Abstract
The mycobacterial cell wall contains large amounts of unusual lipids, including mycolic acids that are covalently linked to the underlying arabinogalactan-peptidoglycan complex. Hydrocarbon chains of much of these lipids have been shown to be packed in a direction perpendicular to the plane of the cell surface. In this study, we examined the dynamic properties of the organized lipid domains in the cell wall isolated from Mycobacterium chelonae grown at 30 degrees C. Differential scanning calorimetry showed that much of the lipids underwent major thermal transitions between 30 degree C and 65 degrees C, that is at temperatures above the growth temperature, a result suggesting that a significant portion of the lipids existed in a structure of extremely low fluidity in the growing cells. Spin-labeled fatty acid probes were successfully inserted into the more fluid part of the cell wall. Our model of the cell wall suggests that this domain corresponds to the outermost leaflet, a conclusion reinforced by the observation that labeling of intact cells produced electron spin resonance spectra similar to those of the isolated cell wall. Use of stearate labeled at different positions showed that the fluidity within the outer leaflet increased only slightly as the nitroxide group was placed farther away from the surface. These results are consistent with the model of mycobacterial cell wall containing an asymmetric lipid bilayer, with an internal, less fluid mycolic acid leaflet and an external, more fluid leaflet composed of lipids containing shorter chain fatty acids. The presence of the low-fluidity layer will lower the permeability of the cell wall to lipophilic antibiotics and chemotherapeutic agents and may contribute to the well-known intrinsic resistance of mycobacteria to such compounds.
Full text
PDF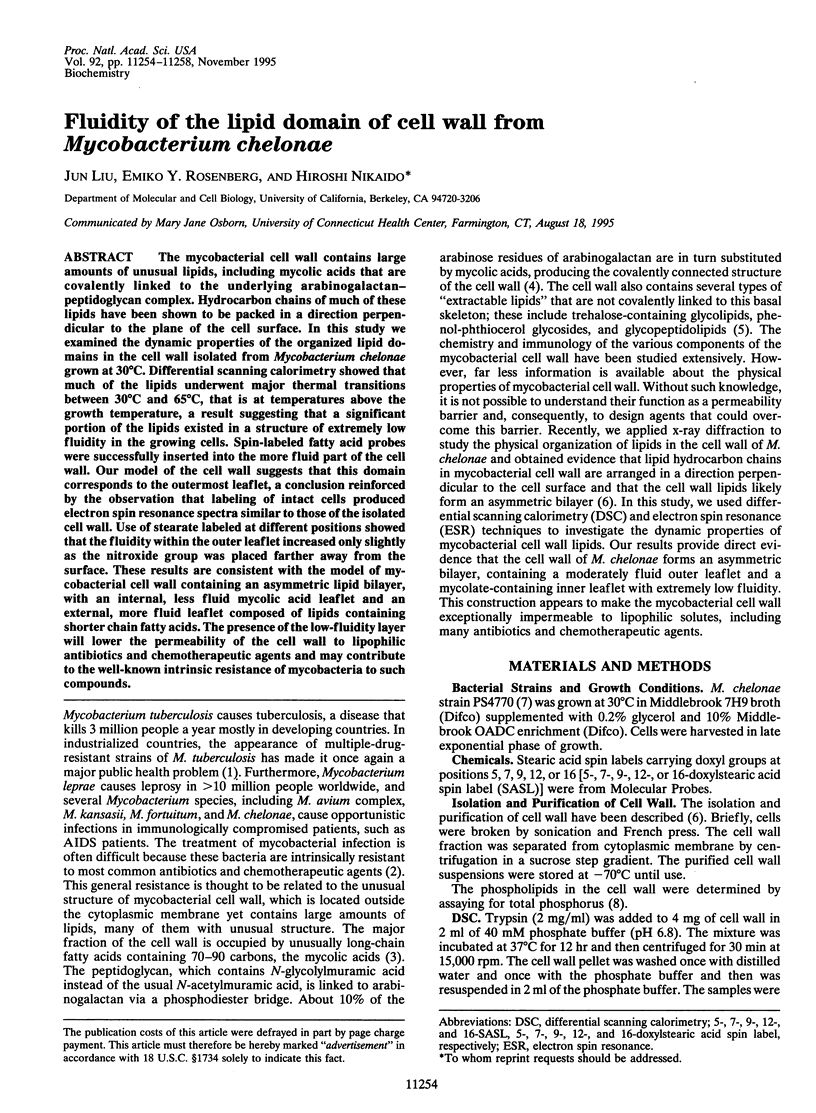
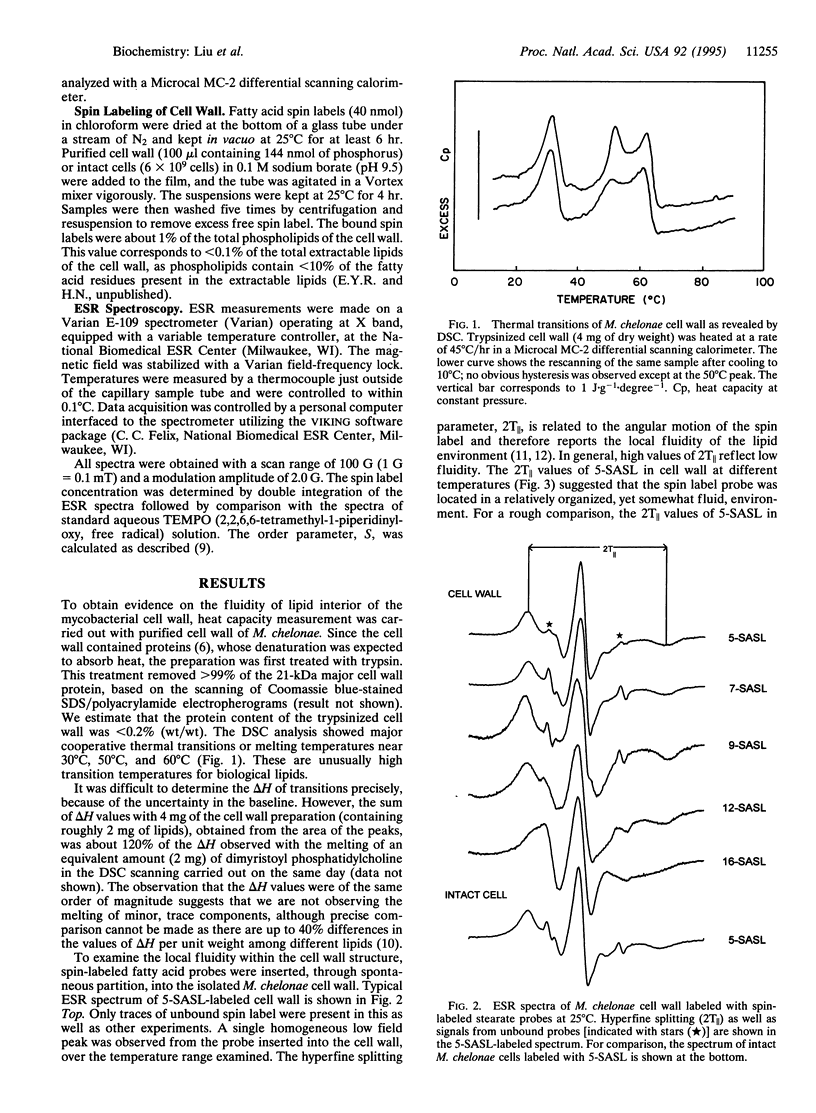
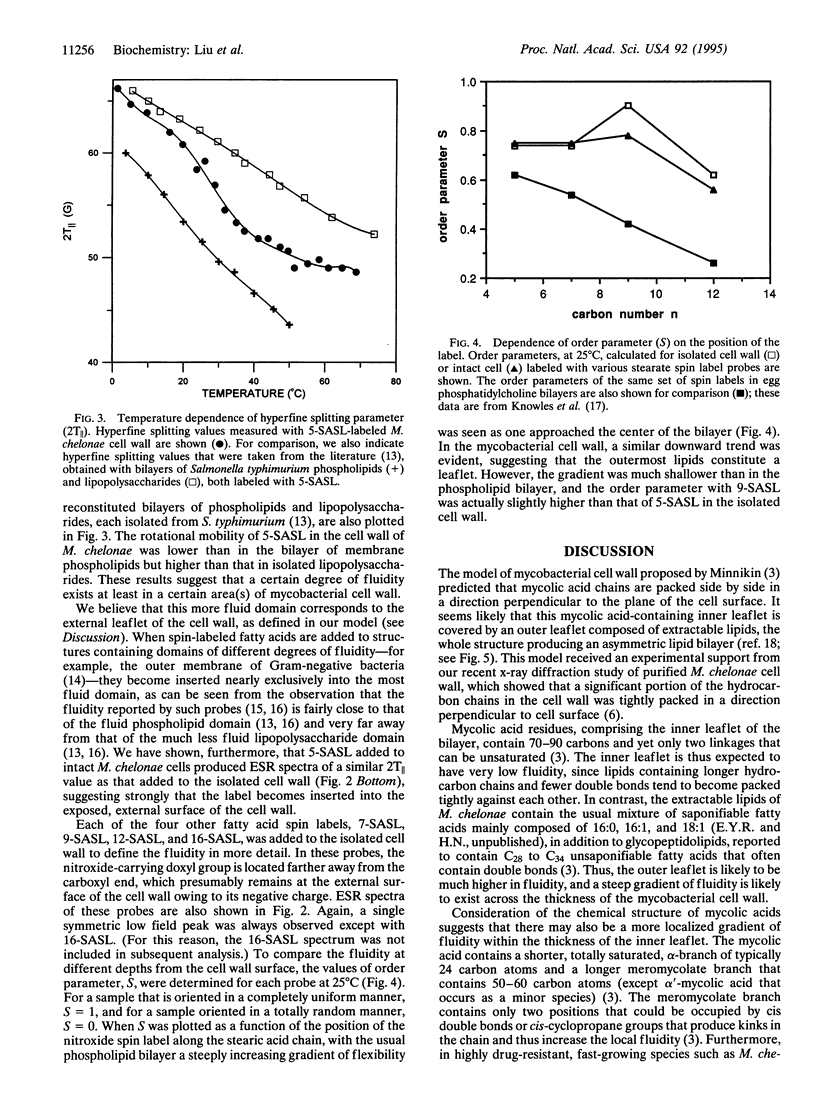
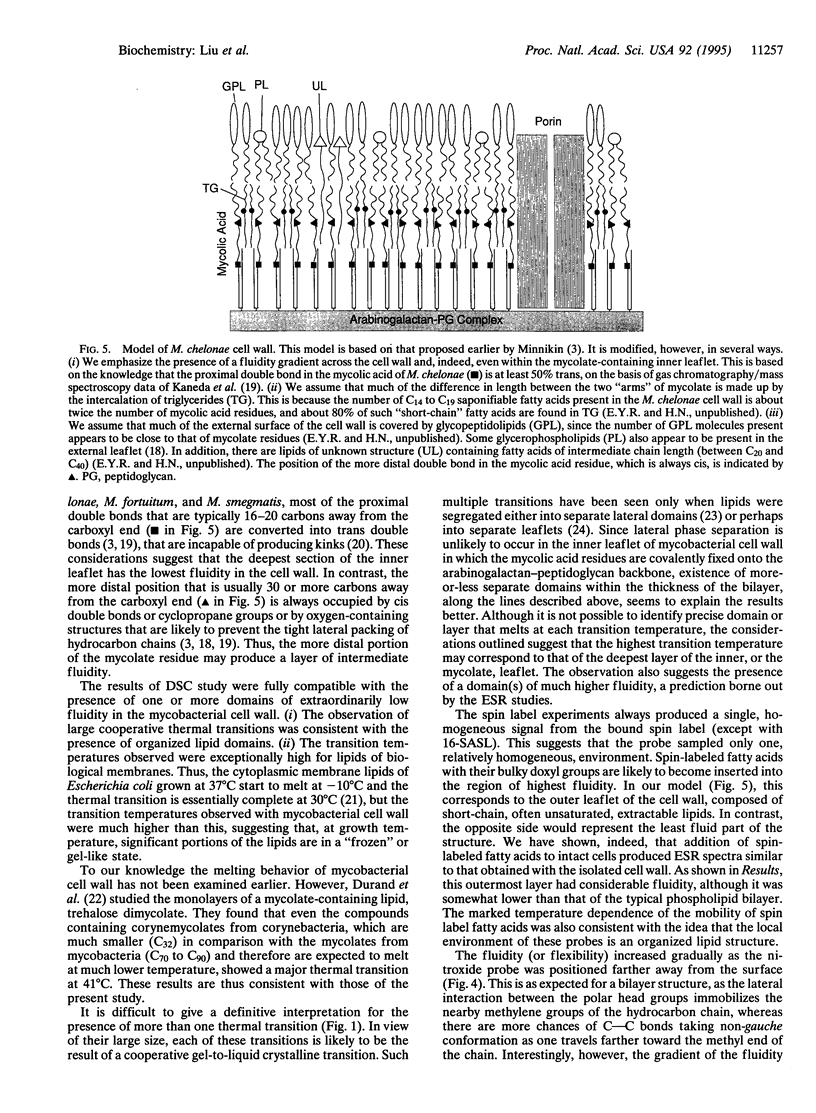
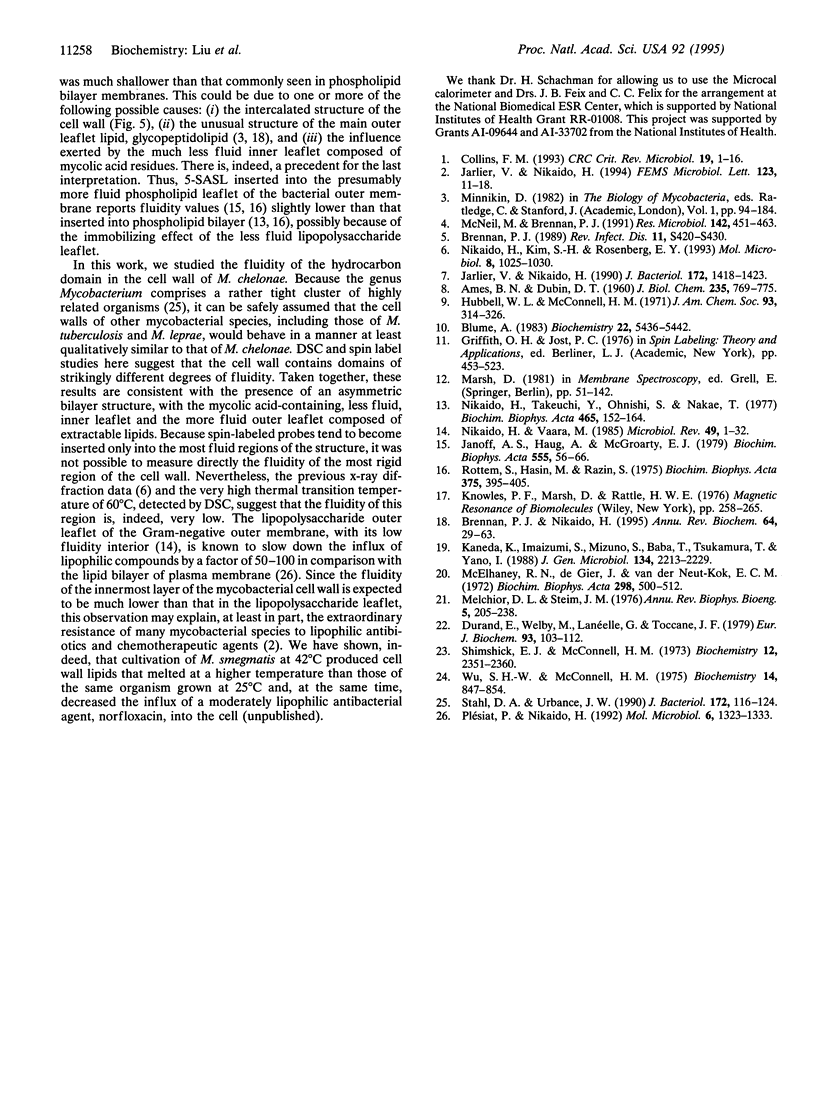
Images in this article
Selected References
These references are in PubMed. This may not be the complete list of references from this article.
- AMES B. N., DUBIN D. T. The role of polyamines in the neutralization of bacteriophage deoxyribonucleic acid. J Biol Chem. 1960 Mar;235:769–775. [PubMed] [Google Scholar]
- Brennan P. J., Nikaido H. The envelope of mycobacteria. Annu Rev Biochem. 1995;64:29–63. doi: 10.1146/annurev.bi.64.070195.000333. [DOI] [PubMed] [Google Scholar]
- Brennan P. J. Structure of mycobacteria: recent developments in defining cell wall carbohydrates and proteins. Rev Infect Dis. 1989 Mar-Apr;11 (Suppl 2):S420–S430. doi: 10.1093/clinids/11.supplement_2.s420. [DOI] [PubMed] [Google Scholar]
- Durand E., Welby M., Laneelle G., Tocanne J. F. Phase behaviour of cord factor and related bacterial glycolipid toxins. A monolayer study. Eur J Biochem. 1979 Jan 2;93(1):103–112. doi: 10.1111/j.1432-1033.1979.tb12799.x. [DOI] [PubMed] [Google Scholar]
- Hong-wei S., McConnell H. Phase separations in phospholipd membranes. Biochemistry. 1975 Feb 25;14(4):847–854. doi: 10.1021/bi00675a032. [DOI] [PubMed] [Google Scholar]
- Hubbell W. L., McConnell H. M. Molecular motion in spin-labeled phospholipids and membranes. J Am Chem Soc. 1971 Jan 27;93(2):314–326. doi: 10.1021/ja00731a005. [DOI] [PubMed] [Google Scholar]
- Janoff A. S., Haug A., McGroarty E. J. Relationship of growth temperature and thermotropic lipid phase changes in cytoplasmic and outer membranes from Escherichia coli K12. Biochim Biophys Acta. 1979 Jul 19;555(1):56–66. doi: 10.1016/0005-2736(79)90071-3. [DOI] [PubMed] [Google Scholar]
- Jarlier V., Nikaido H. Mycobacterial cell wall: structure and role in natural resistance to antibiotics. FEMS Microbiol Lett. 1994 Oct 15;123(1-2):11–18. doi: 10.1111/j.1574-6968.1994.tb07194.x. [DOI] [PubMed] [Google Scholar]
- Jarlier V., Nikaido H. Permeability barrier to hydrophilic solutes in Mycobacterium chelonei. J Bacteriol. 1990 Mar;172(3):1418–1423. doi: 10.1128/jb.172.3.1418-1423.1990. [DOI] [PMC free article] [PubMed] [Google Scholar]
- Kaneda K., Imaizumi S., Mizuno S., Baba T., Tsukamura M., Yano I. Structure and molecular species composition of three homologous series of alpha-mycolic acids from Mycobacterium spp. J Gen Microbiol. 1988 Aug;134(8):2213–2229. doi: 10.1099/00221287-134-8-2213. [DOI] [PubMed] [Google Scholar]
- Marsh D. Electron spin resonance: spin labels. Mol Biol Biochem Biophys. 1981;31:51–142. doi: 10.1007/978-3-642-81537-9_2. [DOI] [PubMed] [Google Scholar]
- McNeil M. R., Brennan P. J. Structure, function and biogenesis of the cell envelope of mycobacteria in relation to bacterial physiology, pathogenesis and drug resistance; some thoughts and possibilities arising from recent structural information. Res Microbiol. 1991 May;142(4):451–463. doi: 10.1016/0923-2508(91)90120-y. [DOI] [PubMed] [Google Scholar]
- Mcelhaney R. N., de Gier J., van der Neut-Kok E. C. The effect of alterations in fatty acid composition and cholesterol content on the nonelectrolyte permeability of Acholeplasma laidlawii B cells and derived liposomes. Biochim Biophys Acta. 1973 Mar 16;298(2):500–512. doi: 10.1016/0005-2736(73)90376-3. [DOI] [PubMed] [Google Scholar]
- Melchior D. L., Steim J. M. Thermotropic transitions in biomembranes. Annu Rev Biophys Bioeng. 1976;5:205–238. doi: 10.1146/annurev.bb.05.060176.001225. [DOI] [PubMed] [Google Scholar]
- Nikaido H., Kim S. H., Rosenberg E. Y. Physical organization of lipids in the cell wall of Mycobacterium chelonae. Mol Microbiol. 1993 Jun;8(6):1025–1030. doi: 10.1111/j.1365-2958.1993.tb01647.x. [DOI] [PubMed] [Google Scholar]
- Nikaido H., Takeuchi Y., Ohnishi S. I., Nakae T. Outer membrane of Salmonella typhimurium. Electron spin resonance studies. Biochim Biophys Acta. 1977 Feb 14;465(1):152–164. doi: 10.1016/0005-2736(77)90363-7. [DOI] [PubMed] [Google Scholar]
- Nikaido H., Vaara M. Molecular basis of bacterial outer membrane permeability. Microbiol Rev. 1985 Mar;49(1):1–32. doi: 10.1128/mr.49.1.1-32.1985. [DOI] [PMC free article] [PubMed] [Google Scholar]
- Plésiat P., Nikaido H. Outer membranes of gram-negative bacteria are permeable to steroid probes. Mol Microbiol. 1992 May;6(10):1323–1333. doi: 10.1111/j.1365-2958.1992.tb00853.x. [DOI] [PubMed] [Google Scholar]
- Rottem S., Hasin M., Razin S. The outer membrane of Proteus mirabilis. II. The extractable lipid fraction and electron-paramagnetic resonance analysis of the outer and cytoplasmic membranes. Biochim Biophys Acta. 1975 Feb 14;375(3):395–405. doi: 10.1016/0005-2736(75)90355-7. [DOI] [PubMed] [Google Scholar]
- Shimshick E. J., McConnell H. M. Lateral phase separation in phospholipid membranes. Biochemistry. 1973 Jun 5;12(12):2351–2360. doi: 10.1021/bi00736a026. [DOI] [PubMed] [Google Scholar]
- Stahl D. A., Urbance J. W. The division between fast- and slow-growing species corresponds to natural relationships among the mycobacteria. J Bacteriol. 1990 Jan;172(1):116–124. doi: 10.1128/jb.172.1.116-124.1990. [DOI] [PMC free article] [PubMed] [Google Scholar]