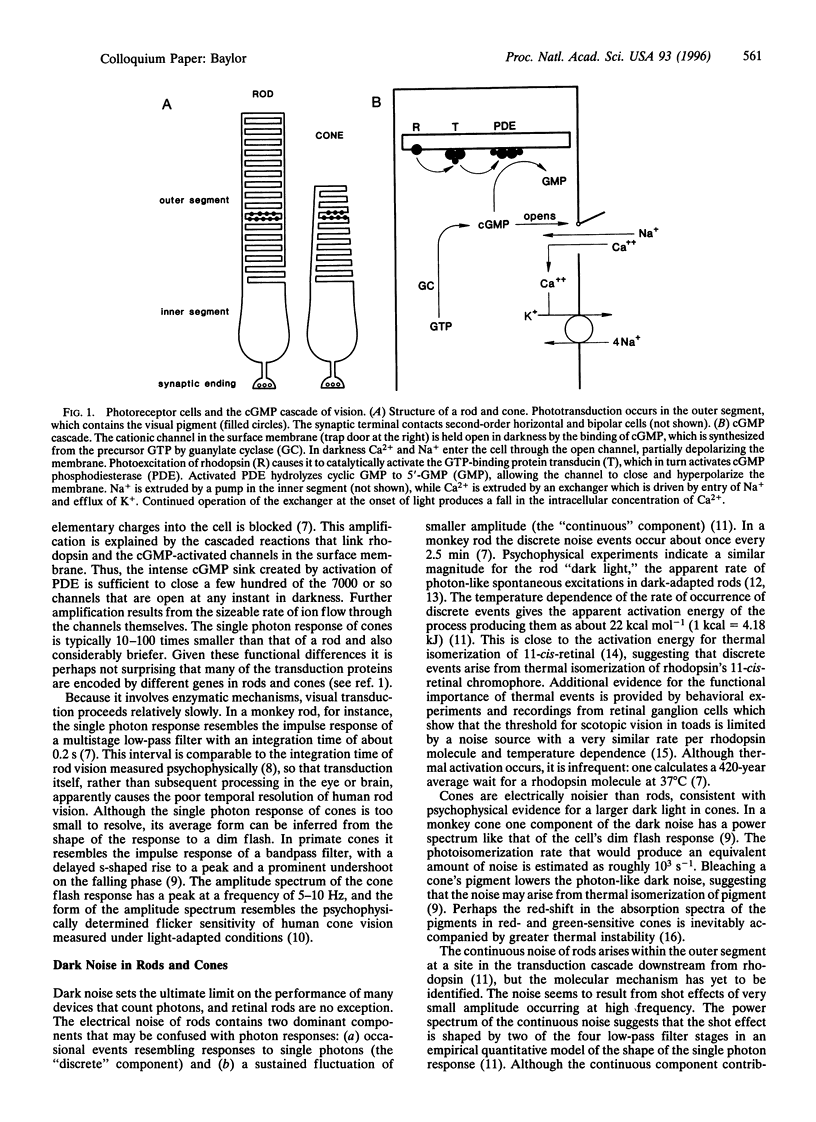
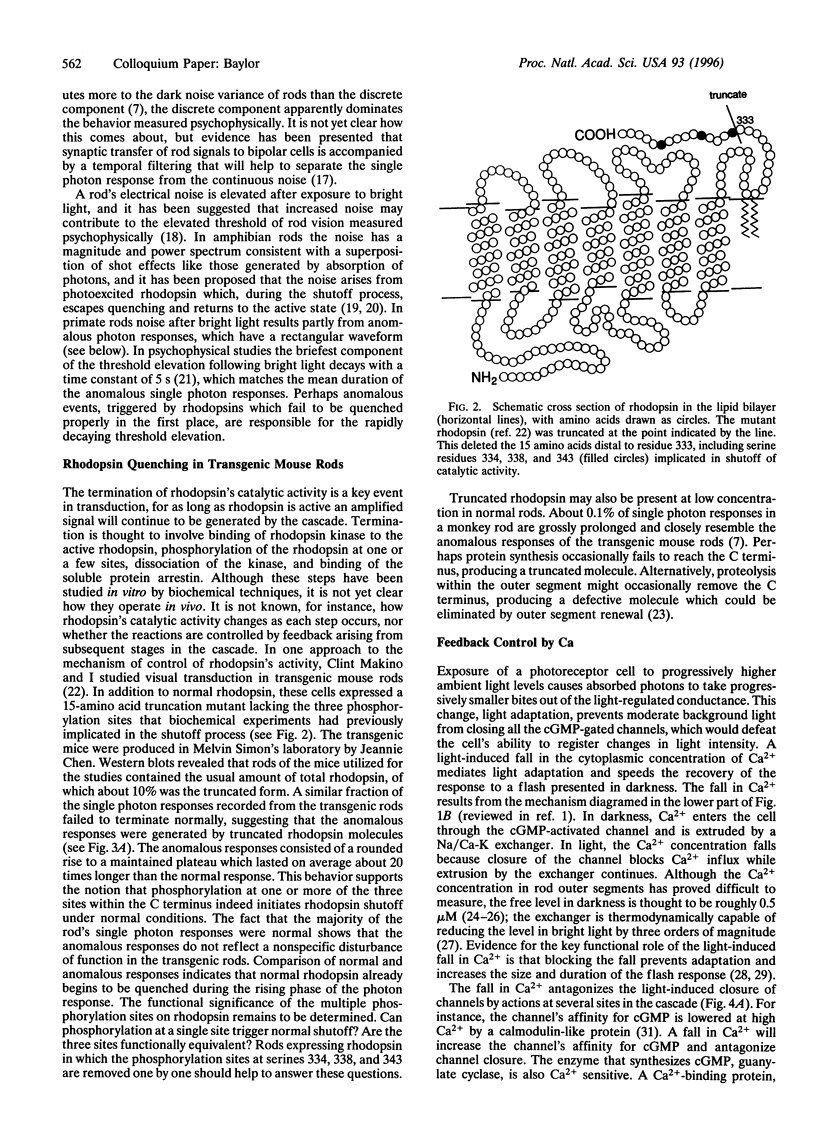
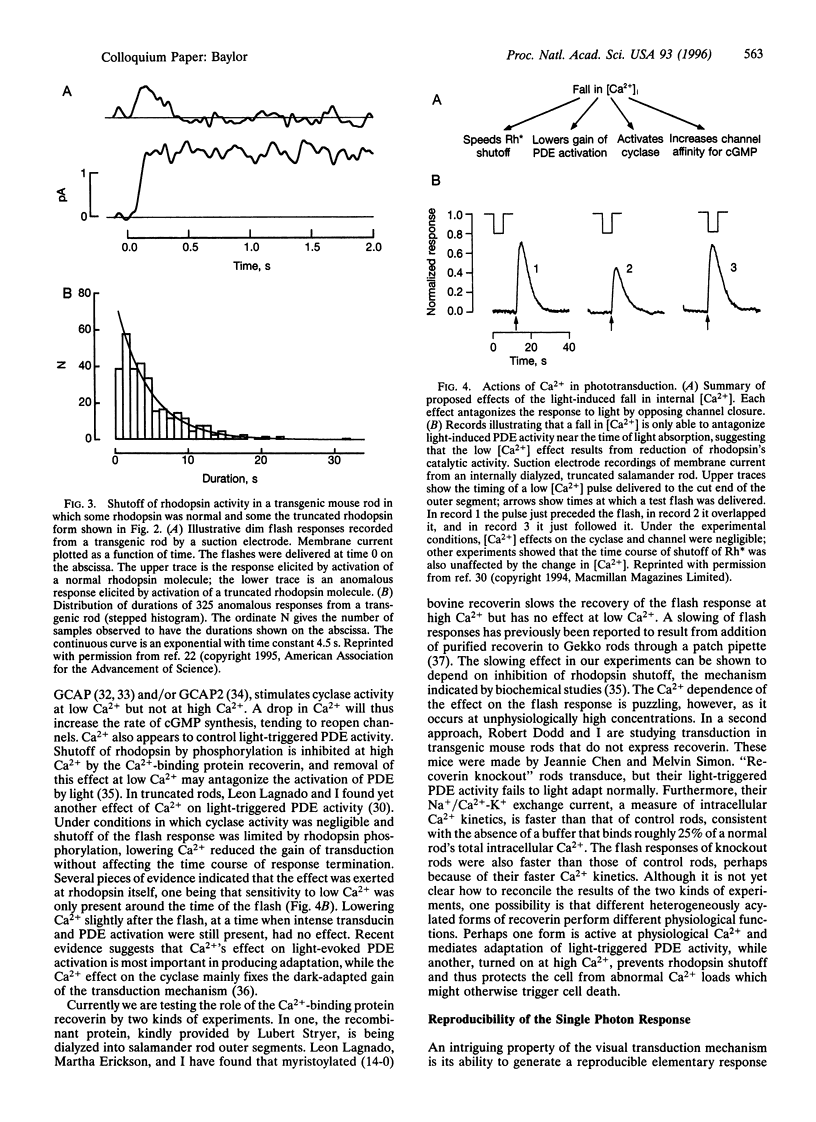
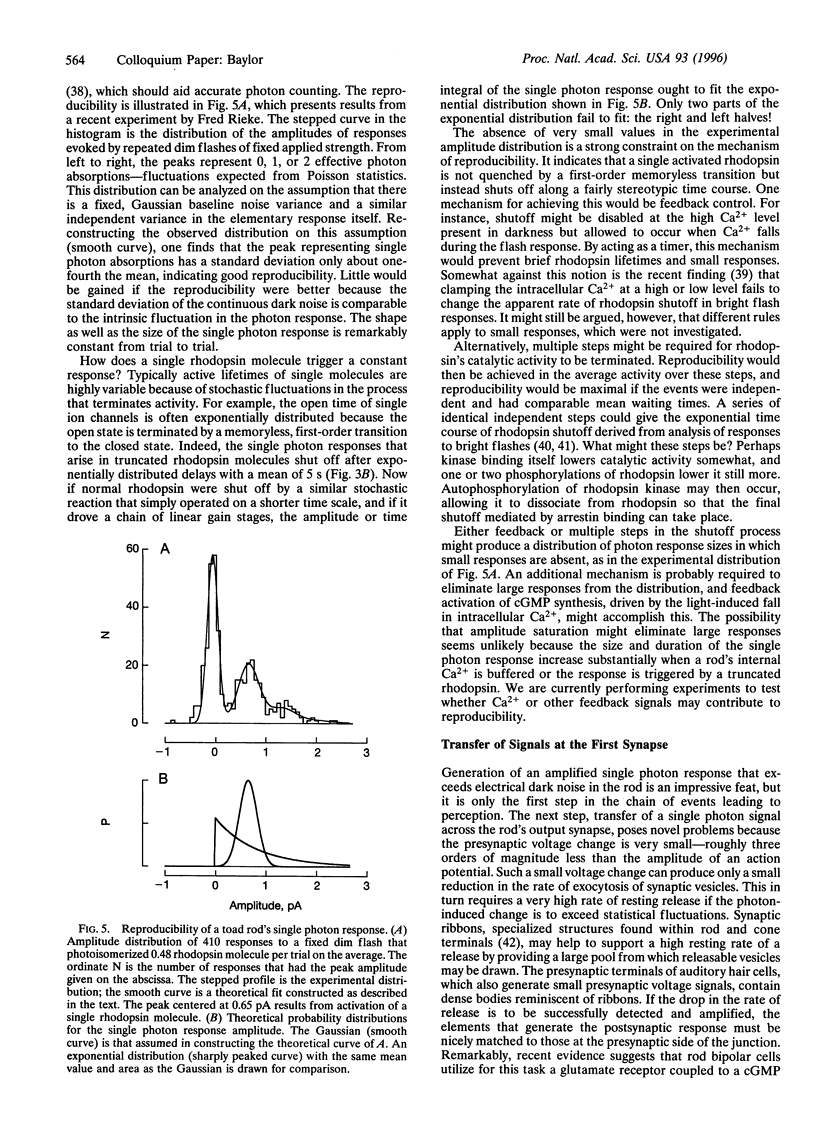
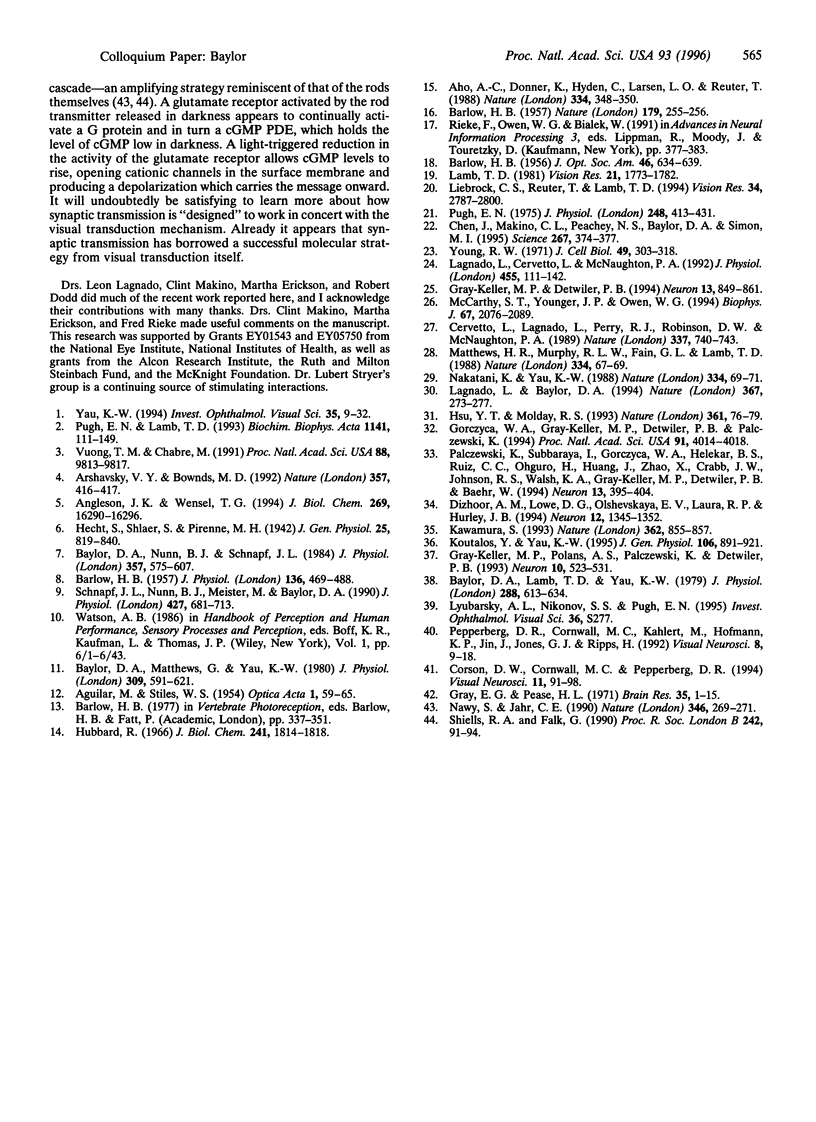
Selected References
These references are in PubMed. This may not be the complete list of references from this article.
- Aho A. C., Donner K., Hydén C., Larsen L. O., Reuter T. Low retinal noise in animals with low body temperature allows high visual sensitivity. Nature. 1988 Jul 28;334(6180):348–350. doi: 10.1038/334348a0. [DOI] [PubMed] [Google Scholar]
- Angleson J. K., Wensel T. G. Enhancement of rod outer segment GTPase accelerating protein activity by the inhibitory subunit of cGMP phosphodiesterase. J Biol Chem. 1994 Jun 10;269(23):16290–16296. [PubMed] [Google Scholar]
- Arshavsky VYu, Bownds M. D. Regulation of deactivation of photoreceptor G protein by its target enzyme and cGMP. Nature. 1992 Jun 4;357(6377):416–417. doi: 10.1038/357416a0. [DOI] [PMC free article] [PubMed] [Google Scholar]
- BARLOW H. B. Increment thresholds at low intensities considered as signal/noise discriminations. J Physiol. 1957 May 23;136(3):469–488. doi: 10.1113/jphysiol.1957.sp005774. [DOI] [PMC free article] [PubMed] [Google Scholar]
- BARLOW H. B. Purkinje shift and retinal noise. Nature. 1957 Feb 2;179(4553):255–256. doi: 10.1038/179255b0. [DOI] [PubMed] [Google Scholar]
- BARLOW H. B. Retinal noise and absolute threshold. J Opt Soc Am. 1956 Aug;46(8):634–639. doi: 10.1364/josa.46.000634. [DOI] [PubMed] [Google Scholar]
- Baylor D. A., Lamb T. D., Yau K. W. Responses of retinal rods to single photons. J Physiol. 1979 Mar;288:613–634. [PMC free article] [PubMed] [Google Scholar]
- Baylor D. A., Matthews G., Yau K. W. Two components of electrical dark noise in toad retinal rod outer segments. J Physiol. 1980 Dec;309:591–621. doi: 10.1113/jphysiol.1980.sp013529. [DOI] [PMC free article] [PubMed] [Google Scholar]
- Baylor D. A., Nunn B. J., Schnapf J. L. The photocurrent, noise and spectral sensitivity of rods of the monkey Macaca fascicularis. J Physiol. 1984 Dec;357:575–607. doi: 10.1113/jphysiol.1984.sp015518. [DOI] [PMC free article] [PubMed] [Google Scholar]
- Cervetto L., Lagnado L., Perry R. J., Robinson D. W., McNaughton P. A. Extrusion of calcium from rod outer segments is driven by both sodium and potassium gradients. Nature. 1989 Feb 23;337(6209):740–743. doi: 10.1038/337740a0. [DOI] [PubMed] [Google Scholar]
- Chen J., Makino C. L., Peachey N. S., Baylor D. A., Simon M. I. Mechanisms of rhodopsin inactivation in vivo as revealed by a COOH-terminal truncation mutant. Science. 1995 Jan 20;267(5196):374–377. doi: 10.1126/science.7824934. [DOI] [PubMed] [Google Scholar]
- Corson D. W., Cornwall M. C., Pepperberg D. R. Evidence for the prolonged photoactivated lifetime of an analogue visual pigment containing 11-cis 9-desmethylretinal. Vis Neurosci. 1994 Jan-Feb;11(1):91–98. doi: 10.1017/s0952523800011135. [DOI] [PubMed] [Google Scholar]
- Dizhoor A. M., Lowe D. G., Olshevskaya E. V., Laura R. P., Hurley J. B. The human photoreceptor membrane guanylyl cyclase, RetGC, is present in outer segments and is regulated by calcium and a soluble activator. Neuron. 1994 Jun;12(6):1345–1352. doi: 10.1016/0896-6273(94)90449-9. [DOI] [PubMed] [Google Scholar]
- Gorczyca W. A., Gray-Keller M. P., Detwiler P. B., Palczewski K. Purification and physiological evaluation of a guanylate cyclase activating protein from retinal rods. Proc Natl Acad Sci U S A. 1994 Apr 26;91(9):4014–4018. doi: 10.1073/pnas.91.9.4014. [DOI] [PMC free article] [PubMed] [Google Scholar]
- Gray-Keller M. P., Detwiler P. B. The calcium feedback signal in the phototransduction cascade of vertebrate rods. Neuron. 1994 Oct;13(4):849–861. doi: 10.1016/0896-6273(94)90251-8. [DOI] [PubMed] [Google Scholar]
- Gray-Keller M. P., Polans A. S., Palczewski K., Detwiler P. B. The effect of recoverin-like calcium-binding proteins on the photoresponse of retinal rods. Neuron. 1993 Mar;10(3):523–531. doi: 10.1016/0896-6273(93)90339-s. [DOI] [PubMed] [Google Scholar]
- Gray E. G., Pease H. L. On understanding the organisation of the retinal receptor synapses. Brain Res. 1971 Dec 10;35(1):1–15. doi: 10.1016/0006-8993(71)90591-9. [DOI] [PubMed] [Google Scholar]
- Hecht S., Shlaer S., Pirenne M. H. ENERGY, QUANTA, AND VISION. J Gen Physiol. 1942 Jul 20;25(6):819–840. doi: 10.1085/jgp.25.6.819. [DOI] [PMC free article] [PubMed] [Google Scholar]
- Hsu Y. T., Molday R. S. Modulation of the cGMP-gated channel of rod photoreceptor cells by calmodulin. Nature. 1993 Jan 7;361(6407):76–79. doi: 10.1038/361076a0. [DOI] [PubMed] [Google Scholar]
- Hubbard R. The stereoisomerization of 11-cis-retinal. J Biol Chem. 1966 Apr 25;241(8):1814–1818. [PubMed] [Google Scholar]
- Kawamura S. Rhodopsin phosphorylation as a mechanism of cyclic GMP phosphodiesterase regulation by S-modulin. Nature. 1993 Apr 29;362(6423):855–857. doi: 10.1038/362855a0. [DOI] [PubMed] [Google Scholar]
- Koutalos Y., Nakatani K., Yau K. W. The cGMP-phosphodiesterase and its contribution to sensitivity regulation in retinal rods. J Gen Physiol. 1995 Nov;106(5):891–921. doi: 10.1085/jgp.106.5.891. [DOI] [PMC free article] [PubMed] [Google Scholar]
- Lagnado L., Baylor D. A. Calcium controls light-triggered formation of catalytically active rhodopsin. Nature. 1994 Jan 20;367(6460):273–277. doi: 10.1038/367273a0. [DOI] [PubMed] [Google Scholar]
- Lagnado L., Cervetto L., McNaughton P. A. Calcium homeostasis in the outer segments of retinal rods from the tiger salamander. J Physiol. 1992 Sep;455:111–142. doi: 10.1113/jphysiol.1992.sp019293. [DOI] [PMC free article] [PubMed] [Google Scholar]
- Lamb T. D. The involvement of rod photoreceptors in dark adaptation. Vision Res. 1981;21(12):1773–1782. doi: 10.1016/0042-6989(81)90211-x. [DOI] [PubMed] [Google Scholar]
- Leibrock C. S., Reuter T., Lamb T. D. Dark adaptation of toad rod photoreceptors following small bleaches. Vision Res. 1994 Nov;34(21):2787–2800. doi: 10.1016/0042-6989(94)90048-5. [DOI] [PubMed] [Google Scholar]
- Matthews H. R., Murphy R. L., Fain G. L., Lamb T. D. Photoreceptor light adaptation is mediated by cytoplasmic calcium concentration. Nature. 1988 Jul 7;334(6177):67–69. doi: 10.1038/334067a0. [DOI] [PubMed] [Google Scholar]
- McCarthy S. T., Younger J. P., Owen W. G. Free calcium concentrations in bullfrog rods determined in the presence of multiple forms of Fura-2. Biophys J. 1994 Nov;67(5):2076–2089. doi: 10.1016/S0006-3495(94)80691-5. [DOI] [PMC free article] [PubMed] [Google Scholar]
- Nakatani K., Yau K. W. Calcium and light adaptation in retinal rods and cones. Nature. 1988 Jul 7;334(6177):69–71. doi: 10.1038/334069a0. [DOI] [PubMed] [Google Scholar]
- Nawy S., Jahr C. E. Suppression by glutamate of cGMP-activated conductance in retinal bipolar cells. Nature. 1990 Jul 19;346(6281):269–271. doi: 10.1038/346269a0. [DOI] [PubMed] [Google Scholar]
- Palczewski K., Subbaraya I., Gorczyca W. A., Helekar B. S., Ruiz C. C., Ohguro H., Huang J., Zhao X., Crabb J. W., Johnson R. S. Molecular cloning and characterization of retinal photoreceptor guanylyl cyclase-activating protein. Neuron. 1994 Aug;13(2):395–404. doi: 10.1016/0896-6273(94)90355-7. [DOI] [PubMed] [Google Scholar]
- Pepperberg D. R., Cornwall M. C., Kahlert M., Hofmann K. P., Jin J., Jones G. J., Ripps H. Light-dependent delay in the falling phase of the retinal rod photoresponse. Vis Neurosci. 1992 Jan;8(1):9–18. doi: 10.1017/s0952523800006441. [DOI] [PubMed] [Google Scholar]
- Pugh E. N., Jr, Lamb T. D. Amplification and kinetics of the activation steps in phototransduction. Biochim Biophys Acta. 1993 Mar 1;1141(2-3):111–149. doi: 10.1016/0005-2728(93)90038-h. [DOI] [PubMed] [Google Scholar]
- Pugh E. N. Rushton's paradox: rod dark adaptation after flash photolysis. J Physiol. 1975 Jun;248(2):413–431. doi: 10.1113/jphysiol.1975.sp010982. [DOI] [PMC free article] [PubMed] [Google Scholar]
- Schnapf J. L., Nunn B. J., Meister M., Baylor D. A. Visual transduction in cones of the monkey Macaca fascicularis. J Physiol. 1990 Aug;427:681–713. doi: 10.1113/jphysiol.1990.sp018193. [DOI] [PMC free article] [PubMed] [Google Scholar]
- Shiells R. A., Falk G. Glutamate receptors of rod bipolar cells are linked to a cyclic GMP cascade via a G-protein. Proc Biol Sci. 1990 Nov 22;242(1304):91–94. doi: 10.1098/rspb.1990.0109. [DOI] [PubMed] [Google Scholar]
- Vuong T. M., Chabre M. Deactivation kinetics of the transduction cascade of vision. Proc Natl Acad Sci U S A. 1991 Nov 1;88(21):9813–9817. doi: 10.1073/pnas.88.21.9813. [DOI] [PMC free article] [PubMed] [Google Scholar]
- Yau K. W. Phototransduction mechanism in retinal rods and cones. The Friedenwald Lecture. Invest Ophthalmol Vis Sci. 1994 Jan;35(1):9–32. [PubMed] [Google Scholar]
- Young R. W. The renewal of rod and cone outer segments in the rhesus monkey. J Cell Biol. 1971 May 1;49(2):303–318. doi: 10.1083/jcb.49.2.303. [DOI] [PMC free article] [PubMed] [Google Scholar]