Abstract
Cystic fibrosis is a disease characterized by abnormalities in the epithelia of the lungs, intestine, salivary and sweat glands, liver, and reproductive systems, often as a result of inadequate hydration of their secretions. The primary defect in cystic fibrosis is the altered activity of a cAMP-activated Cl- channel, the cystic fibrosis transmembrane conductance regulator (CFTR) channel. However, it is not clear how a defect in the CFTR Cl- channel function leads to the observed pathological changes. Although much is known about the structural properties and regulation of the CFTR, little is known of its relationship to cellular functions other than the cAMP-dependent Cl- secretion. Here we report that cell volume regulation after hypotonic challenge is also defective in intestinal crypt epithelial cells isolated from CFTR -/- mutant mice. Moreover, the impairment of the regulatory volume decrease in CFTR -/- crypts appears to be related to the inability of a K+ conductance to provide a pathway for the exit of this cation during the volume adjustments. This provides evidence that the lack of CFTR protein may have additional consequences for the cellular function other than the abnormal cAMP-mediated Cl- secretion.
Full text
PDF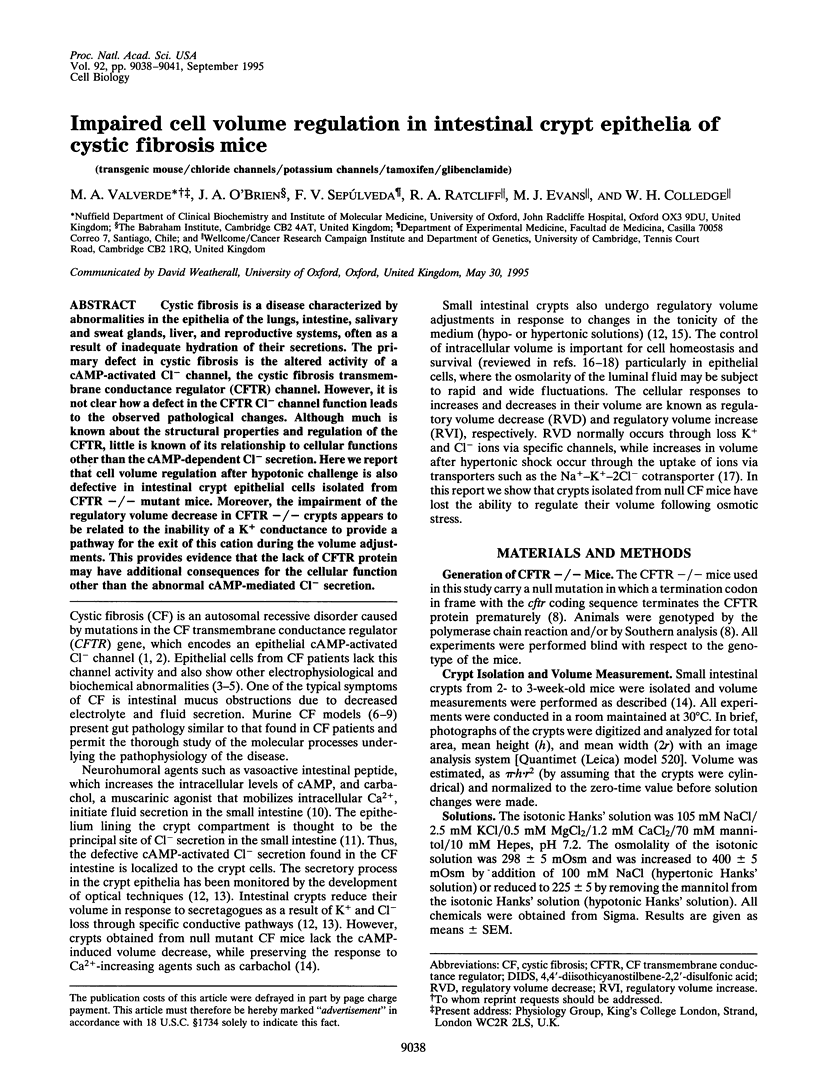
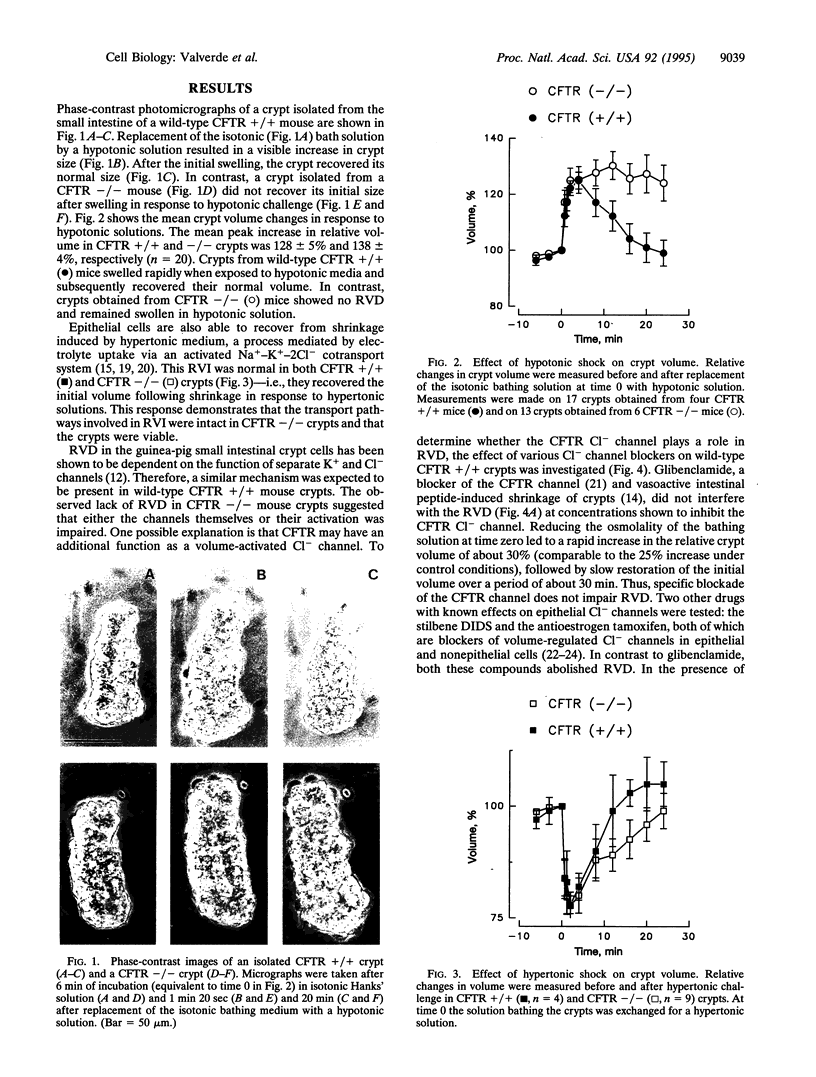
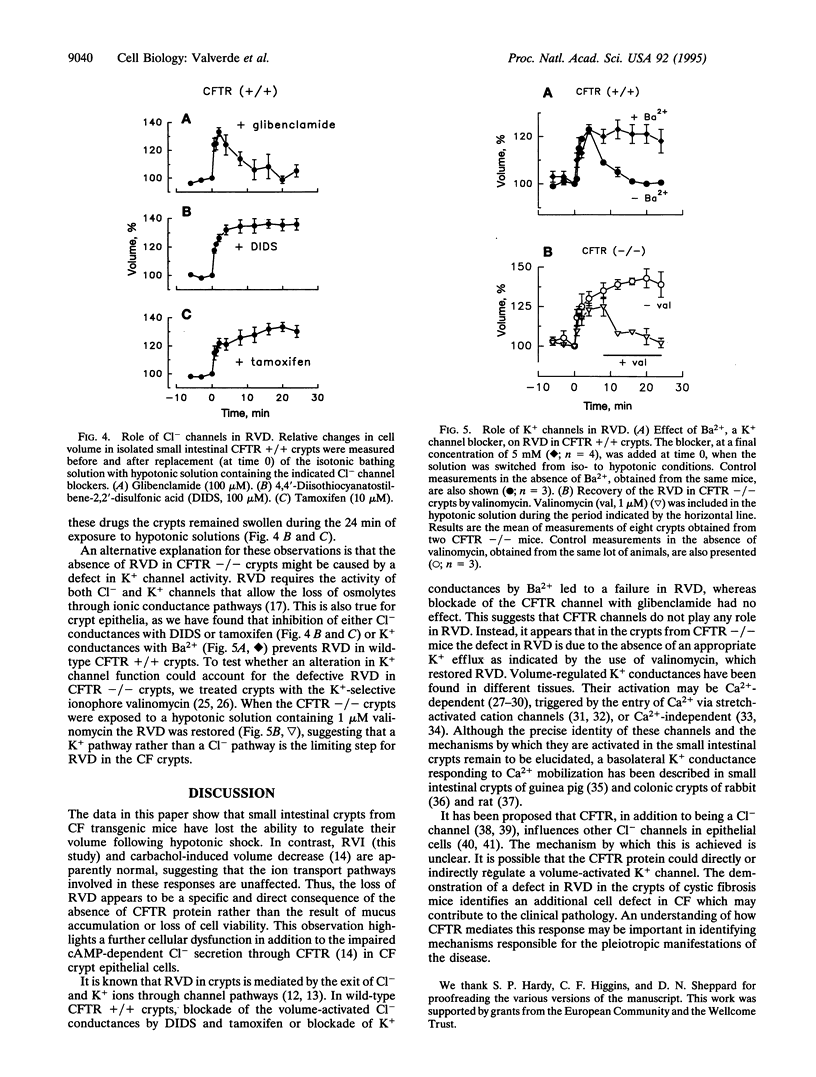
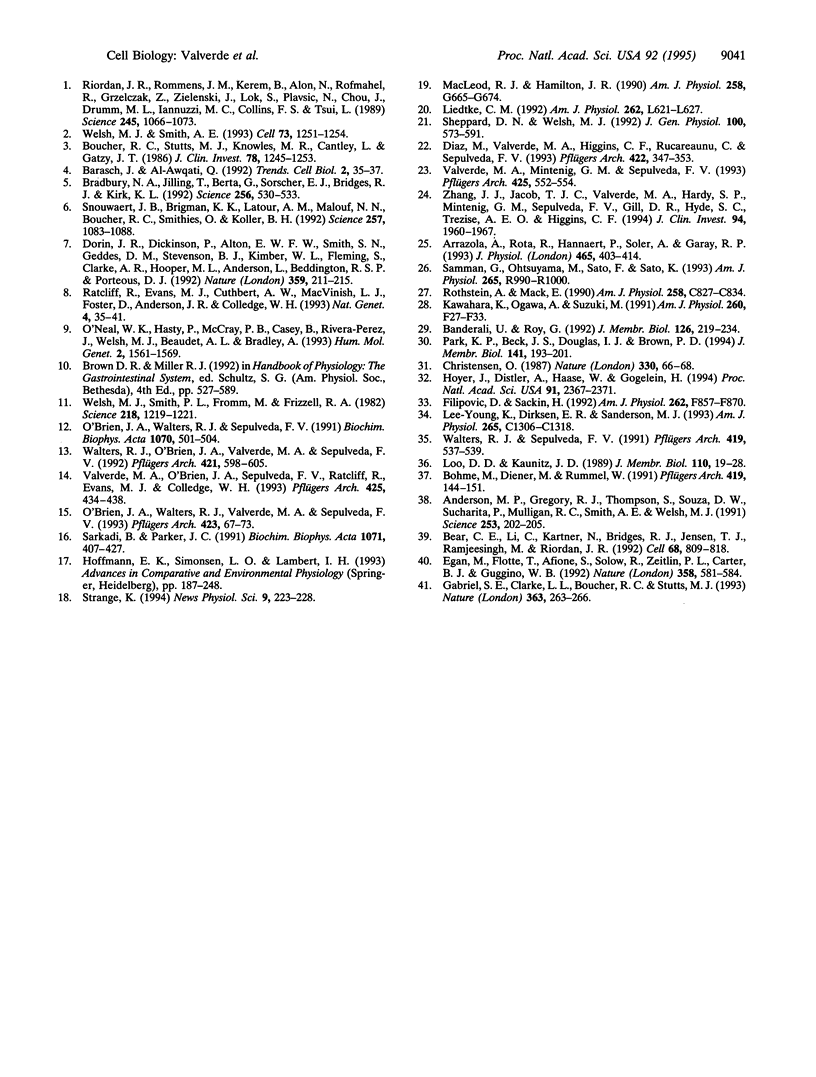
Images in this article
Selected References
These references are in PubMed. This may not be the complete list of references from this article.
- Anderson M. P., Gregory R. J., Thompson S., Souza D. W., Paul S., Mulligan R. C., Smith A. E., Welsh M. J. Demonstration that CFTR is a chloride channel by alteration of its anion selectivity. Science. 1991 Jul 12;253(5016):202–205. doi: 10.1126/science.1712984. [DOI] [PubMed] [Google Scholar]
- Arrazola A., Rota R., Hannaert P., Soler A., Garay R. P. Cell volume regulation in rat thymocytes. J Physiol. 1993 Jun;465:403–414. doi: 10.1113/jphysiol.1993.sp019683. [DOI] [PMC free article] [PubMed] [Google Scholar]
- Banderali U., Roy G. Activation of K+ and Cl- channels in MDCK cells during volume regulation in hypotonic media. J Membr Biol. 1992 Mar;126(3):219–234. doi: 10.1007/BF00232319. [DOI] [PubMed] [Google Scholar]
- Barasch J., Al-Awqati Q. Chloride channels, Golgi pH and cystic fibrosis. Trends Cell Biol. 1992 Feb;2(2):35–37. doi: 10.1016/0962-8924(92)90149-h. [DOI] [PubMed] [Google Scholar]
- Bear C. E., Li C. H., Kartner N., Bridges R. J., Jensen T. J., Ramjeesingh M., Riordan J. R. Purification and functional reconstitution of the cystic fibrosis transmembrane conductance regulator (CFTR). Cell. 1992 Feb 21;68(4):809–818. doi: 10.1016/0092-8674(92)90155-6. [DOI] [PubMed] [Google Scholar]
- Boucher R. C., Stutts M. J., Knowles M. R., Cantley L., Gatzy J. T. Na+ transport in cystic fibrosis respiratory epithelia. Abnormal basal rate and response to adenylate cyclase activation. J Clin Invest. 1986 Nov;78(5):1245–1252. doi: 10.1172/JCI112708. [DOI] [PMC free article] [PubMed] [Google Scholar]
- Bradbury N. A., Jilling T., Berta G., Sorscher E. J., Bridges R. J., Kirk K. L. Regulation of plasma membrane recycling by CFTR. Science. 1992 Apr 24;256(5056):530–532. doi: 10.1126/science.1373908. [DOI] [PubMed] [Google Scholar]
- Böhme M., Diener M., Rummel W. Calcium- and cyclic-AMP-mediated secretory responses in isolated colonic crypts. Pflugers Arch. 1991 Sep;419(2):144–151. doi: 10.1007/BF00373000. [DOI] [PubMed] [Google Scholar]
- Christensen O. Mediation of cell volume regulation by Ca2+ influx through stretch-activated channels. Nature. 1987 Nov 5;330(6143):66–68. doi: 10.1038/330066a0. [DOI] [PubMed] [Google Scholar]
- Dorin J. R., Dickinson P., Alton E. W., Smith S. N., Geddes D. M., Stevenson B. J., Kimber W. L., Fleming S., Clarke A. R., Hooper M. L. Cystic fibrosis in the mouse by targeted insertional mutagenesis. Nature. 1992 Sep 17;359(6392):211–215. doi: 10.1038/359211a0. [DOI] [PubMed] [Google Scholar]
- Díaz M., Valverde M. A., Higgins C. F., Rucăreanu C., Sepúlveda F. V. Volume-activated chloride channels in HeLa cells are blocked by verapamil and dideoxyforskolin. Pflugers Arch. 1993 Jan;422(4):347–353. doi: 10.1007/BF00374290. [DOI] [PubMed] [Google Scholar]
- Egan M., Flotte T., Afione S., Solow R., Zeitlin P. L., Carter B. J., Guggino W. B. Defective regulation of outwardly rectifying Cl- channels by protein kinase A corrected by insertion of CFTR. Nature. 1992 Aug 13;358(6387):581–584. doi: 10.1038/358581a0. [DOI] [PubMed] [Google Scholar]
- Filipovic D., Sackin H. Stretch- and volume-activated channels in isolated proximal tubule cells. Am J Physiol. 1992 May;262(5 Pt 2):F857–F870. doi: 10.1152/ajprenal.1992.262.5.F857. [DOI] [PubMed] [Google Scholar]
- Gabriel S. E., Clarke L. L., Boucher R. C., Stutts M. J. CFTR and outward rectifying chloride channels are distinct proteins with a regulatory relationship. Nature. 1993 May 20;363(6426):263–268. doi: 10.1038/363263a0. [DOI] [PubMed] [Google Scholar]
- Hoyer J., Distler A., Haase W., Gögelein H. Ca2+ influx through stretch-activated cation channels activates maxi K+ channels in porcine endocardial endothelium. Proc Natl Acad Sci U S A. 1994 Mar 15;91(6):2367–2371. doi: 10.1073/pnas.91.6.2367. [DOI] [PMC free article] [PubMed] [Google Scholar]
- Kawahara K., Ogawa A., Suzuki M. Hyposmotic activation of Ca-activated K channels in cultured rabbit kidney proximal tubule cells. Am J Physiol. 1991 Jan;260(1 Pt 2):F27–F33. doi: 10.1152/ajprenal.1991.260.1.F27. [DOI] [PubMed] [Google Scholar]
- Kim Y. K., Dirksen E. R., Sanderson M. J. Stretch-activated channels in airway epithelial cells. Am J Physiol. 1993 Nov;265(5 Pt 1):C1306–C1318. doi: 10.1152/ajpcell.1993.265.5.C1306. [DOI] [PubMed] [Google Scholar]
- Liedtke C. M. Bumetanide-sensitive NaCl uptake in rabbit tracheal epithelial cells is stimulated by neurohormones and hypertonicity. Am J Physiol. 1992 May;262(5 Pt 1):L621–L627. doi: 10.1152/ajplung.1992.262.5.L621. [DOI] [PubMed] [Google Scholar]
- Loo D. D., Kaunitz J. D. Ca2+ and cAMP activate K+ channels in the basolateral membrane of crypt cells isolated from rabbit distal colon. J Membr Biol. 1989 Aug;110(1):19–28. doi: 10.1007/BF01870989. [DOI] [PubMed] [Google Scholar]
- MacLeod R. J., Hamilton J. R. Regulatory volume increase in mammalian jejunal villus cells is due to bumetanide-sensitive NaKCl2 cotransport. Am J Physiol. 1990 May;258(5 Pt 1):G665–G674. doi: 10.1152/ajpgi.1990.258.5.G665. [DOI] [PubMed] [Google Scholar]
- O'Brien J. A., Walters R. J., Sepúlveda F. V. Regulatory volume decrease in small intestinal crypts is inhibited by K+ and Cl- channel blockers. Biochim Biophys Acta. 1991 Dec 9;1070(2):501–504. doi: 10.1016/0005-2736(91)90093-n. [DOI] [PubMed] [Google Scholar]
- O'Brien J. A., Walters R. J., Valverde M. A., Sepúlveda F. V. Regulatory volume increase after hypertonicity- or vasoactive-intestinal-peptide-induced cell-volume decrease in small-intestinal crypts is dependent on Na(+)-K(+)-2Cl- cotransport. Pflugers Arch. 1993 Apr;423(1-2):67–73. doi: 10.1007/BF00374962. [DOI] [PubMed] [Google Scholar]
- O'Neal W. K., Hasty P., McCray P. B., Jr, Casey B., Rivera-Pérez J., Welsh M. J., Beaudet A. L., Bradley A. A severe phenotype in mice with a duplication of exon 3 in the cystic fibrosis locus. Hum Mol Genet. 1993 Oct;2(10):1561–1569. doi: 10.1093/hmg/2.10.1561. [DOI] [PubMed] [Google Scholar]
- Park K. P., Beck J. S., Douglas I. J., Brown P. D. Ca(2+)-activated K+ channels are involved in regulatory volume decrease in acinar cells isolated from the rat lacrimal gland. J Membr Biol. 1994 Aug;141(2):193–201. doi: 10.1007/BF00238253. [DOI] [PubMed] [Google Scholar]
- Ratcliff R., Evans M. J., Cuthbert A. W., MacVinish L. J., Foster D., Anderson J. R., Colledge W. H. Production of a severe cystic fibrosis mutation in mice by gene targeting. Nat Genet. 1993 May;4(1):35–41. doi: 10.1038/ng0593-35. [DOI] [PubMed] [Google Scholar]
- Riordan J. R., Rommens J. M., Kerem B., Alon N., Rozmahel R., Grzelczak Z., Zielenski J., Lok S., Plavsic N., Chou J. L. Identification of the cystic fibrosis gene: cloning and characterization of complementary DNA. Science. 1989 Sep 8;245(4922):1066–1073. doi: 10.1126/science.2475911. [DOI] [PubMed] [Google Scholar]
- Rothstein A., Mack E. Volume-activated K+ and Cl- pathways of dissociated epithelial cells (MDCK): role of Ca2+. Am J Physiol. 1990 May;258(5 Pt 1):C827–C834. doi: 10.1152/ajpcell.1990.258.5.C827. [DOI] [PubMed] [Google Scholar]
- Samman G., Ohtsuyama M., Sato F., Sato K. Volume-activated K+ and Cl- pathways of dissociated eccrine clear cells. Am J Physiol. 1993 Nov;265(5 Pt 2):R990–1000. doi: 10.1152/ajpregu.1993.265.5.R990. [DOI] [PubMed] [Google Scholar]
- Sarkadi B., Parker J. C. Activation of ion transport pathways by changes in cell volume. Biochim Biophys Acta. 1991 Dec 12;1071(4):407–427. doi: 10.1016/0304-4157(91)90005-h. [DOI] [PubMed] [Google Scholar]
- Sheppard D. N., Welsh M. J. Effect of ATP-sensitive K+ channel regulators on cystic fibrosis transmembrane conductance regulator chloride currents. J Gen Physiol. 1992 Oct;100(4):573–591. doi: 10.1085/jgp.100.4.573. [DOI] [PMC free article] [PubMed] [Google Scholar]
- Snouwaert J. N., Brigman K. K., Latour A. M., Malouf N. N., Boucher R. C., Smithies O., Koller B. H. An animal model for cystic fibrosis made by gene targeting. Science. 1992 Aug 21;257(5073):1083–1088. doi: 10.1126/science.257.5073.1083. [DOI] [PubMed] [Google Scholar]
- Valverde M. A., Mintenig G. M., Sepúlveda F. V. Differential effects of tamoxifen and I- on three distinguishable chloride currents activated in T84 intestinal cells. Pflugers Arch. 1993 Dec;425(5-6):552–554. doi: 10.1007/BF00374885. [DOI] [PubMed] [Google Scholar]
- Valverde M. A., O'Brien J. A., Sepúlveda F. V., Ratcliff R., Evans M. J., Colledge W. H. Inactivation of the murine cftr gene abolishes cAMP-mediated but not Ca(2+)-mediated secretagogue-induced volume decrease in small-intestinal crypts. Pflugers Arch. 1993 Dec;425(5-6):434–438. doi: 10.1007/BF00374869. [DOI] [PubMed] [Google Scholar]
- Walters R. J., O'Brien J. A., Valverde M. A., Sepúlveda F. V. Membrane conductance and cell volume changes evoked by vasoactive intestinal polypeptide and carbachol in small intestinal crypts. Pflugers Arch. 1992 Sep;421(6):598–605. doi: 10.1007/BF00375057. [DOI] [PubMed] [Google Scholar]
- Walters R. J., Sepúlveda F. V. A basolateral K+ conductance modulated by carbachol dominates the membrane potential of small intestinal crypts. Pflugers Arch. 1991 Nov;419(5):537–539. doi: 10.1007/BF00370802. [DOI] [PubMed] [Google Scholar]
- Welsh M. J., Smith A. E. Molecular mechanisms of CFTR chloride channel dysfunction in cystic fibrosis. Cell. 1993 Jul 2;73(7):1251–1254. doi: 10.1016/0092-8674(93)90353-r. [DOI] [PubMed] [Google Scholar]
- Welsh M. J., Smith P. L., Fromm M., Frizzell R. A. Crypts are the site of intestinal fluid and electrolyte secretion. Science. 1982 Dec 17;218(4578):1219–1221. doi: 10.1126/science.6293054. [DOI] [PubMed] [Google Scholar]