Abstract
Structurally neighboring residues are categorized according to their separation in the primary sequence as proximal (1-4 positions apart) and otherwise distal, which in turn is divided into near (5-20 positions), far (21-50 positions), very far ( > 50 positions), and interchain (from different chains of the same structure). These categories describe the linear distance histogram (LDH) for three-dimensional neighboring residue types. Among the main results are the following: (i) nearest-neighbor hydrophobic residues tend to be increasingly distally separated in the linear sequence, thus most often connecting distinct secondary structure units. (ii) The LDHs of oppositely charged nearest-neighbors emphasize proximal positions with a subsidiary maximum for very far positions. (iii) Cysteine-cysteine structural interactions rarely involve proximal positions. (iv) The greatest numbers of interchain specific nearest-neighbors in protein structures are composed of oppositely charged residues. (v) The largest fraction of side-chain neighboring residues from beta-strands involves near positions, emphasizing associations between consecutive strands. (vi) Exposed residue pairs are predominantly located in proximal linear positions, while buried residue pairs principally correspond to far or very far distal positions. The results are principally invariant to protein sizes, amino acid usages, linear distance normalizations, and over- and underrepresentations among nearest-neighbor types. Interpretations and hypotheses concerning the LDHs, particularly those of hydrophobic and charged pairings, are discussed with respect to protein stability and functionality. The pronounced occurrence of oppositely charged interchain contacts is consistent with many observations on protein complexes where multichain stabilization is facilitated by electrostatic interactions.
Full text
PDF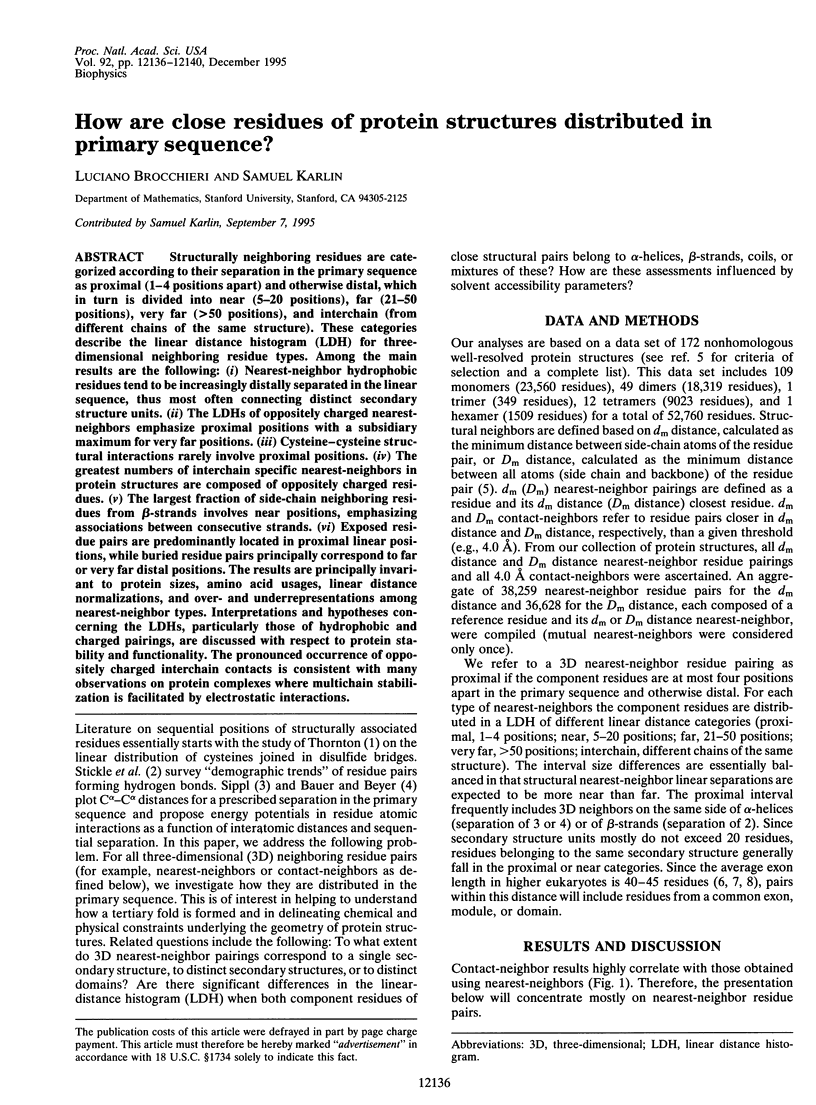
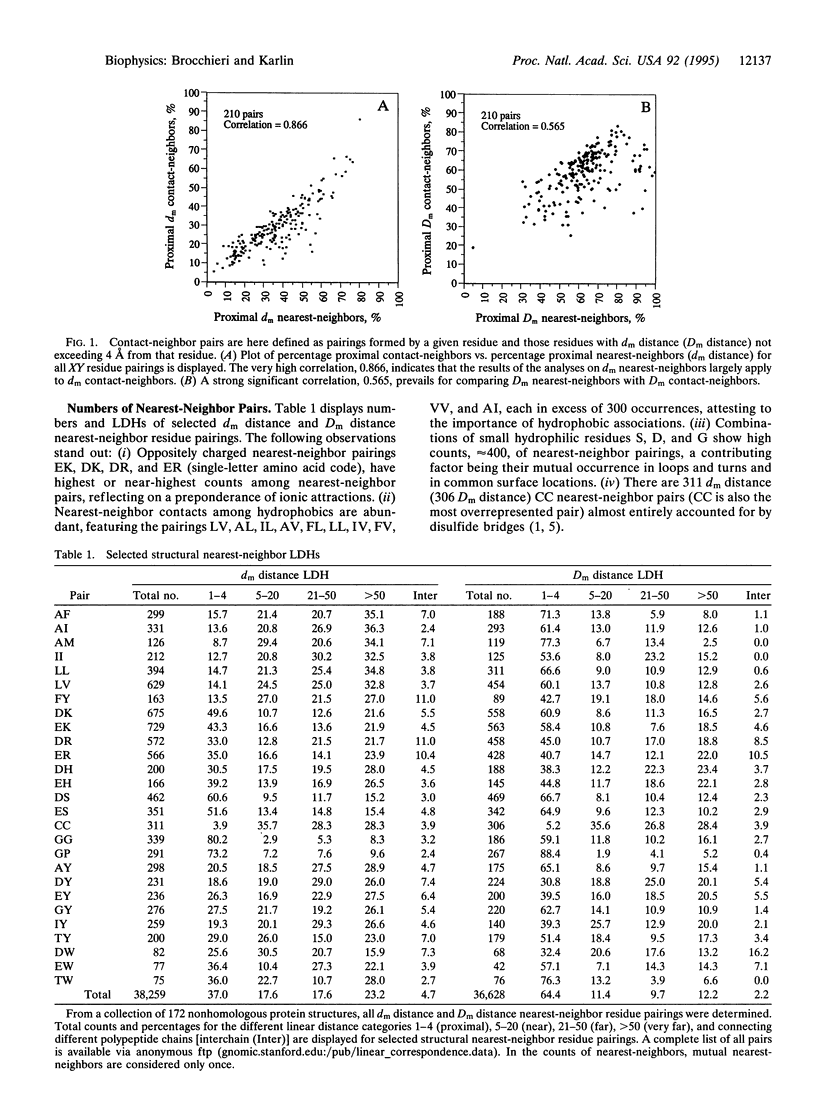
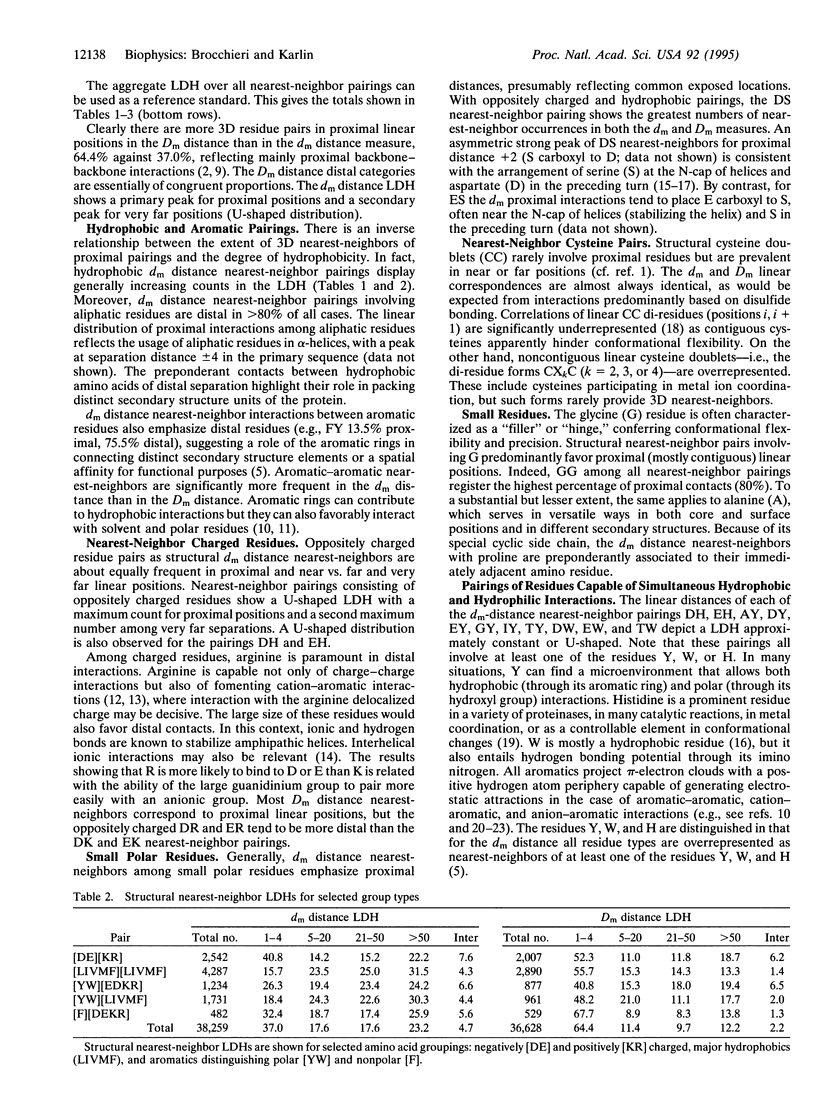
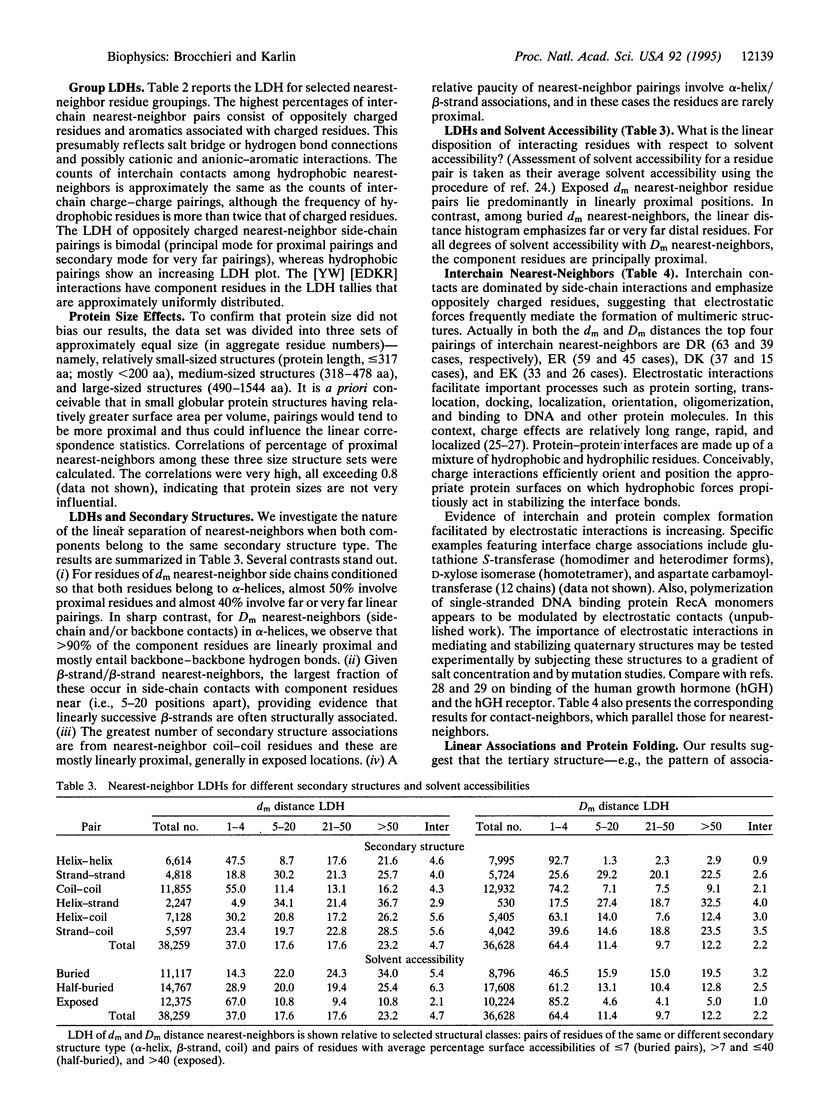
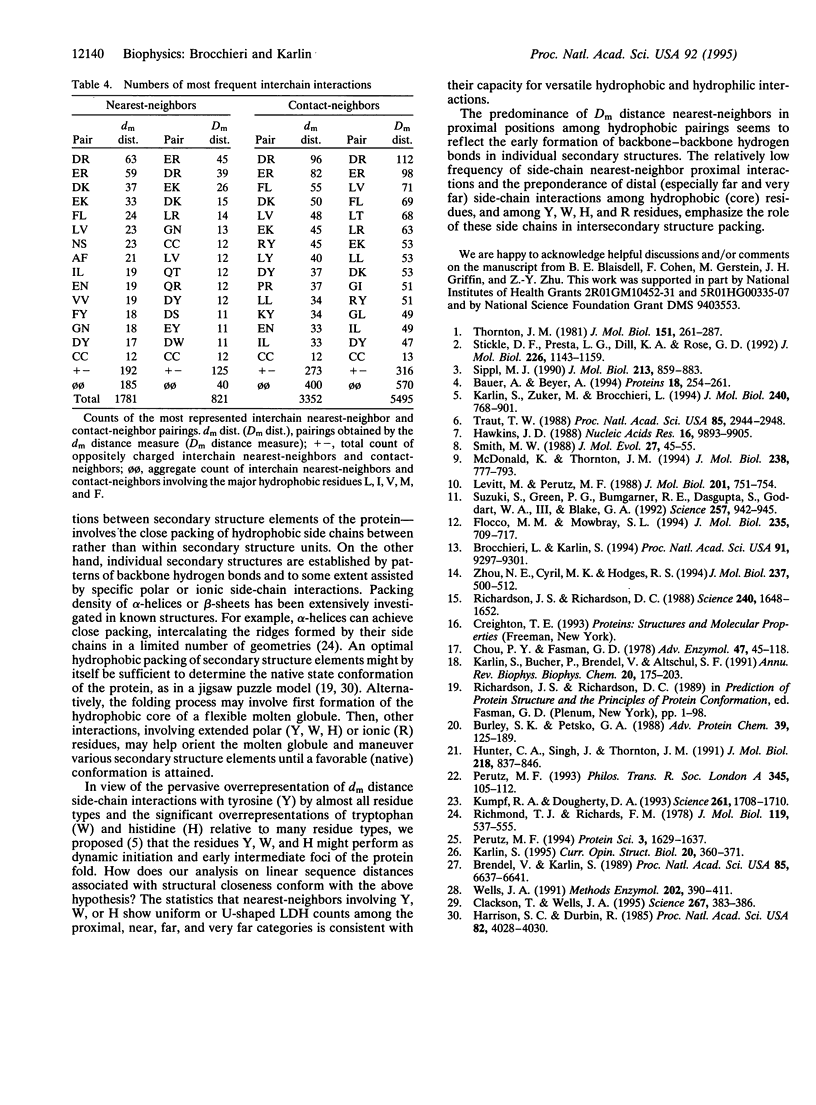
Selected References
These references are in PubMed. This may not be the complete list of references from this article.
- Bauer A., Beyer A. An improved pair potential to recognize native protein folds. Proteins. 1994 Mar;18(3):254–261. doi: 10.1002/prot.340180306. [DOI] [PubMed] [Google Scholar]
- Blaisdell B. E., Karlin S. Distinctive charge configurations in proteins of the Epstein-Barr virus and possible functions. Proc Natl Acad Sci U S A. 1988 Sep;85(18):6637–6641. doi: 10.1073/pnas.85.18.6637. [DOI] [PMC free article] [PubMed] [Google Scholar]
- Brocchieri L., Karlin S. Geometry of interplanar residue contacts in protein structures. Proc Natl Acad Sci U S A. 1994 Sep 27;91(20):9297–9301. doi: 10.1073/pnas.91.20.9297. [DOI] [PMC free article] [PubMed] [Google Scholar]
- Burley S. K., Petsko G. A. Weakly polar interactions in proteins. Adv Protein Chem. 1988;39:125–189. doi: 10.1016/s0065-3233(08)60376-9. [DOI] [PubMed] [Google Scholar]
- Chou P. Y., Fasman G. D. Prediction of the secondary structure of proteins from their amino acid sequence. Adv Enzymol Relat Areas Mol Biol. 1978;47:45–148. doi: 10.1002/9780470122921.ch2. [DOI] [PubMed] [Google Scholar]
- Clackson T., Wells J. A. A hot spot of binding energy in a hormone-receptor interface. Science. 1995 Jan 20;267(5196):383–386. doi: 10.1126/science.7529940. [DOI] [PubMed] [Google Scholar]
- Flocco M. M., Mowbray S. L. Planar stacking interactions of arginine and aromatic side-chains in proteins. J Mol Biol. 1994 Jan 14;235(2):709–717. doi: 10.1006/jmbi.1994.1022. [DOI] [PubMed] [Google Scholar]
- Harrison S. C., Durbin R. Is there a single pathway for the folding of a polypeptide chain? Proc Natl Acad Sci U S A. 1985 Jun;82(12):4028–4030. doi: 10.1073/pnas.82.12.4028. [DOI] [PMC free article] [PubMed] [Google Scholar]
- Hawkins J. D. A survey on intron and exon lengths. Nucleic Acids Res. 1988 Nov 11;16(21):9893–9908. doi: 10.1093/nar/16.21.9893. [DOI] [PMC free article] [PubMed] [Google Scholar]
- Hunter C. A., Singh J., Thornton J. M. Pi-pi interactions: the geometry and energetics of phenylalanine-phenylalanine interactions in proteins. J Mol Biol. 1991 Apr 20;218(4):837–846. doi: 10.1016/0022-2836(91)90271-7. [DOI] [PubMed] [Google Scholar]
- Karlin S., Bucher P., Brendel V., Altschul S. F. Statistical methods and insights for protein and DNA sequences. Annu Rev Biophys Biophys Chem. 1991;20:175–203. doi: 10.1146/annurev.bb.20.060191.001135. [DOI] [PubMed] [Google Scholar]
- Karlin S. Statistical significance of sequence patterns in proteins. Curr Opin Struct Biol. 1995 Jun;5(3):360–371. doi: 10.1016/0959-440x(95)80098-0. [DOI] [PubMed] [Google Scholar]
- Kumpf R. A., Dougherty D. A. A mechanism for ion selectivity in potassium channels: computational studies of cation-pi interactions. Science. 1993 Sep 24;261(5129):1708–1710. doi: 10.1126/science.8378771. [DOI] [PubMed] [Google Scholar]
- Levitt M., Perutz M. F. Aromatic rings act as hydrogen bond acceptors. J Mol Biol. 1988 Jun 20;201(4):751–754. doi: 10.1016/0022-2836(88)90471-8. [DOI] [PubMed] [Google Scholar]
- McDonald I. K., Thornton J. M. Satisfying hydrogen bonding potential in proteins. J Mol Biol. 1994 May 20;238(5):777–793. doi: 10.1006/jmbi.1994.1334. [DOI] [PubMed] [Google Scholar]
- Perutz M. Polar zippers: their role in human disease. Protein Sci. 1994 Oct;3(10):1629–1637. doi: 10.1002/pro.5560031002. [DOI] [PMC free article] [PubMed] [Google Scholar]
- Richardson J. S., Richardson D. C. Amino acid preferences for specific locations at the ends of alpha helices. Science. 1988 Jun 17;240(4859):1648–1652. doi: 10.1126/science.3381086. [DOI] [PubMed] [Google Scholar]
- Richmond T. J., Richards F. M. Packing of alpha-helices: geometrical constraints and contact areas. J Mol Biol. 1978 Mar 15;119(4):537–555. doi: 10.1016/0022-2836(78)90201-2. [DOI] [PubMed] [Google Scholar]
- Sippl M. J. Calculation of conformational ensembles from potentials of mean force. An approach to the knowledge-based prediction of local structures in globular proteins. J Mol Biol. 1990 Jun 20;213(4):859–883. doi: 10.1016/s0022-2836(05)80269-4. [DOI] [PubMed] [Google Scholar]
- Smith M. W. Structure of vertebrate genes: a statistical analysis implicating selection. J Mol Evol. 1988;27(1):45–55. doi: 10.1007/BF02099729. [DOI] [PubMed] [Google Scholar]
- Stickle D. F., Presta L. G., Dill K. A., Rose G. D. Hydrogen bonding in globular proteins. J Mol Biol. 1992 Aug 20;226(4):1143–1159. doi: 10.1016/0022-2836(92)91058-w. [DOI] [PubMed] [Google Scholar]
- Suzuki S., Green P. G., Bumgarner R. E., Dasgupta S., Goddard W. A., 3rd, Blake G. A. Benzene forms hydrogen bonds with water. Science. 1992 Aug 14;257(5072):942–945. doi: 10.1126/science.257.5072.942. [DOI] [PubMed] [Google Scholar]
- Thornton J. M. Disulphide bridges in globular proteins. J Mol Biol. 1981 Sep 15;151(2):261–287. doi: 10.1016/0022-2836(81)90515-5. [DOI] [PubMed] [Google Scholar]
- Traut T. W. Do exons code for structural or functional units in proteins? Proc Natl Acad Sci U S A. 1988 May;85(9):2944–2948. doi: 10.1073/pnas.85.9.2944. [DOI] [PMC free article] [PubMed] [Google Scholar]
- Wells J. A. Systematic mutational analyses of protein-protein interfaces. Methods Enzymol. 1991;202:390–411. doi: 10.1016/0076-6879(91)02020-a. [DOI] [PubMed] [Google Scholar]
- Zhou N. E., Kay C. M., Hodges R. S. The role of interhelical ionic interactions in controlling protein folding and stability. De novo designed synthetic two-stranded alpha-helical coiled-coils. J Mol Biol. 1994 Apr 8;237(4):500–512. doi: 10.1006/jmbi.1994.1250. [DOI] [PubMed] [Google Scholar]