Abstract
Rhodopsin is the G protein-coupled receptor that upon light activation triggers the visual transduction cascade. Rod cell outer segment disc membranes were isolated from dark-adapted frog retinas and were extracted with Tween detergents to obtain two-dimensional rhodopsin crystals for electron crystallography. When Tween 80 was used, tubular structures with a p2 lattice (a = 32 A, b = 83 A, gamma = 91 degrees) were formed. The use of a Tween 80/Tween 20 mixture favored the formation of larger p22(1)2(1) lattices (a = 40 A, b = 146 A, gamma = 90 degrees). Micrographs from frozen hydrated frog rhodopsin crystals were processed, and projection structures to 7-A resolution for the p22(1)2(1) form and to 6-A resolution for the p2 form were calculated. The maps of frog rhodopsin in both crystal forms are very similar to the 9-A map obtained previously for bovine rhodopsin and show that the arrangement of the helices is the same. In a tentative topographic model, helices 4, 6, and 7 are nearly perpendicular to the plane of the membrane. In the higher-resolution projection maps of frog rhodopsin, helix 5 looks more tilted than it appeared previously. The quality of the two frog rhodopsin crystals suggests that they would be suitable to obtain a three-dimensional structure in which all helices would be resolved.
Full text
PDF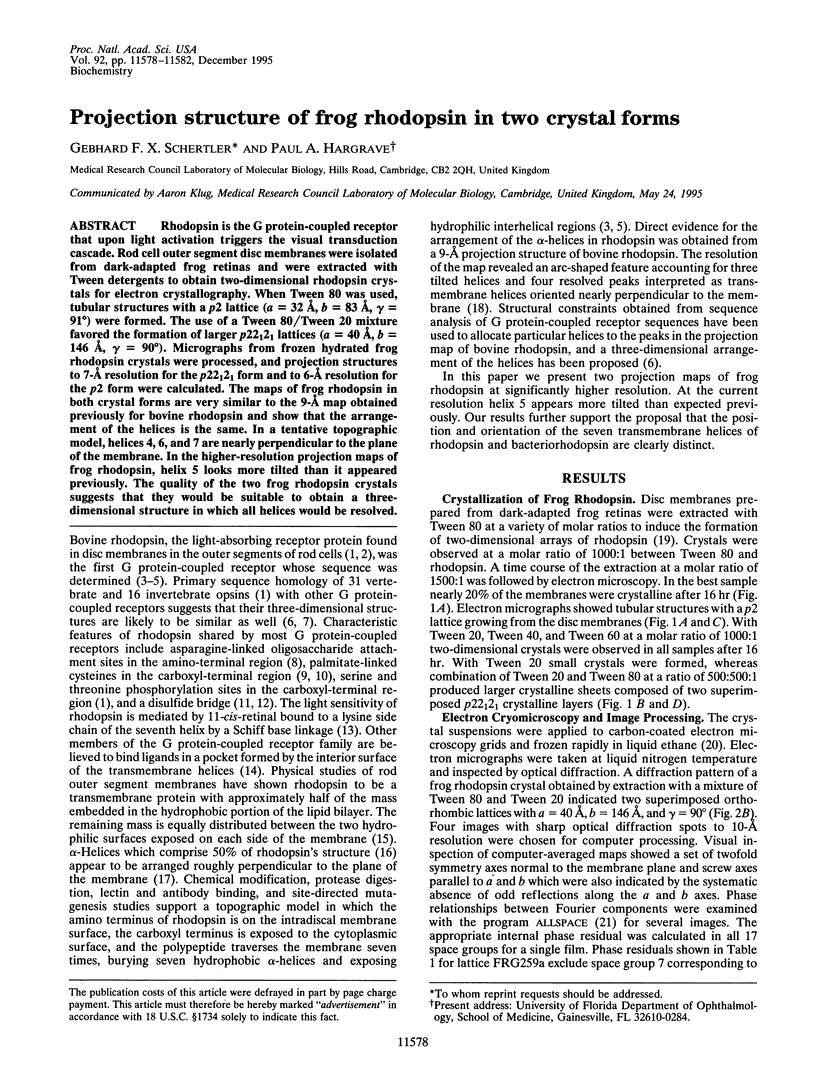
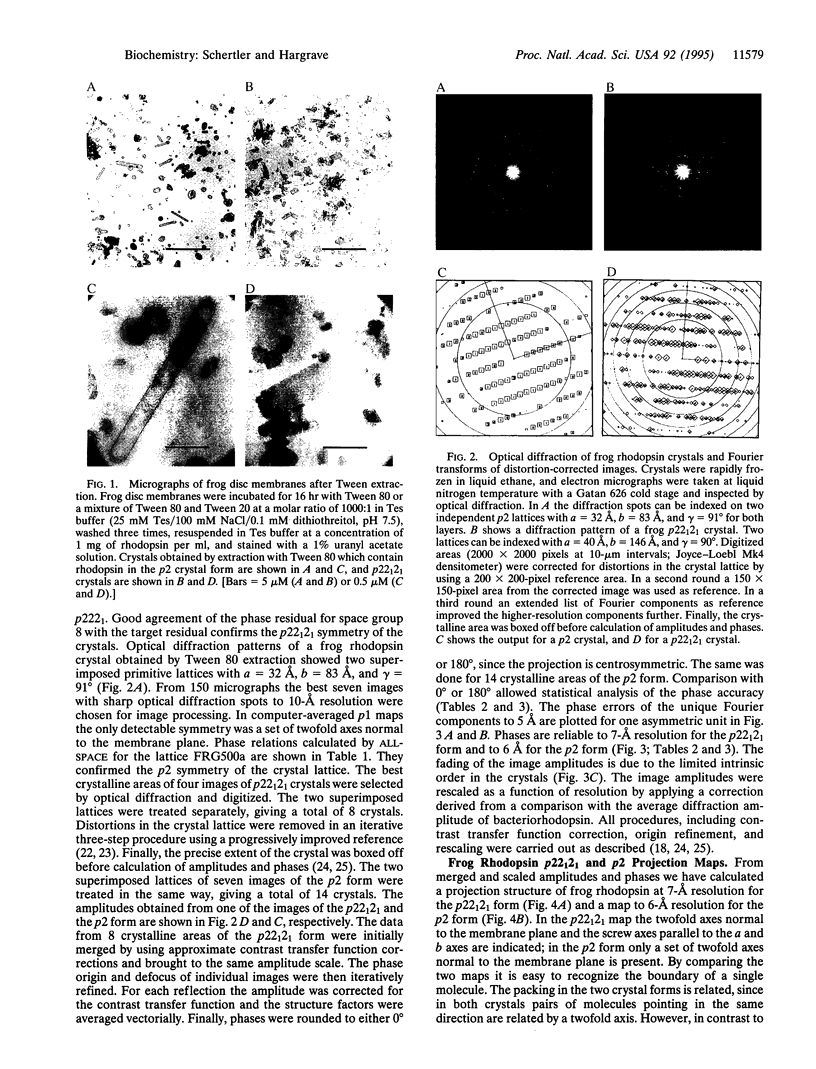
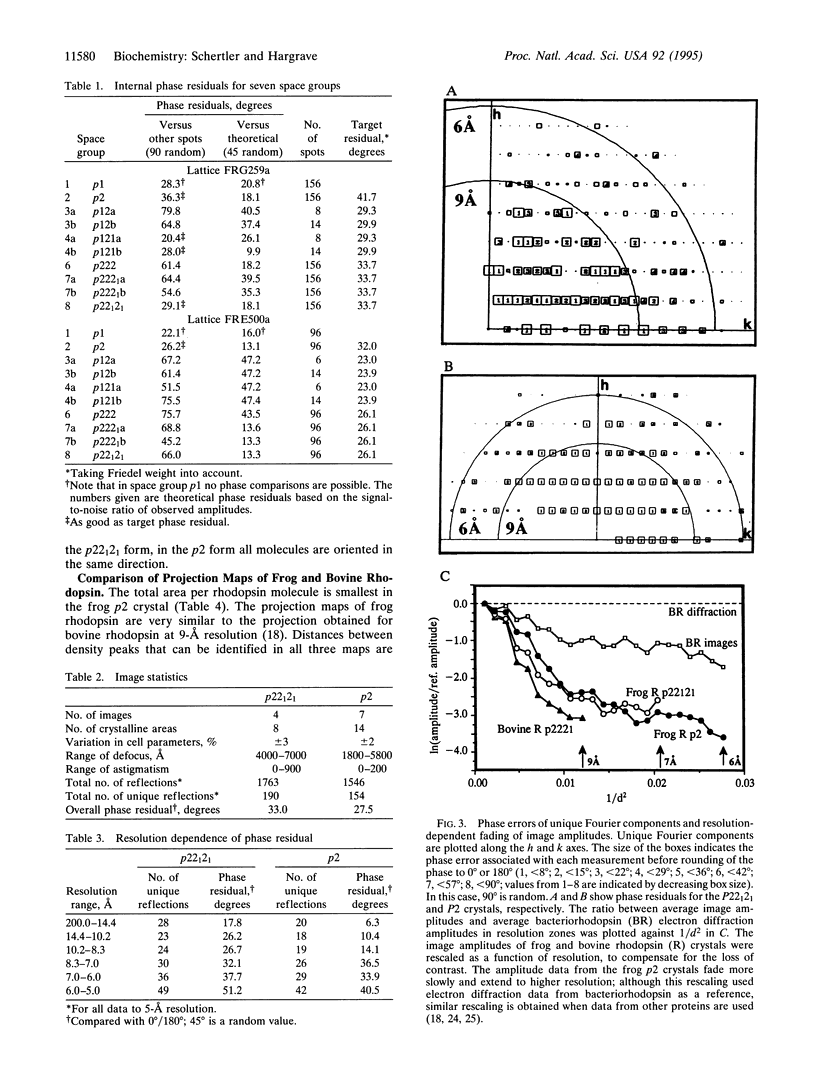
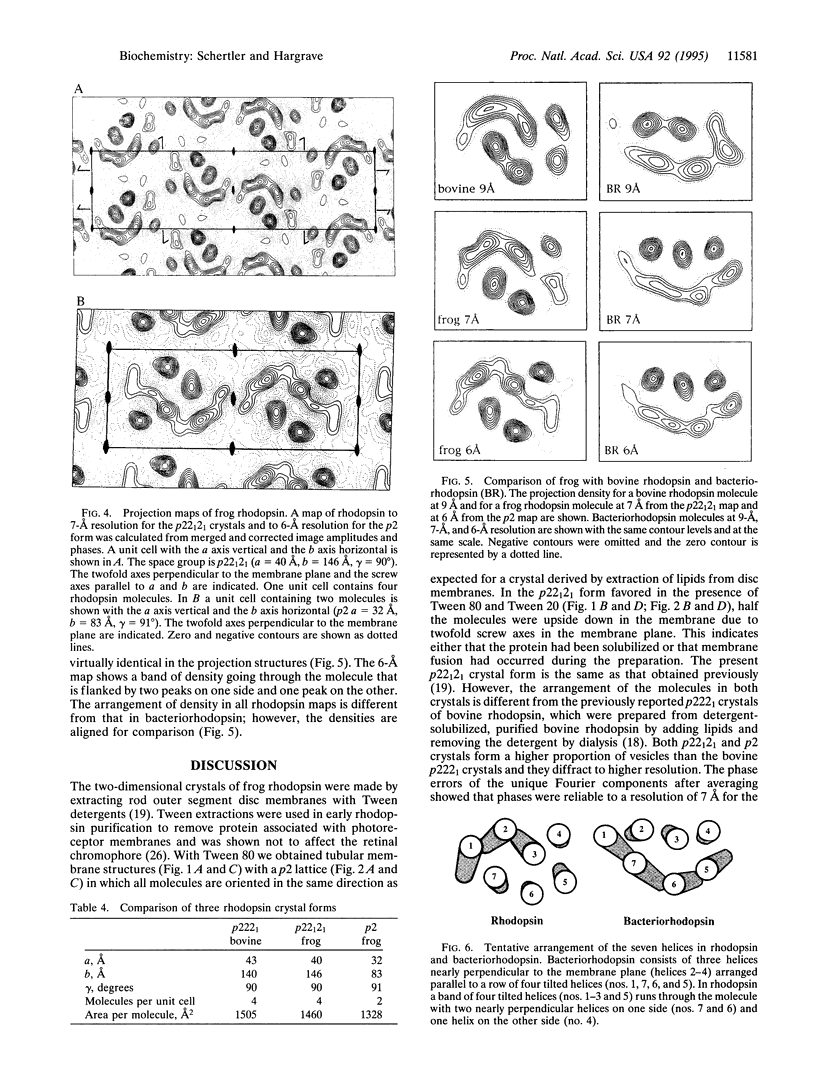
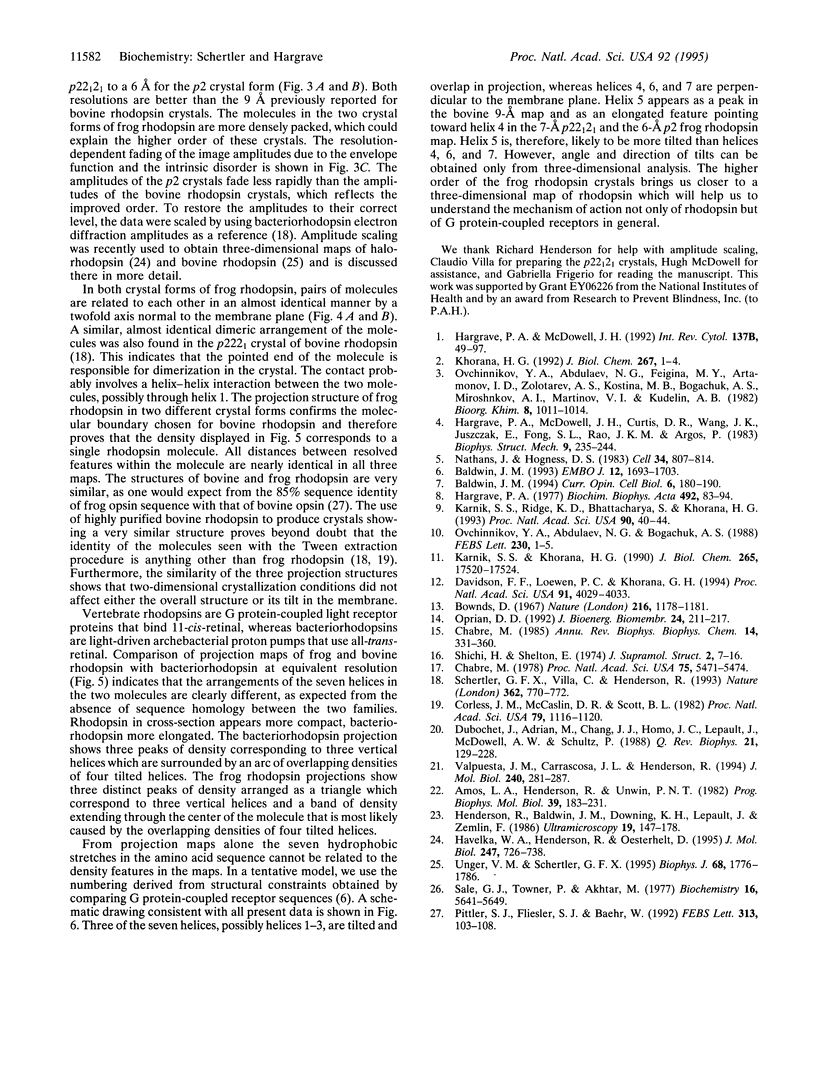
Images in this article
Selected References
These references are in PubMed. This may not be the complete list of references from this article.
- Amos L. A., Henderson R., Unwin P. N. Three-dimensional structure determination by electron microscopy of two-dimensional crystals. Prog Biophys Mol Biol. 1982;39(3):183–231. doi: 10.1016/0079-6107(83)90017-2. [DOI] [PubMed] [Google Scholar]
- Baldwin J. M. Structure and function of receptors coupled to G proteins. Curr Opin Cell Biol. 1994 Apr;6(2):180–190. doi: 10.1016/0955-0674(94)90134-1. [DOI] [PubMed] [Google Scholar]
- Baldwin J. M. The probable arrangement of the helices in G protein-coupled receptors. EMBO J. 1993 Apr;12(4):1693–1703. doi: 10.1002/j.1460-2075.1993.tb05814.x. [DOI] [PMC free article] [PubMed] [Google Scholar]
- Bownds D. Site of attachment of retinal in rhodopsin. Nature. 1967 Dec 23;216(5121):1178–1181. doi: 10.1038/2161178a0. [DOI] [PubMed] [Google Scholar]
- Chabre M. Diamagnetic anisotropy and orientation of alpha helix in frog rhodopsin and meta II intermediate. Proc Natl Acad Sci U S A. 1978 Nov;75(11):5471–5474. doi: 10.1073/pnas.75.11.5471. [DOI] [PMC free article] [PubMed] [Google Scholar]
- Chabre M. Trigger and amplification mechanisms in visual phototransduction. Annu Rev Biophys Biophys Chem. 1985;14:331–360. doi: 10.1146/annurev.bb.14.060185.001555. [DOI] [PubMed] [Google Scholar]
- Corless J. M., McCaslin D. R., Scott B. L. Two-dimensional rhodopsin crystals from disk membranes of frog retinal rod outer segments. Proc Natl Acad Sci U S A. 1982 Feb;79(4):1116–1120. doi: 10.1073/pnas.79.4.1116. [DOI] [PMC free article] [PubMed] [Google Scholar]
- Davidson F. F., Loewen P. C., Khorana H. G. Structure and function in rhodopsin: replacement by alanine of cysteine residues 110 and 187, components of a conserved disulfide bond in rhodopsin, affects the light-activated metarhodopsin II state. Proc Natl Acad Sci U S A. 1994 Apr 26;91(9):4029–4033. doi: 10.1073/pnas.91.9.4029. [DOI] [PMC free article] [PubMed] [Google Scholar]
- Dubochet J., Adrian M., Chang J. J., Homo J. C., Lepault J., McDowall A. W., Schultz P. Cryo-electron microscopy of vitrified specimens. Q Rev Biophys. 1988 May;21(2):129–228. doi: 10.1017/s0033583500004297. [DOI] [PubMed] [Google Scholar]
- Hargrave P. A., McDowell J. H., Curtis D. R., Wang J. K., Juszczak E., Fong S. L., Rao J. K., Argos P. The structure of bovine rhodopsin. Biophys Struct Mech. 1983;9(4):235–244. doi: 10.1007/BF00535659. [DOI] [PubMed] [Google Scholar]
- Hargrave P. A., McDowell J. H. Rhodopsin and phototransduction. Int Rev Cytol. 1992;137B:49–97. doi: 10.1016/s0074-7696(08)62600-5. [DOI] [PubMed] [Google Scholar]
- Hargrave P. A. The amino-terminal tryptic peptide of bovine rhodopsin. A glycopeptide containing two sites of oligosaccharide attachment. Biochim Biophys Acta. 1977 May 27;492(1):83–94. doi: 10.1016/0005-2795(77)90216-1. [DOI] [PubMed] [Google Scholar]
- Havelka W. A., Henderson R., Oesterhelt D. Three-dimensional structure of halorhodopsin at 7 A resolution. J Mol Biol. 1995 Apr 7;247(4):726–738. doi: 10.1006/jmbi.1995.0176. [DOI] [PubMed] [Google Scholar]
- Karnik S. S., Khorana H. G. Assembly of functional rhodopsin requires a disulfide bond between cysteine residues 110 and 187. J Biol Chem. 1990 Oct 15;265(29):17520–17524. [PubMed] [Google Scholar]
- Karnik S. S., Ridge K. D., Bhattacharya S., Khorana H. G. Palmitoylation of bovine opsin and its cysteine mutants in COS cells. Proc Natl Acad Sci U S A. 1993 Jan 1;90(1):40–44. doi: 10.1073/pnas.90.1.40. [DOI] [PMC free article] [PubMed] [Google Scholar]
- Khorana H. G. Rhodopsin, photoreceptor of the rod cell. An emerging pattern for structure and function. J Biol Chem. 1992 Jan 5;267(1):1–4. [PubMed] [Google Scholar]
- Nathans J., Hogness D. S. Isolation, sequence analysis, and intron-exon arrangement of the gene encoding bovine rhodopsin. Cell. 1983 Oct;34(3):807–814. doi: 10.1016/0092-8674(83)90537-8. [DOI] [PubMed] [Google Scholar]
- Oprian D. D. The ligand-binding domain of rhodopsin and other G protein-linked receptors. J Bioenerg Biomembr. 1992 Apr;24(2):211–217. doi: 10.1007/BF00762679. [DOI] [PubMed] [Google Scholar]
- Ovchinnikov YuA, Abdulaev N. G., Bogachuk A. S. Two adjacent cysteine residues in the C-terminal cytoplasmic fragment of bovine rhodopsin are palmitylated. FEBS Lett. 1988 Mar 28;230(1-2):1–5. doi: 10.1016/0014-5793(88)80628-8. [DOI] [PubMed] [Google Scholar]
- Pittler S. J., Fliesler S. J., Baehr W. Primary structure of frog rhodopsin. FEBS Lett. 1992 Nov 23;313(2):103–108. doi: 10.1016/0014-5793(92)81422-i. [DOI] [PubMed] [Google Scholar]
- Sale G. J., Towner P., Akhtar M. Functional rhodopsin complex consisting of three noncovalently linked fragments. Biochemistry. 1977 Dec 13;16(25):5641–5649. doi: 10.1021/bi00644a040. [DOI] [PubMed] [Google Scholar]
- Schertler G. F., Villa C., Henderson R. Projection structure of rhodopsin. Nature. 1993 Apr 22;362(6422):770–772. doi: 10.1038/362770a0. [DOI] [PubMed] [Google Scholar]
- Shichi H., Shelton E. Assessment of physiological integrity of sonicated retinal rod membranes. J Supramol Struct. 1974;2(1):7–16. doi: 10.1002/jss.400020103. [DOI] [PubMed] [Google Scholar]
- Unger V. M., Schertler G. F. Low resolution structure of bovine rhodopsin determined by electron cryo-microscopy. Biophys J. 1995 May;68(5):1776–1786. doi: 10.1016/S0006-3495(95)80354-1. [DOI] [PMC free article] [PubMed] [Google Scholar]
- Valpuesta J. M., Carrascosa J. L., Henderson R. Analysis of electron microscope images and electron diffraction patterns of thin crystals of phi 29 connectors in ice. J Mol Biol. 1994 Jul 22;240(4):281–287. doi: 10.1006/jmbi.1994.1445. [DOI] [PubMed] [Google Scholar]