Abstract
Although the rates of chemical reactions become faster with increasing temperature, the converse may be observed with protein-folding reactions. The rate constant for folding initially increases with temperature, goes through a maximum, and then decreases. The activation enthalpy is thus highly temperature dependent because of a large change in specific heat (delta Cp). Such a delta Cp term is usually presumed to be a consequence of a large decrease in exposure of hydrophobic surfaces to water as the reaction proceeds from the denatured state to the transition state for folding: the hydrophobic side chains are surrounded by "icebergs" of water that melt with increasing temperature, thus making a large contribution to the Cp of the denatured state and a smaller one to the more compact transition state. The rate could also be affected by temperature-induced changes in the conformational population of the ground state: the heat required for the progressive melting of residual structure in the denatured state will contribute to delta Cp. By examining two proteins with different refolding mechanisms, we are able to find both of these two processes; barley chymotrypsin inhibitor 2, which refolds from a highly unfolded state, fits well to a hydrophobic interaction model with a constant delta Cp of activation, whereas barnase, which refolds from a more structured denatured state, deviates from this ideal behavior.
Full text
PDF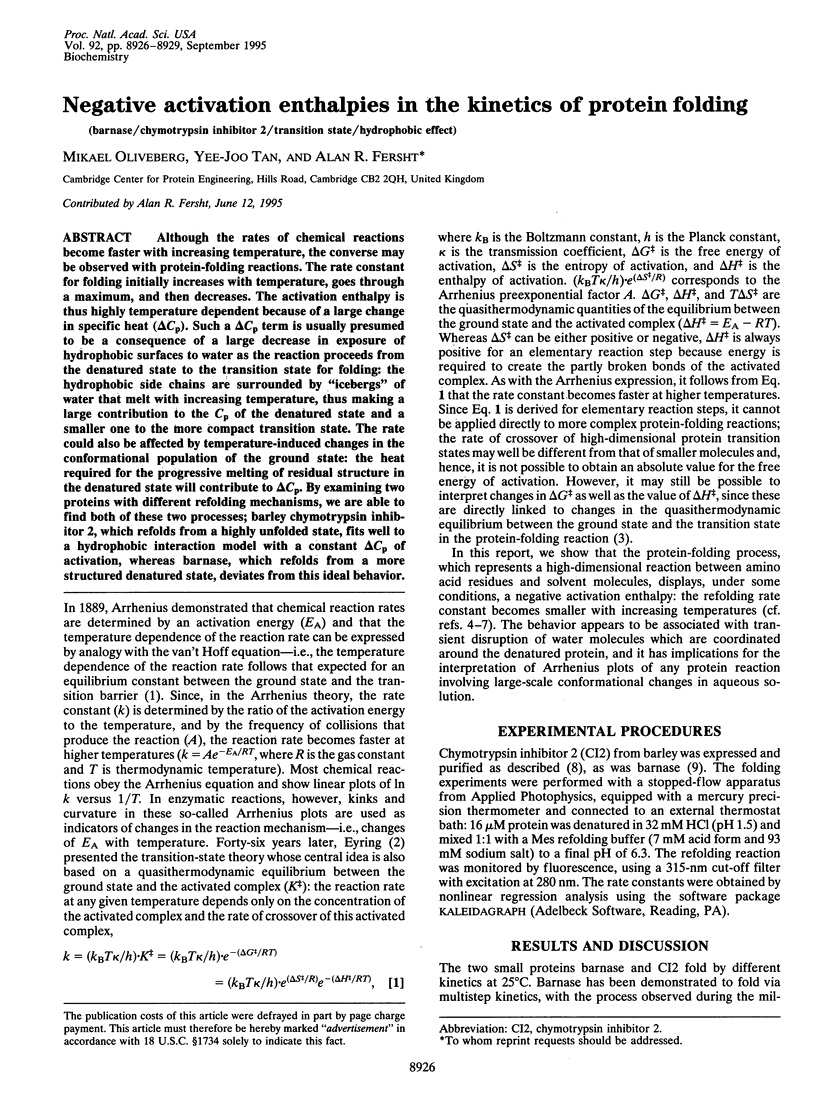
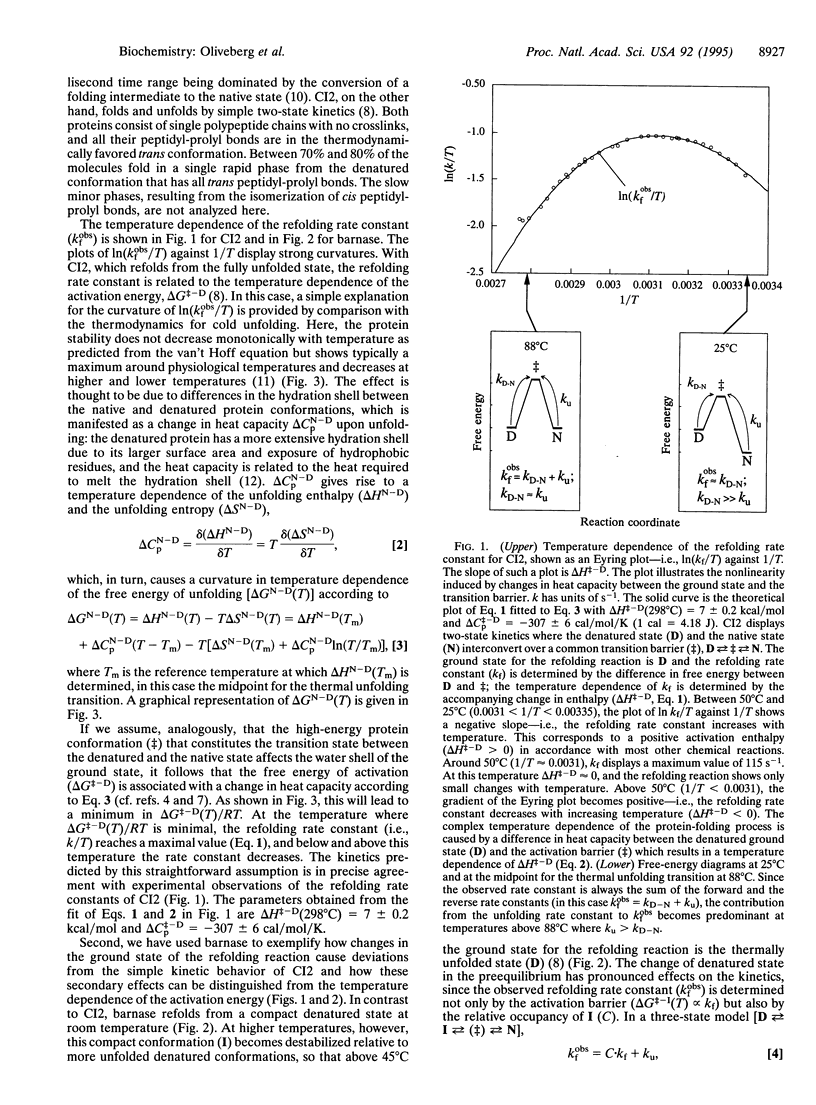
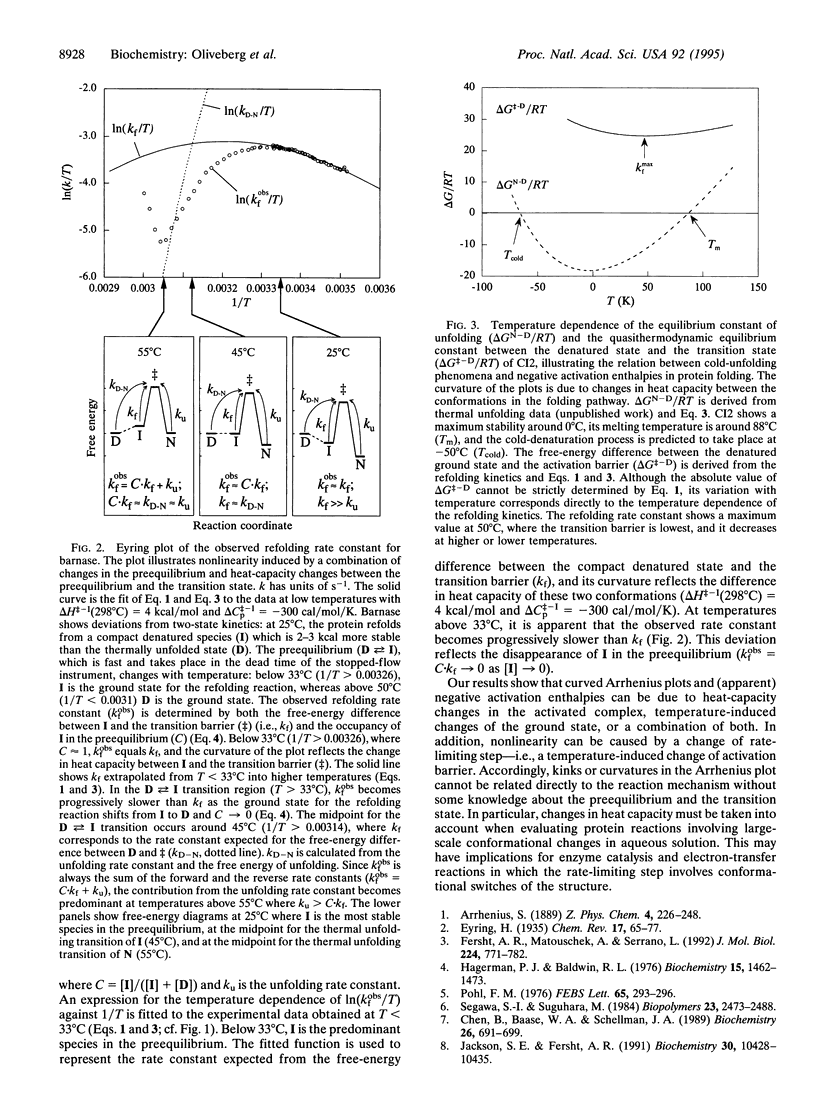
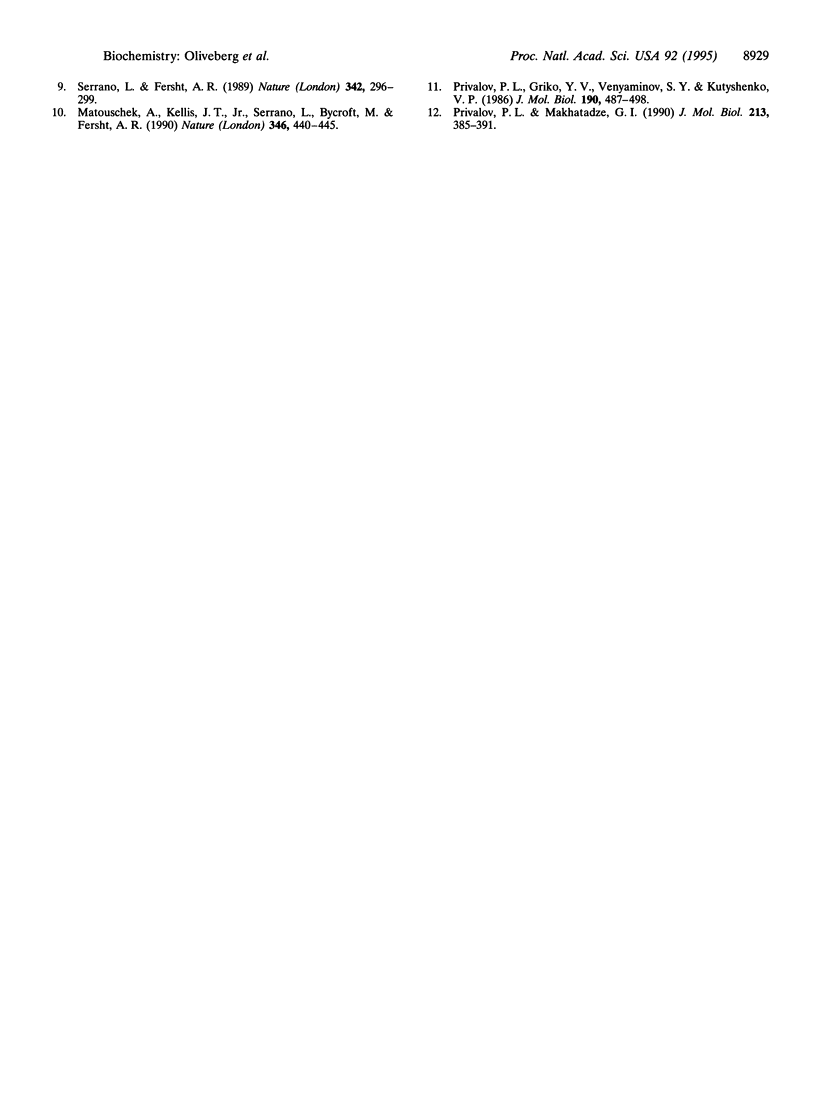
Selected References
These references are in PubMed. This may not be the complete list of references from this article.
- Chen B. L., Baase W. A., Schellman J. A. Low-temperature unfolding of a mutant of phage T4 lysozyme. 2. Kinetic investigations. Biochemistry. 1989 Jan 24;28(2):691–699. doi: 10.1021/bi00428a042. [DOI] [PubMed] [Google Scholar]
- Fersht A. R., Matouschek A., Serrano L. The folding of an enzyme. I. Theory of protein engineering analysis of stability and pathway of protein folding. J Mol Biol. 1992 Apr 5;224(3):771–782. doi: 10.1016/0022-2836(92)90561-w. [DOI] [PubMed] [Google Scholar]
- Hagerman P. J., Baldwin R. L. A quantitative treatment of the kinetics of the folding transition of ribonuclease A. Biochemistry. 1976 Apr 6;15(7):1462–1473. doi: 10.1021/bi00652a017. [DOI] [PubMed] [Google Scholar]
- Jackson S. E., Fersht A. R. Folding of chymotrypsin inhibitor 2. 1. Evidence for a two-state transition. Biochemistry. 1991 Oct 29;30(43):10428–10435. doi: 10.1021/bi00107a010. [DOI] [PubMed] [Google Scholar]
- Matouschek A., Kellis J. T., Jr, Serrano L., Bycroft M., Fersht A. R. Transient folding intermediates characterized by protein engineering. Nature. 1990 Aug 2;346(6283):440–445. doi: 10.1038/346440a0. [DOI] [PubMed] [Google Scholar]
- Pohl F. M. Temperature-dependence of the kinetics of folding of chymotrypsinogen A. FEBS Lett. 1976 Jun 15;65(3):293–296. doi: 10.1016/0014-5793(76)80132-9. [DOI] [PubMed] [Google Scholar]
- Privalov P. L., Griko YuV, Venyaminov SYu, Kutyshenko V. P. Cold denaturation of myoglobin. J Mol Biol. 1986 Aug 5;190(3):487–498. doi: 10.1016/0022-2836(86)90017-3. [DOI] [PubMed] [Google Scholar]
- Privalov P. L., Makhatadze G. I. Heat capacity of proteins. II. Partial molar heat capacity of the unfolded polypeptide chain of proteins: protein unfolding effects. J Mol Biol. 1990 May 20;213(2):385–391. doi: 10.1016/S0022-2836(05)80198-6. [DOI] [PubMed] [Google Scholar]
- Segawa S., Sugihara M. Characterization of the transition state of lysozyme unfolding. I. Effect of protein-solvent interactions on the transition state. Biopolymers. 1984 Nov;23(11 Pt 2):2473–2488. doi: 10.1002/bip.360231122. [DOI] [PubMed] [Google Scholar]
- Serrano L., Fersht A. R. Capping and alpha-helix stability. Nature. 1989 Nov 16;342(6247):296–299. doi: 10.1038/342296a0. [DOI] [PubMed] [Google Scholar]