Abstract
As a measure of dynamical structure, short-term fluctuations of coherence between 0.3 and 100 Hz in the electroencephalogram (EEG) of humans were studied from recordings made by chronic subdural macroelectrodes 5-10 mm apart, on temporal, frontal, and parietal lobes, and from intracranial probes deep in the temporal lobe, including the hippocampus, during sleep, alert, and seizure states. The time series of coherence between adjacent sites calculated every second or less often varies widely in stability over time; sometimes it is stable for half a minute or more. Within 2-min samples, coherence commonly fluctuates by a factor up to 2-3, in all bands, within the time scale of seconds to tens of seconds. The power spectrum of the time series of these fluctuations is broad, extending to 0.02 Hz or slower, and is weighted toward the slower frequencies; little power is faster than 0.5 Hz. Some records show conspicuous swings with a preferred duration of 5-15s, either irregularly or quasirhythmically with a broad peak around 0.1 Hz. Periodicity is not statistically significant in most records. In our sampling, we have not found a consistent difference between lobes of the brain, subdural and depth electrodes, or sleeping and waking states. Seizures generally raise the mean coherence in all frequencies and may reduce the fluctuations by a ceiling effect. The coherence time series of different bands is positively correlated (0.45 overall); significant nonindependence extends for at least two octaves. Coherence fluctuations are quite local; the time series of adjacent electrodes is correlated with that of the nearest neighbor pairs (10 mm) to a coefficient averaging approximately 0.4, falling to approximately 0.2 for neighbors-but-one (20 mm) and to < 0.1 for neighbors-but-two (30 mm). The evidence indicates fine structure in time and space, a dynamic and local determination of this measure of cooperativity. Widely separated frequencies tending to fluctuate together exclude independent oscillators as the general or usual basis of the EEG, although a few rhythms are well known under special conditions. Broad-band events may be the more usual generators. Loci only a few millimeters apart can fluctuate widely in seconds, either in parallel or independently. Scalp EEG coherence cannot be predicted from subdural or deep recordings, or vice versa, and intracortical microelectrodes show still greater coherence fluctuation in space and time. Widely used computations of chaos and dimensionality made upon data from scalp or even subdural or depth electrodes, even when reproducible in successive samples, cannot be considered representative of the brain or the given structure or brain state but only of the scale or view (receptive field) of the electrodes used. Relevant to the evolution of more complex brains, which is an outstanding fact of animal evolution, we believe that measures of cooperativity are likely to be among the dynamic features by which major evolutionary grades of brains differ.
Full text
PDF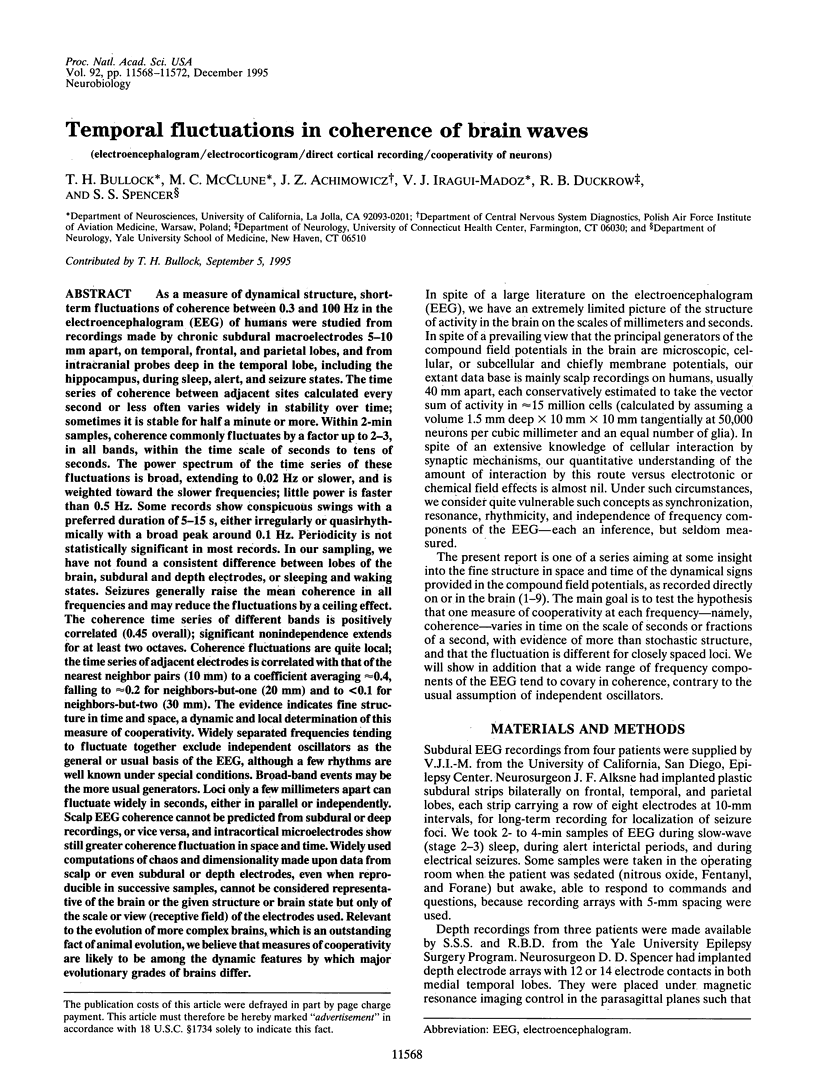
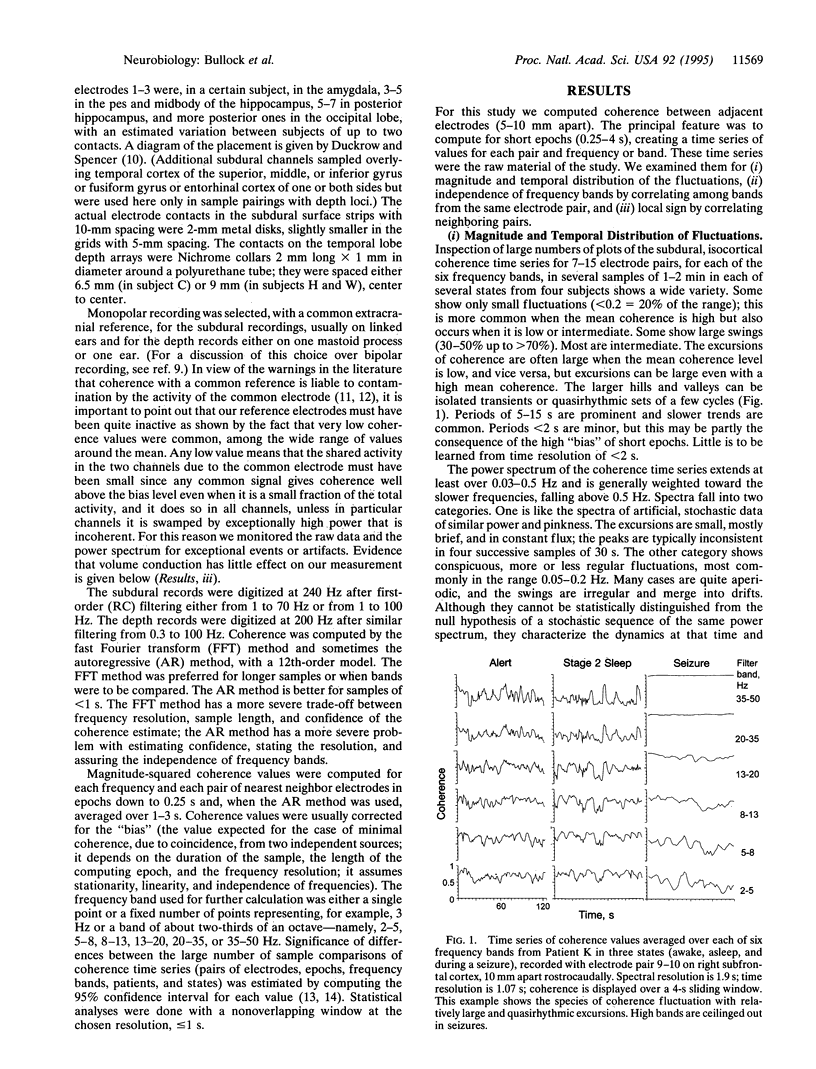
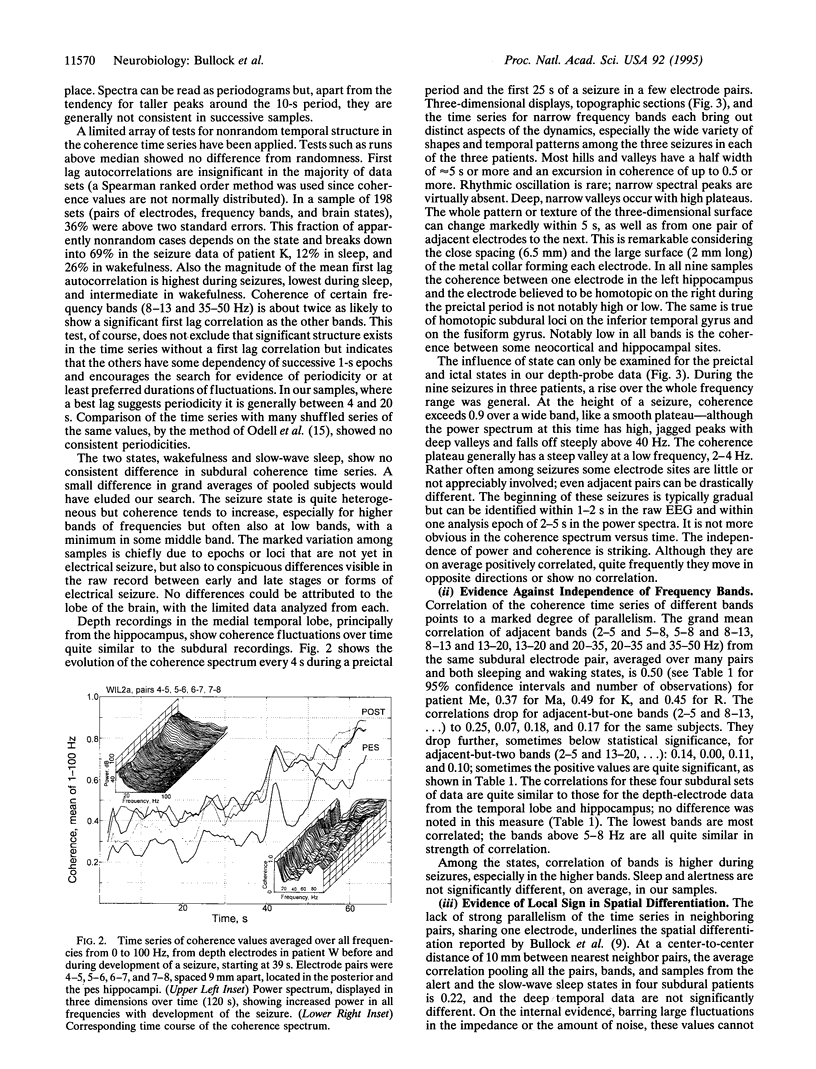
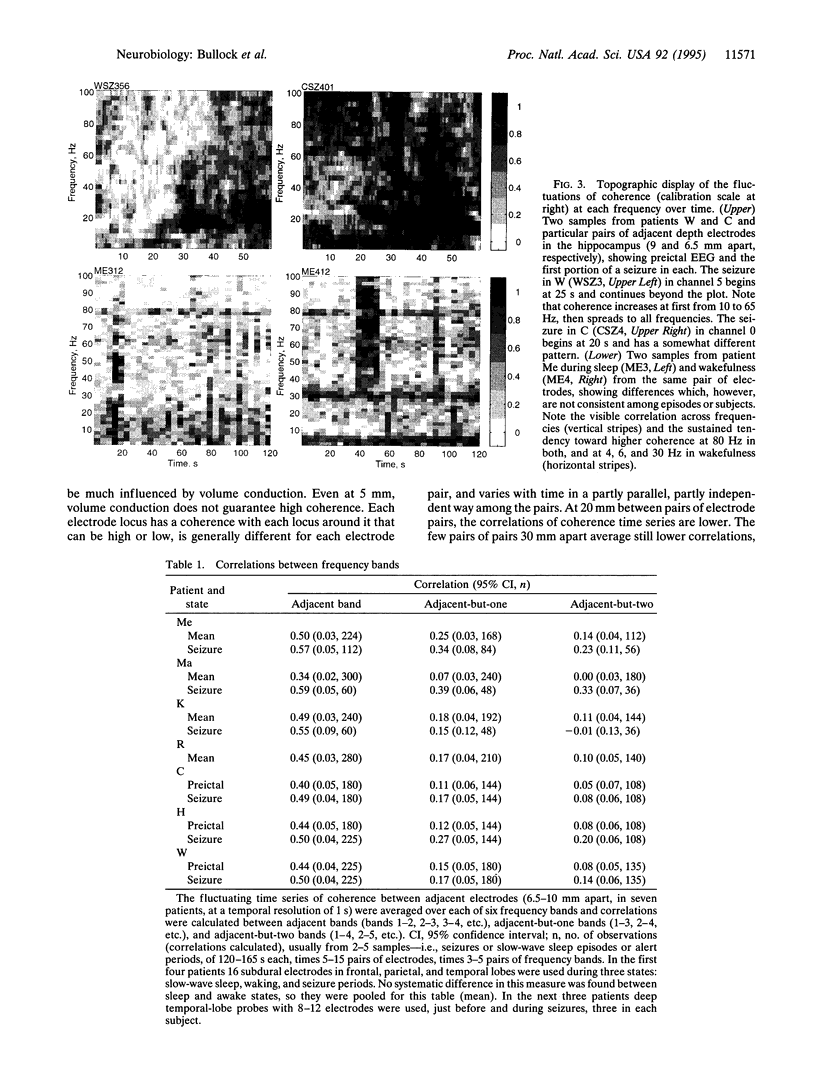
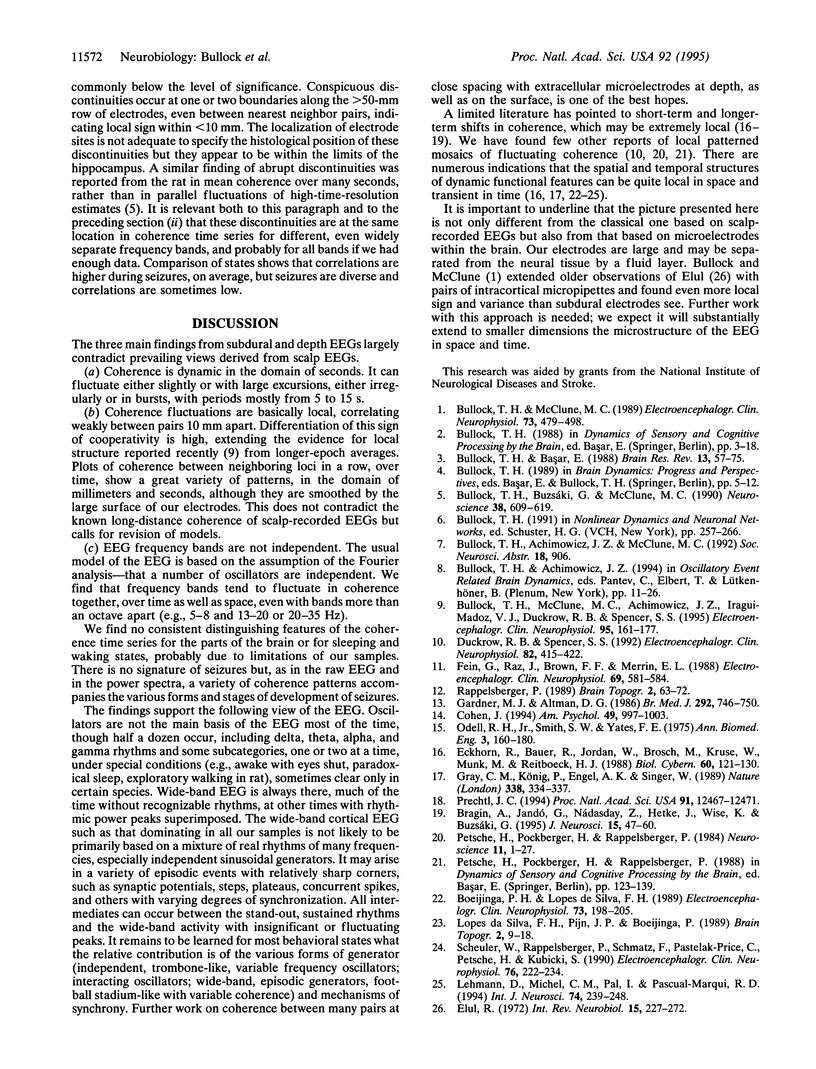
Images in this article
Selected References
These references are in PubMed. This may not be the complete list of references from this article.
- Boeijinga P. H., Lopes da Silva F. H. A new method to estimate time delays between EEG signals applied to beta activity of the olfactory cortical areas. Electroencephalogr Clin Neurophysiol. 1989 Sep;73(3):198–205. doi: 10.1016/0013-4694(89)90120-x. [DOI] [PubMed] [Google Scholar]
- Bragin A., Jandó G., Nádasdy Z., Hetke J., Wise K., Buzsáki G. Gamma (40-100 Hz) oscillation in the hippocampus of the behaving rat. J Neurosci. 1995 Jan;15(1 Pt 1):47–60. doi: 10.1523/JNEUROSCI.15-01-00047.1995. [DOI] [PMC free article] [PubMed] [Google Scholar]
- Bullock T. H., Basar E. Comparison of ongoing compound field potentials in the brains of invertebrates and vertebrates. Brain Res. 1988 Jan-Mar;472(1):57–75. doi: 10.1016/0165-0173(88)90005-7. [DOI] [PubMed] [Google Scholar]
- Bullock T. H., Buzsáki G., McClune M. C. Coherence of compound field potentials reveals discontinuities in the CA1-subiculum of the hippocampus in freely-moving rats. Neuroscience. 1990;38(3):609–619. doi: 10.1016/0306-4522(90)90055-9. [DOI] [PubMed] [Google Scholar]
- Bullock T. H., McClune M. C., Achimowicz J. Z., Iragui-Madoz V. J., Duckrow R. B., Spencer S. S. EEG coherence has structure in the millimeter domain: subdural and hippocampal recordings from epileptic patients. Electroencephalogr Clin Neurophysiol. 1995 Sep;95(3):161–177. doi: 10.1016/0013-4694(95)93347-a. [DOI] [PubMed] [Google Scholar]
- Bullock T. H., McClune M. C. Lateral coherence of the electrocorticogram: a new measure of brain synchrony. Electroencephalogr Clin Neurophysiol. 1989 Dec;73(6):479–498. doi: 10.1016/0013-4694(89)90258-7. [DOI] [PubMed] [Google Scholar]
- Duckrow R. B., Spencer S. S. Regional coherence and the transfer of ictal activity during seizure onset in the medial temporal lobe. Electroencephalogr Clin Neurophysiol. 1992 Jun;82(6):415–422. doi: 10.1016/0013-4694(92)90046-k. [DOI] [PubMed] [Google Scholar]
- Eckhorn R., Bauer R., Jordan W., Brosch M., Kruse W., Munk M., Reitboeck H. J. Coherent oscillations: a mechanism of feature linking in the visual cortex? Multiple electrode and correlation analyses in the cat. Biol Cybern. 1988;60(2):121–130. doi: 10.1007/BF00202899. [DOI] [PubMed] [Google Scholar]
- Elul R. The genesis of the EEG. Int Rev Neurobiol. 1971 Jul;15:227–272. doi: 10.1016/s0074-7742(08)60333-5. [DOI] [PubMed] [Google Scholar]
- Fein G., Raz J., Brown F. F., Merrin E. L. Common reference coherence data are confounded by power and phase effects. Electroencephalogr Clin Neurophysiol. 1988 Jun;69(6):581–584. doi: 10.1016/0013-4694(88)90171-x. [DOI] [PubMed] [Google Scholar]
- Gardner M. J., Altman D. G. Confidence intervals rather than P values: estimation rather than hypothesis testing. Br Med J (Clin Res Ed) 1986 Mar 15;292(6522):746–750. doi: 10.1136/bmj.292.6522.746. [DOI] [PMC free article] [PubMed] [Google Scholar]
- Gray C. M., König P., Engel A. K., Singer W. Oscillatory responses in cat visual cortex exhibit inter-columnar synchronization which reflects global stimulus properties. Nature. 1989 Mar 23;338(6213):334–337. doi: 10.1038/338334a0. [DOI] [PubMed] [Google Scholar]
- Lehmann D., Michel C. M., Pal I., Pascual-Marqui R. D. Event-related potential maps depend on prestimulus brain electric microstate map. Int J Neurosci. 1994 Jan-Feb;74(1-4):239–248. doi: 10.3109/00207459408987242. [DOI] [PubMed] [Google Scholar]
- Lopes da Silva F., Pijn J. P., Boeijinga P. Interdependence of EEG signals: linear vs. nonlinear associations and the significance of time delays and phase shifts. Brain Topogr. 1989 Fall-Winter;2(1-2):9–18. doi: 10.1007/BF01128839. [DOI] [PubMed] [Google Scholar]
- Odell R. H., Jr, Smith S. W., Yates F. E. A permutation test for periodicities in short, noisy time series. Ann Biomed Eng. 1975 Jun;3(2):160–180. doi: 10.1007/BF02363068. [DOI] [PubMed] [Google Scholar]
- Petsche H., Pockberger H., Rappelsberger P. On the search for the sources of the electroencephalogram. Neuroscience. 1984 Jan;11(1):1–27. doi: 10.1016/0306-4522(84)90212-4. [DOI] [PubMed] [Google Scholar]
- Prechtl J. C. Visual motion induces synchronous oscillations in turtle visual cortex. Proc Natl Acad Sci U S A. 1994 Dec 20;91(26):12467–12471. doi: 10.1073/pnas.91.26.12467. [DOI] [PMC free article] [PubMed] [Google Scholar]
- Rappelsberger P. The reference problem and mapping of coherence: a simulation study. Brain Topogr. 1989 Fall-Winter;2(1-2):63–72. doi: 10.1007/BF01128844. [DOI] [PubMed] [Google Scholar]
- Scheuler W., Rappelsberger P., Schmatz F., Pastelak-Price C., Petsche H., Kubicki S. Periodicity analysis of sleep EEG in the second and minute ranges--example of application in different alpha activities in sleep. Electroencephalogr Clin Neurophysiol. 1990 Sep;76(3):222–234. doi: 10.1016/0013-4694(90)90017-e. [DOI] [PubMed] [Google Scholar]