Abstract
A hierarchy of enzyme-catalyzed positive feedback loops is examined by mathematical and numerical analysis. Four systems are described, from the simplest, in which an enzyme catalyzes its own formation from an inactive precursor, to the most complex, in which two sequential feedback loops act in a cascade. In the latter we also examine the function of a long-range feedback, in which the final enzyme produced in the second loop activates the initial step in the first loop. When the enzymes generated are subject to inhibition or inactivation, all four systems exhibit threshold properties akin to excitable systems like neuron firing. For those that are amenable to mathematical analysis, expressions are derived that relate the excitation threshold to the kinetics of enzyme generation and inhibition and the initial conditions. For the most complex system, it was expedient to employ numerical simulation to demonstrate threshold behavior, and in this case long-range feedback was seen to have two distinct effects. At sufficiently high catalytic rates, this feedback is capable of exciting an otherwise subthreshold system. At lower catalytic rates, where the long-range feedback does not significantly affect the threshold, it nonetheless has a major effect in potentiating the response above the threshold. In particular, oscillatory behavior observed in simulations of sequential feedback loops is abolished when a long-range feedback is present.
Full text
PDF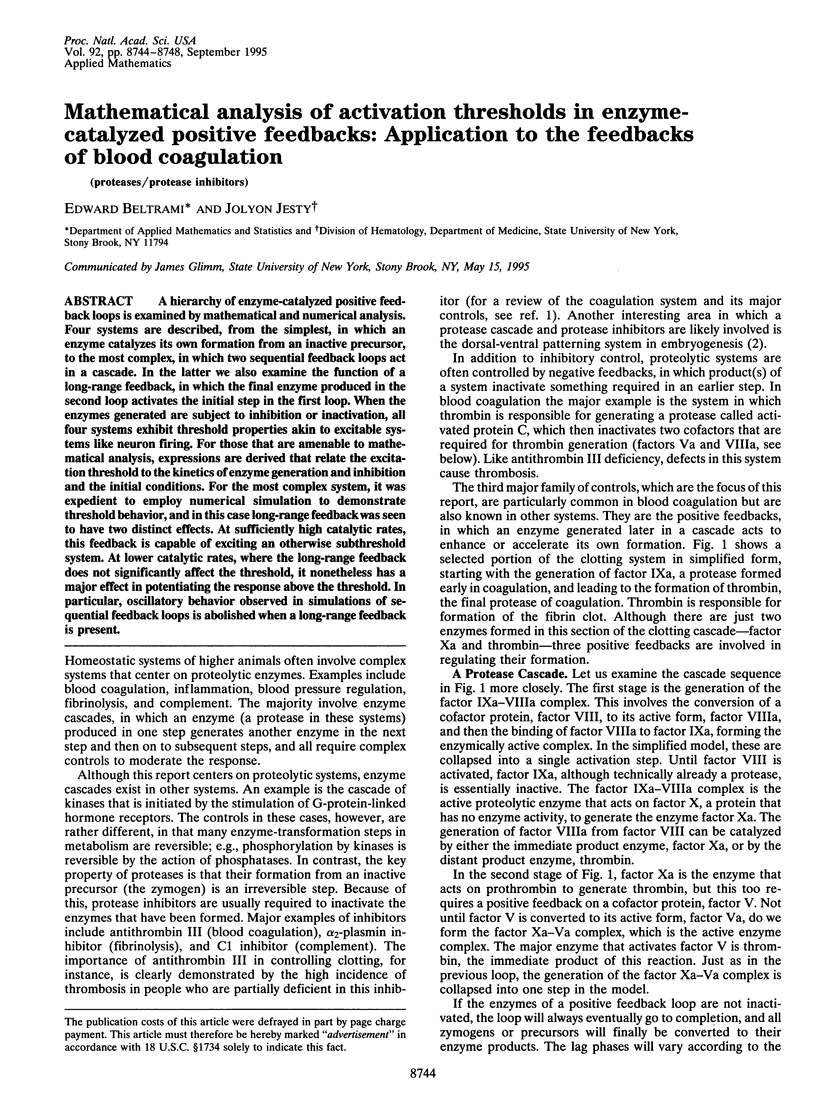
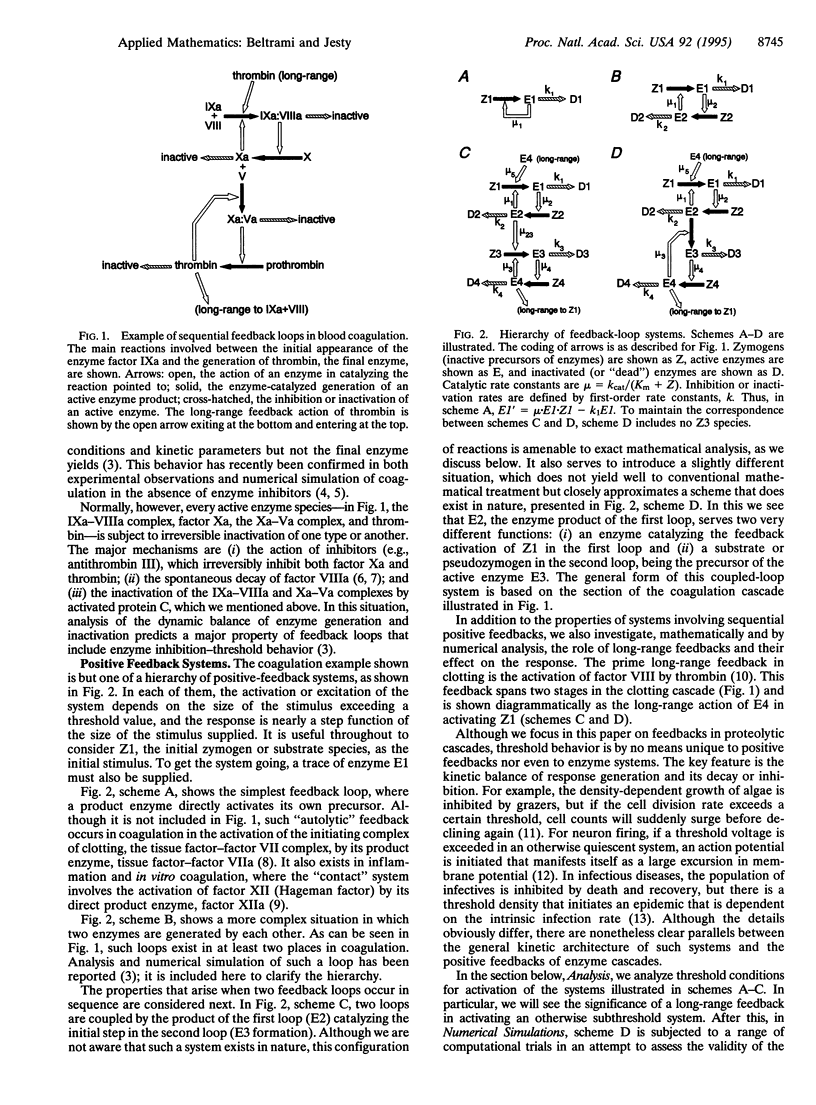
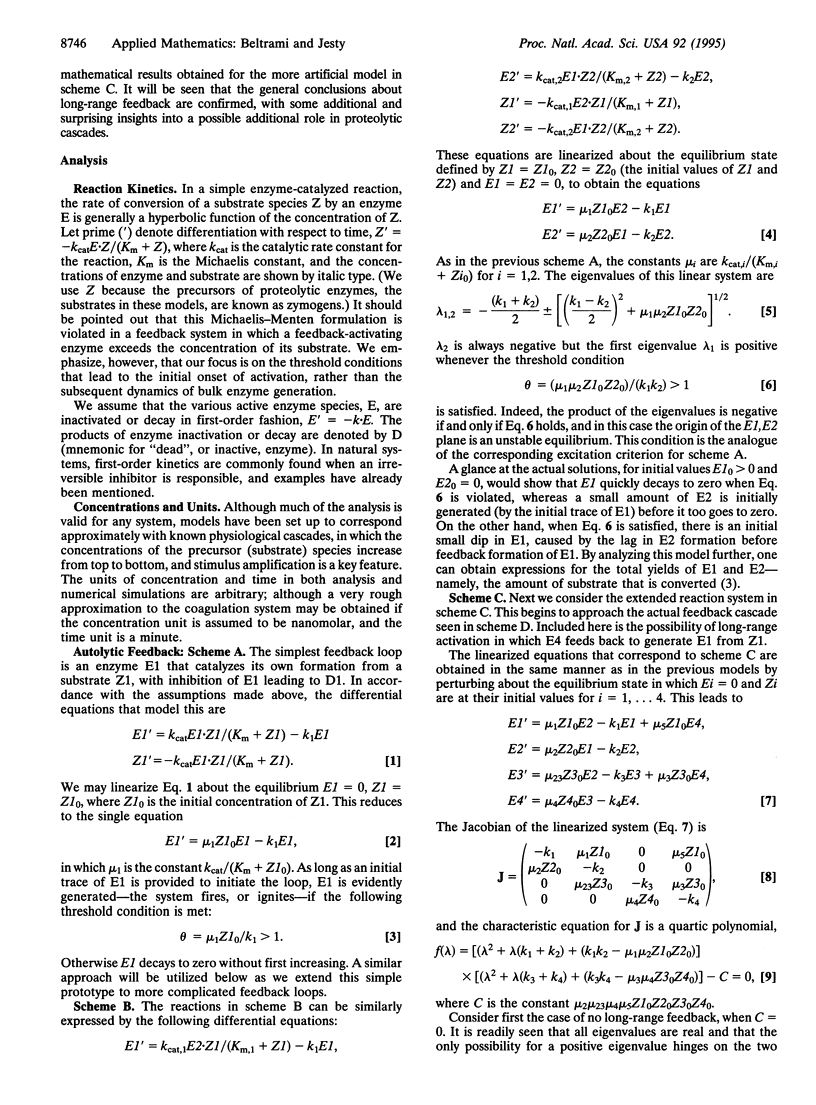
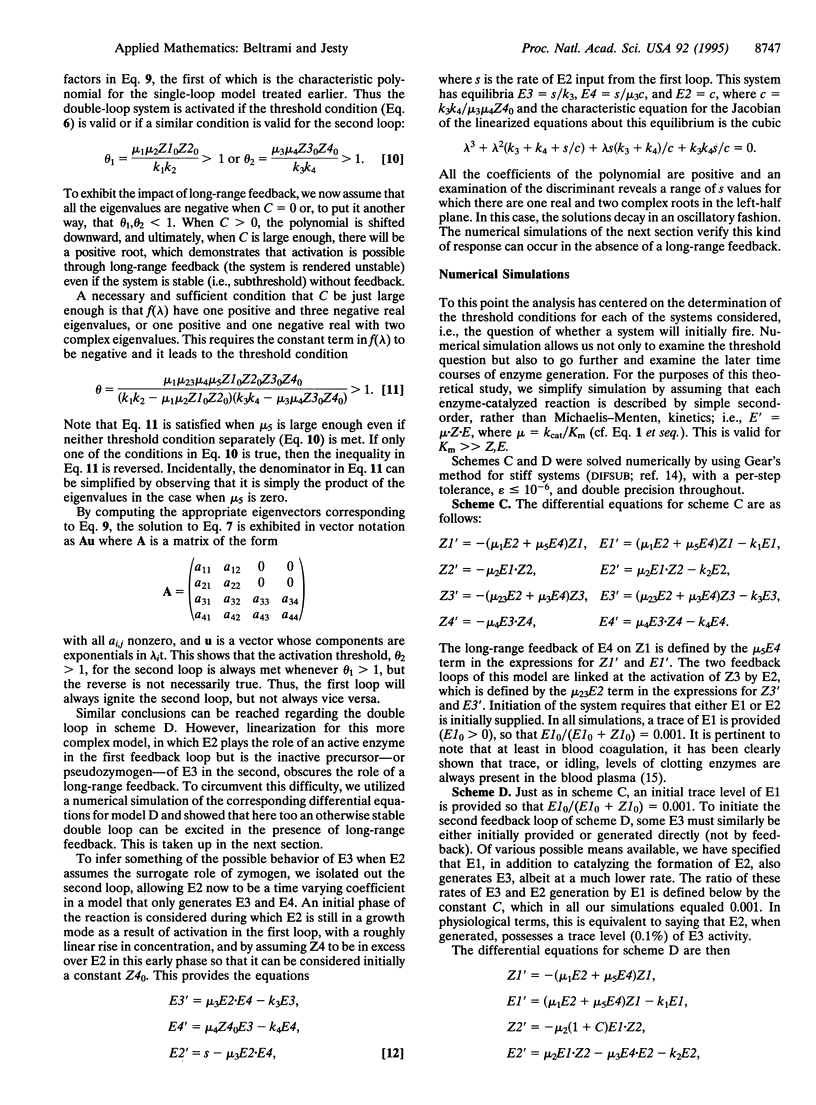
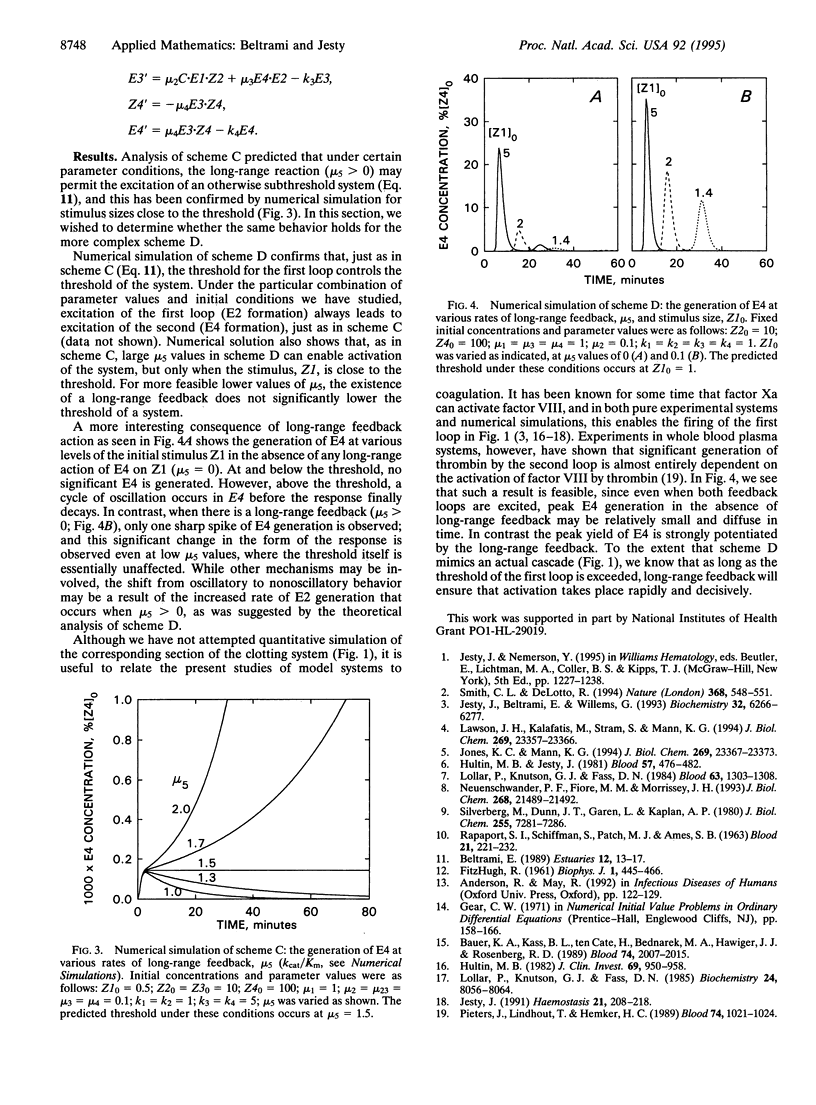
Selected References
These references are in PubMed. This may not be the complete list of references from this article.
- Bauer K. A., Kass B. L., ten Cate H., Bednarek M. A., Hawiger J. J., Rosenberg R. D. Detection of factor X activation in humans. Blood. 1989 Nov 1;74(6):2007–2015. [PubMed] [Google Scholar]
- Fitzhugh R. Impulses and Physiological States in Theoretical Models of Nerve Membrane. Biophys J. 1961 Jul;1(6):445–466. doi: 10.1016/s0006-3495(61)86902-6. [DOI] [PMC free article] [PubMed] [Google Scholar]
- Hultin M. B., Jesty J. The activation and inactivation of human factor VIII by thrombin: effect of inhibitors of thrombin. Blood. 1981 Mar;57(3):476–482. [PubMed] [Google Scholar]
- Hultin M. B. Role of human factor VIII in factor X activation. J Clin Invest. 1982 Apr;69(4):950–958. doi: 10.1172/JCI110534. [DOI] [PMC free article] [PubMed] [Google Scholar]
- Jesty J., Beltrami E., Willems G. Mathematical analysis of a proteolytic positive-feedback loop: dependence of lag time and enzyme yields on the initial conditions and kinetic parameters. Biochemistry. 1993 Jun 22;32(24):6266–6274. doi: 10.1021/bi00075a021. [DOI] [PubMed] [Google Scholar]
- Jesty J. Interaction of feedback control and product inhibition in the activation of factor X by factors IXa and VIII. Haemostasis. 1991;21(4):208–218. doi: 10.1159/000216230. [DOI] [PubMed] [Google Scholar]
- Jones K. C., Mann K. G. A model for the tissue factor pathway to thrombin. II. A mathematical simulation. J Biol Chem. 1994 Sep 16;269(37):23367–23373. [PubMed] [Google Scholar]
- Lawson J. H., Kalafatis M., Stram S., Mann K. G. A model for the tissue factor pathway to thrombin. I. An empirical study. J Biol Chem. 1994 Sep 16;269(37):23357–23366. [PubMed] [Google Scholar]
- Lollar P., Knutson G. J., Fass D. N. Activation of porcine factor VIII:C by thrombin and factor Xa. Biochemistry. 1985 Dec 31;24(27):8056–8064. doi: 10.1021/bi00348a033. [DOI] [PubMed] [Google Scholar]
- Lollar P., Knutson G. J., Fass D. N. Stabilization of thrombin-activated porcine factor VIII:C by factor IXa phospholipid. Blood. 1984 Jun;63(6):1303–1308. [PubMed] [Google Scholar]
- Neuenschwander P. F., Fiore M. M., Morrissey J. H. Factor VII autoactivation proceeds via interaction of distinct protease-cofactor and zymogen-cofactor complexes. Implications of a two-dimensional enzyme kinetic mechanism. J Biol Chem. 1993 Oct 15;268(29):21489–21492. [PubMed] [Google Scholar]
- Pieters J., Lindhout T., Hemker H. C. In situ-generated thrombin is the only enzyme that effectively activates factor VIII and factor V in thromboplastin-activated plasma. Blood. 1989 Aug 15;74(3):1021–1024. [PubMed] [Google Scholar]
- RAPAPORT S. I., SCHIFFMAN S., PATCH M. J., AMES S. B. The importance of activation of antihemophilic globulin and proaccelerin by traces of thrombin in the generation of intrinsic prothrombinase activity. Blood. 1963 Feb;21:221–236. [PubMed] [Google Scholar]
- Silverberg M., Dunn J. T., Garen L., Kaplan A. P. Autoactivation of human Hageman factor. Demonstration utilizing a synthetic substrate. J Biol Chem. 1980 Aug 10;255(15):7281–7286. [PubMed] [Google Scholar]
- Smith C. L., DeLotto R. Ventralizing signal determined by protease activation in Drosophila embryogenesis. Nature. 1994 Apr 7;368(6471):548–551. doi: 10.1038/368548a0. [DOI] [PubMed] [Google Scholar]