Abstract
Voltage-gated channel proteins sense a change in the transmembrane electric field and respond with a conformational change that allows ions to diffuse across the pore-forming structure. Site-specific mutagenesis combined with electrophysiological analysis of expressed mutants in amphibian oocytes has previously established the S4 transmembrane segment as an element of the voltage sensor. Here, we show that mutations of conserved negatively charged residues in S2 and S3 of a brain K+ channel, thought of as countercharges for the positively charged residues in S4, selectively modulate channel gating without modifying the permeation properties. Mutations of Glu235 in S2 that neutralize or reverse charge increase the probability of channel opening and the apparent gating valence. In contrast, replacements of Glu272 by Arg or Thr268 by Asp in S3 decrease the open probability and the apparent gating valence. Residue Glu225 in S2 tolerated replacement only by acidic residues, whereas Asp258 in S3 was intolerant to any attempted change. These results imply that S2 and S3 are unlikely to be involved in channel lining, yet, together with S4, may be additional components of the voltage-sensing structure.
Full text
PDF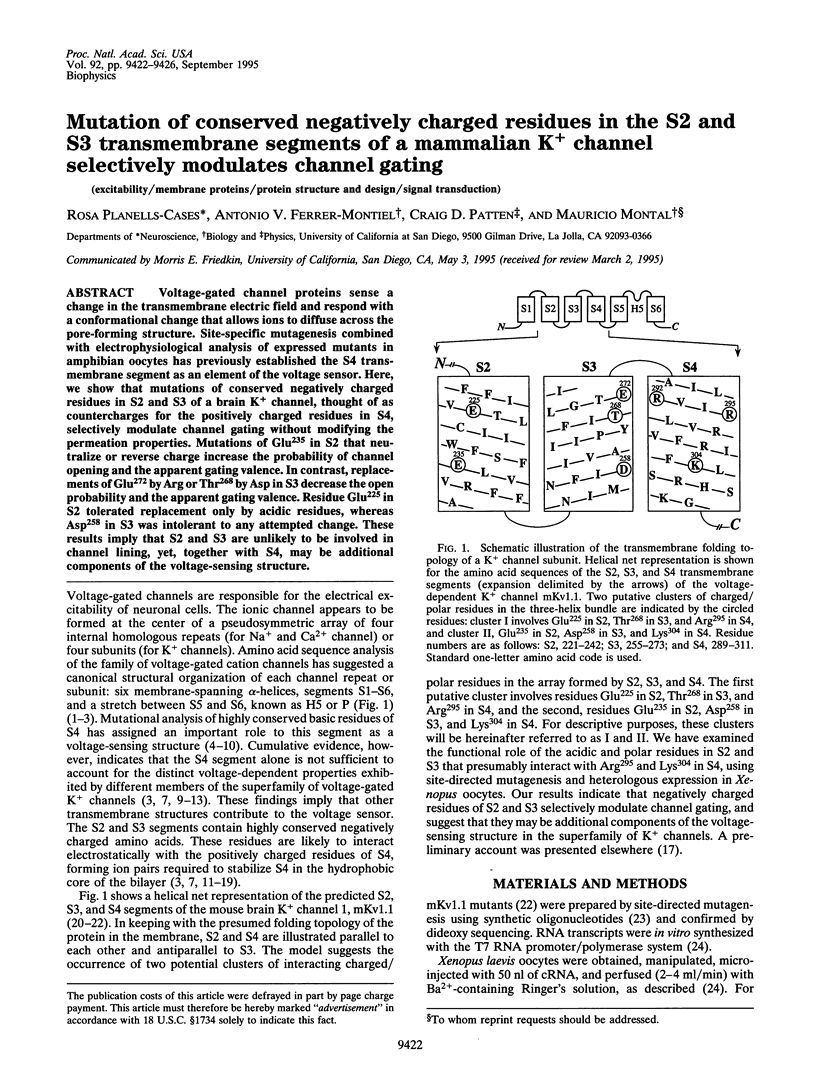
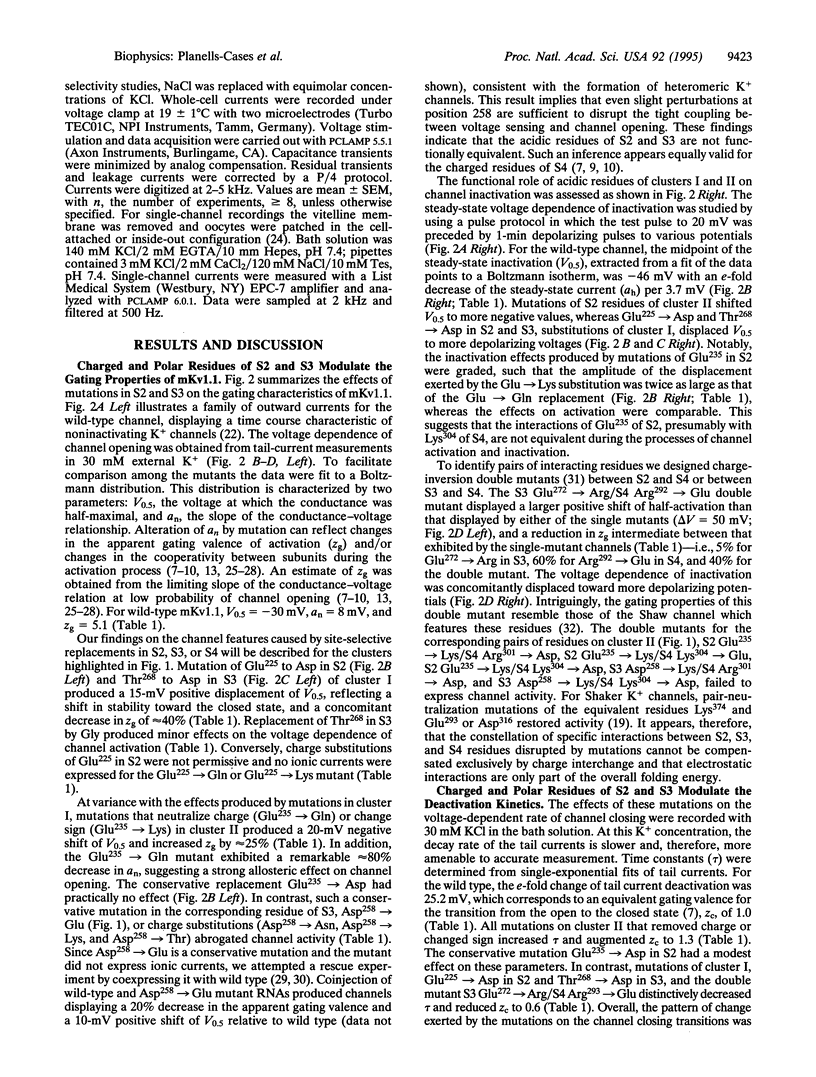
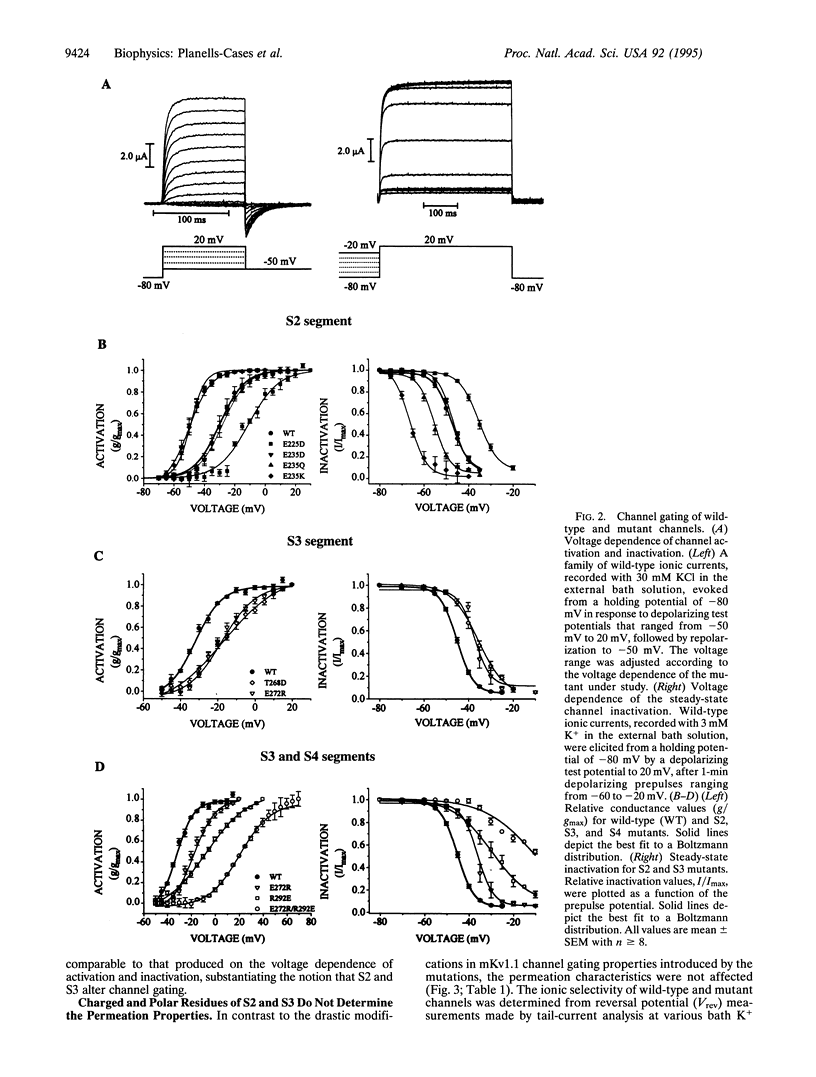
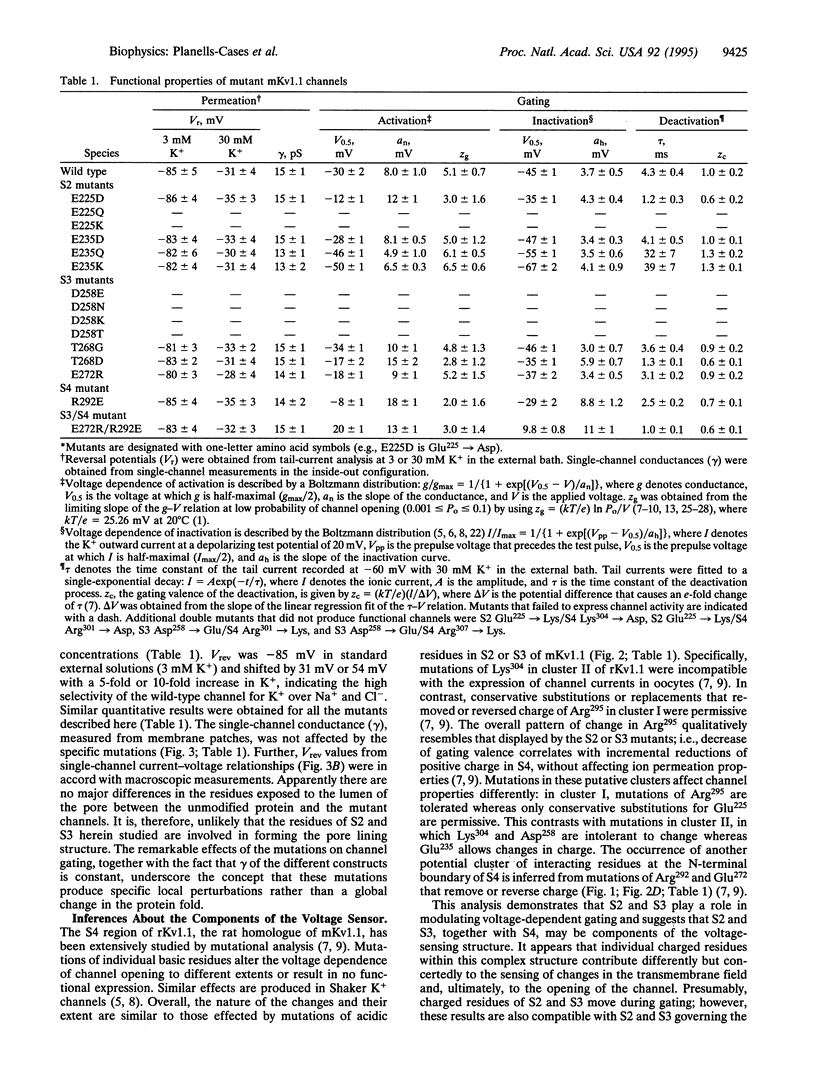
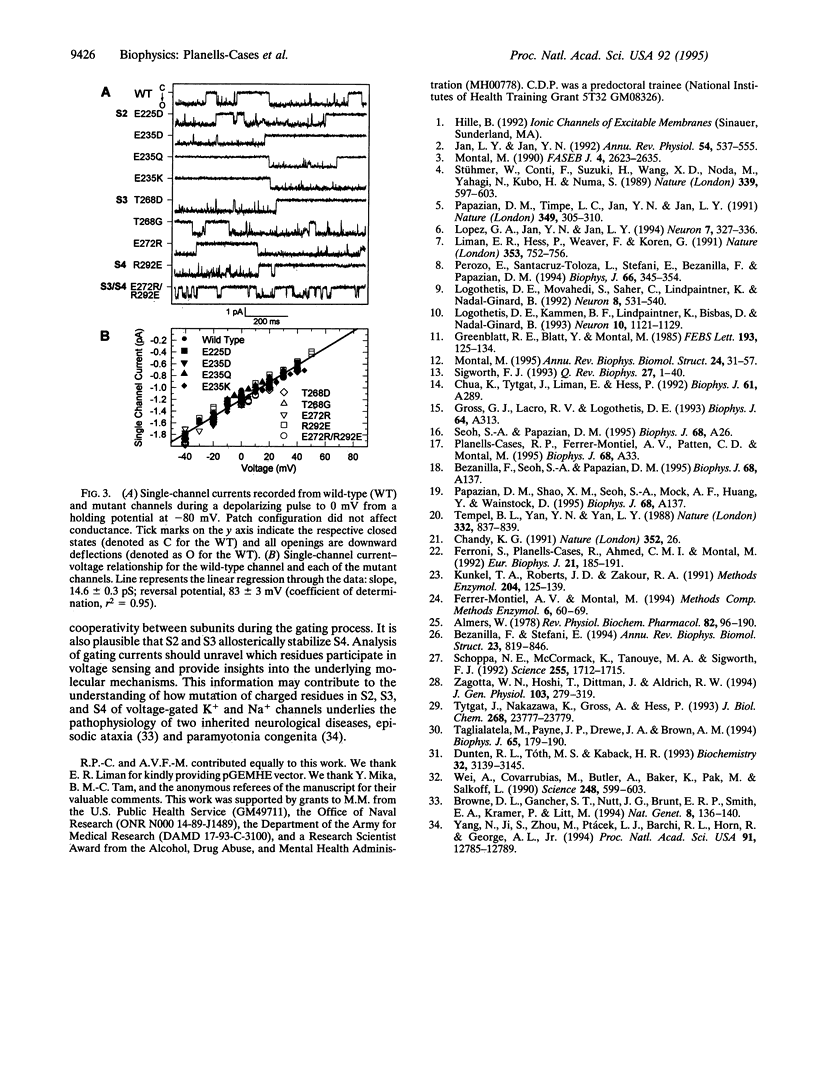
Selected References
These references are in PubMed. This may not be the complete list of references from this article.
- Almers W. Gating currents and charge movements in excitable membranes. Rev Physiol Biochem Pharmacol. 1978;82:96–190. doi: 10.1007/BFb0030498. [DOI] [PubMed] [Google Scholar]
- Bezanilla F., Stefani E. Voltage-dependent gating of ionic channels. Annu Rev Biophys Biomol Struct. 1994;23:819–846. doi: 10.1146/annurev.bb.23.060194.004131. [DOI] [PubMed] [Google Scholar]
- Browne D. L., Gancher S. T., Nutt J. G., Brunt E. R., Smith E. A., Kramer P., Litt M. Episodic ataxia/myokymia syndrome is associated with point mutations in the human potassium channel gene, KCNA1. Nat Genet. 1994 Oct;8(2):136–140. doi: 10.1038/ng1094-136. [DOI] [PubMed] [Google Scholar]
- Chandy K. G. Simplified gene nomenclature. Nature. 1991 Jul 4;352(6330):26–26. doi: 10.1038/352026b0. [DOI] [PubMed] [Google Scholar]
- Dunten R. L., Sahin-Tóth M., Kaback H. R. Role of the charge pair aspartic acid-237-lysine-358 in the lactose permease of Escherichia coli. Biochemistry. 1993 Mar 30;32(12):3139–3145. doi: 10.1021/bi00063a028. [DOI] [PubMed] [Google Scholar]
- Ferroni S., Planells-Cases R., Ahmed C. M., Montal M. Expression of a genomic clone encoding a brain potassium channel in mammalian cells using lipofection. Eur Biophys J. 1992;21(3):185–191. doi: 10.1007/BF00196762. [DOI] [PubMed] [Google Scholar]
- Greenblatt R. E., Blatt Y., Montal M. The structure of the voltage-sensitive sodium channel. Inferences derived from computer-aided analysis of the Electrophorus electricus channel primary structure. FEBS Lett. 1985 Dec 2;193(2):125–134. doi: 10.1016/0014-5793(85)80136-8. [DOI] [PubMed] [Google Scholar]
- Jan L. Y., Jan Y. N. Structural elements involved in specific K+ channel functions. Annu Rev Physiol. 1992;54:537–555. doi: 10.1146/annurev.ph.54.030192.002541. [DOI] [PubMed] [Google Scholar]
- Kunkel T. A., Bebenek K., McClary J. Efficient site-directed mutagenesis using uracil-containing DNA. Methods Enzymol. 1991;204:125–139. doi: 10.1016/0076-6879(91)04008-c. [DOI] [PubMed] [Google Scholar]
- Liman E. R., Hess P., Weaver F., Koren G. Voltage-sensing residues in the S4 region of a mammalian K+ channel. Nature. 1991 Oct 24;353(6346):752–756. doi: 10.1038/353752a0. [DOI] [PubMed] [Google Scholar]
- Logothetis D. E., Kammen B. F., Lindpaintner K., Bisbas D., Nadal-Ginard B. Gating charge differences between two voltage-gated K+ channels are due to the specific charge content of their respective S4 regions. Neuron. 1993 Jun;10(6):1121–1129. doi: 10.1016/0896-6273(93)90060-5. [DOI] [PubMed] [Google Scholar]
- Logothetis D. E., Movahedi S., Satler C., Lindpaintner K., Nadal-Ginard B. Incremental reductions of positive charge within the S4 region of a voltage-gated K+ channel result in corresponding decreases in gating charge. Neuron. 1992 Mar;8(3):531–540. doi: 10.1016/0896-6273(92)90281-h. [DOI] [PubMed] [Google Scholar]
- Lopez G. A., Jan Y. N., Jan L. Y. Hydrophobic substitution mutations in the S4 sequence alter voltage-dependent gating in Shaker K+ channels. Neuron. 1991 Aug;7(2):327–336. doi: 10.1016/0896-6273(91)90271-z. [DOI] [PubMed] [Google Scholar]
- Montal M. Design of molecular function: channels of communication. Annu Rev Biophys Biomol Struct. 1995;24:31–57. doi: 10.1146/annurev.bb.24.060195.000335. [DOI] [PubMed] [Google Scholar]
- Montal M. Molecular anatomy and molecular design of channel proteins. FASEB J. 1990 Jun;4(9):2623–2635. doi: 10.1096/fasebj.4.9.1693348. [DOI] [PubMed] [Google Scholar]
- Papazian D. M., Timpe L. C., Jan Y. N., Jan L. Y. Alteration of voltage-dependence of Shaker potassium channel by mutations in the S4 sequence. Nature. 1991 Jan 24;349(6307):305–310. doi: 10.1038/349305a0. [DOI] [PubMed] [Google Scholar]
- Perozo E., Santacruz-Toloza L., Stefani E., Bezanilla F., Papazian D. M. S4 mutations alter gating currents of Shaker K channels. Biophys J. 1994 Feb;66(2 Pt 1):345–354. doi: 10.1016/s0006-3495(94)80783-0. [DOI] [PMC free article] [PubMed] [Google Scholar]
- Schoppa N. E., McCormack K., Tanouye M. A., Sigworth F. J. The size of gating charge in wild-type and mutant Shaker potassium channels. Science. 1992 Mar 27;255(5052):1712–1715. doi: 10.1126/science.1553560. [DOI] [PubMed] [Google Scholar]
- Sigworth F. J. Voltage gating of ion channels. Q Rev Biophys. 1994 Feb;27(1):1–40. doi: 10.1017/s0033583500002894. [DOI] [PubMed] [Google Scholar]
- Stühmer W., Conti F., Suzuki H., Wang X. D., Noda M., Yahagi N., Kubo H., Numa S. Structural parts involved in activation and inactivation of the sodium channel. Nature. 1989 Jun 22;339(6226):597–603. doi: 10.1038/339597a0. [DOI] [PubMed] [Google Scholar]
- Taglialatela M., Payne J. P., Drewe J. A., Brown A. M. Rescue of lethal subunits into functional K+ channels. Biophys J. 1994 Jan;66(1):179–190. doi: 10.1016/S0006-3495(94)80755-6. [DOI] [PMC free article] [PubMed] [Google Scholar]
- Tempel B. L., Jan Y. N., Jan L. Y. Cloning of a probable potassium channel gene from mouse brain. Nature. 1988 Apr 28;332(6167):837–839. doi: 10.1038/332837a0. [DOI] [PubMed] [Google Scholar]
- Tytgat J., Nakazawa K., Gross A., Hess P. Pursuing the voltage sensor of a voltage-gated mammalian potassium channel. J Biol Chem. 1993 Nov 15;268(32):23777–23779. [PubMed] [Google Scholar]
- Wei A., Covarrubias M., Butler A., Baker K., Pak M., Salkoff L. K+ current diversity is produced by an extended gene family conserved in Drosophila and mouse. Science. 1990 May 4;248(4955):599–603. doi: 10.1126/science.2333511. [DOI] [PubMed] [Google Scholar]
- Yang N., Ji S., Zhou M., Ptácek L. J., Barchi R. L., Horn R., George A. L., Jr Sodium channel mutations in paramyotonia congenita exhibit similar biophysical phenotypes in vitro. Proc Natl Acad Sci U S A. 1994 Dec 20;91(26):12785–12789. doi: 10.1073/pnas.91.26.12785. [DOI] [PMC free article] [PubMed] [Google Scholar]
- Zagotta W. N., Hoshi T., Dittman J., Aldrich R. W. Shaker potassium channel gating. II: Transitions in the activation pathway. J Gen Physiol. 1994 Feb;103(2):279–319. doi: 10.1085/jgp.103.2.279. [DOI] [PMC free article] [PubMed] [Google Scholar]