Abstract
Long-term (18-24 hr) preincubation of NIL hamster cell cultures with D-glucose or D-glucosamine (both of which repress the hexose transport system) gave rise to a striking loss of the hexose transport system ("super-repression") when cycloheximide was also present in the culture medium. However, if 0.2 mM 2,4-dinitrophenol (DNP) was also present, the cycloheximide-mediated super-repression was prevented. Moreover, the presence of DNP at this low concentration contributed to an increase in hexose uptake such that it was substantially higher than that permitted by either of the two repressive sugars alone. When the cultures were maintained in medium containing D-fructose in place of glucose, a marked increase in uptake occurred, and this increase (derepression) was not affected by DNP. The derepression due to glucose deprivation and the increases caused by DNP treatment were also observed when 3-O-methylglucose was used to measure hexose transport. Although cultures maintained in the presence of glucosamine exhibited a repressed hexose transport rate, they did not generate significant amounts of lactic acid. DNP, and other uncouplers of oxidative phosphorylation, promoted a derepressed state of hexose transport but did not stimulate the generation of lactate from glucosamine. These data suggest that the metabolic repression phenomena of hexose transport do not depend on glycolysis but rather on the "energized" state of the cell. The energized state of the cell may also be required for the super-repression of hexose transport that is especially apparent when protein synthesis is blocked by cycloheximide.
Full text
PDF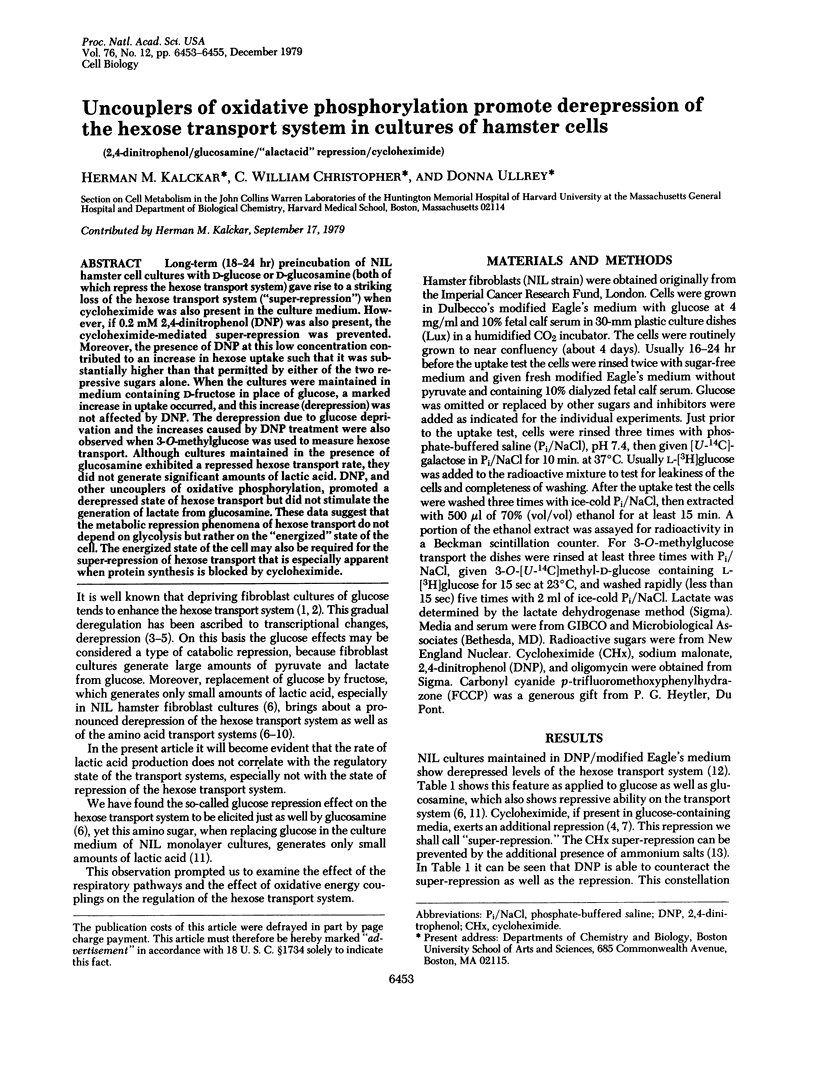
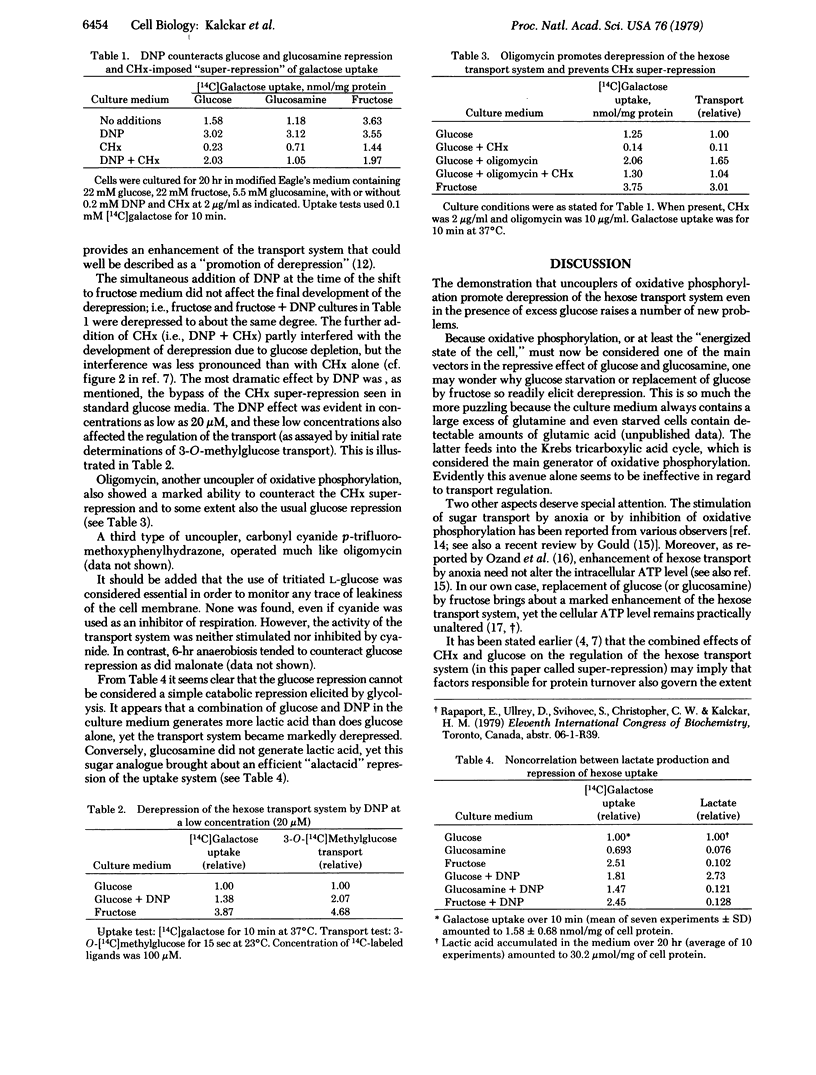
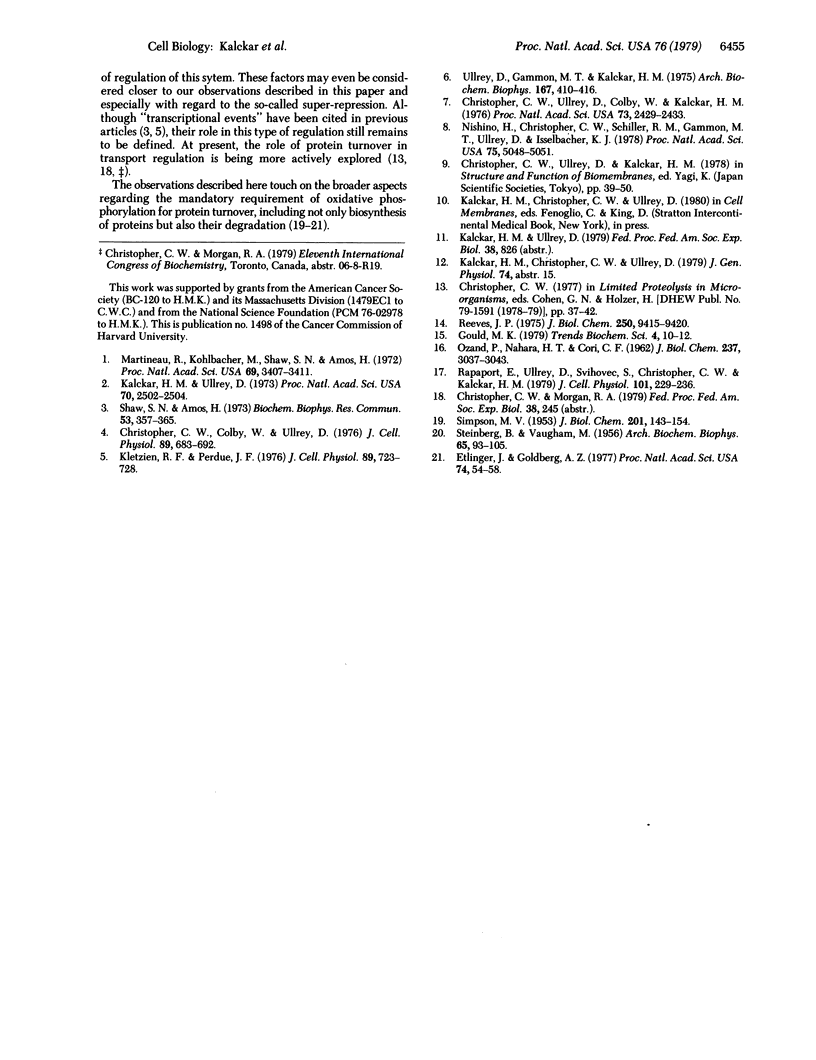
Selected References
These references are in PubMed. This may not be the complete list of references from this article.
- Christopher C. W., Colby W. W., Ullrey D. Derepression and carrier turnover: evidence for two distinct mechanisms of hexose transport regulation in animal cells. J Cell Physiol. 1976 Dec;89(4):683–692. doi: 10.1002/jcp.1040890427. [DOI] [PubMed] [Google Scholar]
- Christopher C. W., Ullrey D., Colby W., Kalckar M. Paradoxical effects of cycloheximide and cytochalasin B on hamster cell hexose uptake. Proc Natl Acad Sci U S A. 1976 Jul;73(7):2429–2433. doi: 10.1073/pnas.73.7.2429. [DOI] [PMC free article] [PubMed] [Google Scholar]
- Etlinger J. D., Goldberg A. L. A soluble ATP-dependent proteolytic system responsible for the degradation of abnormal proteins in reticulocytes. Proc Natl Acad Sci U S A. 1977 Jan;74(1):54–58. doi: 10.1073/pnas.74.1.54. [DOI] [PMC free article] [PubMed] [Google Scholar]
- Kalckar H. M., Ullrey D. Two distinct types of enhancement of galactose uptake into hamster cells: tumor-virus transformation and hexose starvation. Proc Natl Acad Sci U S A. 1973 Sep;70(9):2502–2504. doi: 10.1073/pnas.70.9.2502. [DOI] [PMC free article] [PubMed] [Google Scholar]
- Kletzien R. F., Perdue J. F. Regulation of sugar transport in chick embryo fibroblasts and in fibroblasts transformed by a temperature-sensitive mutant of the Rous sarcoma virus. J Cell Physiol. 1976 Dec;89(4):723–728. doi: 10.1002/jcp.1040890432. [DOI] [PubMed] [Google Scholar]
- Martineau R., Kohlbacher M., Shaw S. N., Amos H. Enhancement of hexose entry into chick fibroblasts by starvation: differential effect on galactose and glucose. Proc Natl Acad Sci U S A. 1972 Nov;69(11):3407–3411. doi: 10.1073/pnas.69.11.3407. [DOI] [PMC free article] [PubMed] [Google Scholar]
- Nishino H., Christopher C. W., Schiller R. M., Gammon M. T., Ullrey D., Isselbacher K. J. Sodium-dependent amino acid transport by cultured hamster cells: membrane vesicles retain transport changes due to glucose starvation and cycloheximide. Proc Natl Acad Sci U S A. 1978 Oct;75(10):5048–5051. doi: 10.1073/pnas.75.10.5048. [DOI] [PMC free article] [PubMed] [Google Scholar]
- OZAND P., NARAHARA H. T., CORI C. F. Studies of tissue permeability. VIII. The effect of anaerobiosis on glucose uptake in frog sartorius muscle. J Biol Chem. 1962 Oct;237:3037–3043. [PubMed] [Google Scholar]
- Rapaport E., Christopher C. W., Svihovec S. K., Ullrey D., Kalckar H. M. Selective high metabolic lability of uridine, guanosine and cytosine triphosphates in response to glucose deprivation and refeeding of untransformed and polyoma virus-transformed hamster fibroblasts. J Cell Physiol. 1979 Nov;101(2):229–235. doi: 10.1002/jcp.1041010205. [DOI] [PubMed] [Google Scholar]
- Reeves J. P. Stimulation of 3-O-methylglucose transport by anaerobiosis in rat thymocytes. J Biol Chem. 1975 Dec 25;250(24):9413–9420. [PubMed] [Google Scholar]
- SIMPSON M. V. The release of labeled amino acids from the proteins of rat liver slices. J Biol Chem. 1953 Mar;201(1):143–154. [PubMed] [Google Scholar]
- STEINBERG D., VAUGHAN M. Observations on intracellular protein catabolism studied in vitro. Arch Biochem Biophys. 1956 Nov;65(1):93–105. doi: 10.1016/0003-9861(56)90180-1. [DOI] [PubMed] [Google Scholar]
- Shaw S. N., Amos H. Insulin stimulation of glucose entry in chick fibroblasts and HeLa cells. Biochem Biophys Res Commun. 1973 Jul 2;53(1):357–365. doi: 10.1016/0006-291x(73)91441-1. [DOI] [PubMed] [Google Scholar]
- Ullrey D., Gammon M. T., Kalckar H. M. Uptake patterns and transport enhancements in cultures of hamster cells deprived of carbohydrates. Arch Biochem Biophys. 1975 Apr;167(2):410–416. doi: 10.1016/0003-9861(75)90481-6. [DOI] [PubMed] [Google Scholar]