Abstract
Mannitol is the most abundant sugar alcohol in nature, occurring in bacteria, fungi, lichens, and many species of vascular plants. Celery (Apium graveolens L.), a plant that forms mannitol photosynthetically, has high photosynthetic rates thought to results from intrinsic differences in the biosynthesis of hexitols vs. sugars. Celery also exhibits high salt tolerance due to the function of mannitol as an osmoprotectant. A mannitol catabolic enzyme that oxidizes mannitol to mannose (mannitol dehydrogenase, MTD) has been identified. In celery plants, MTD activity and tissue mannitol concentration are inversely related. MTD provides the initial step by which translocated mannitol is committed to central metabolism and, by regulating mannitol pool size, is important in regulating salt tolerance at the cellular level. We have now isolated, sequenced, and characterized a Mtd cDNA from celery. Analyses showed that Mtd RNA was more abundant in cells grown on mannitol and less abundant in salt-stressed cells. A protein database search revealed that the previously described ELI3 pathogenesis-related proteins from parsley and Arabidopsis are MTDs. Treatment of celery cells with salicylic acid resulted in increased MTD activity and RNA. Increased MTD activity results in an increased ability to utilize mannitol. Among other effects, this may provide an additional source of carbon and energy for response to pathogen attack. These responses of the primary enzyme controlling mannitol pool size reflect the importance of mannitol metabolism in plant responses to divergent types of environmental stress.
Full text
PDF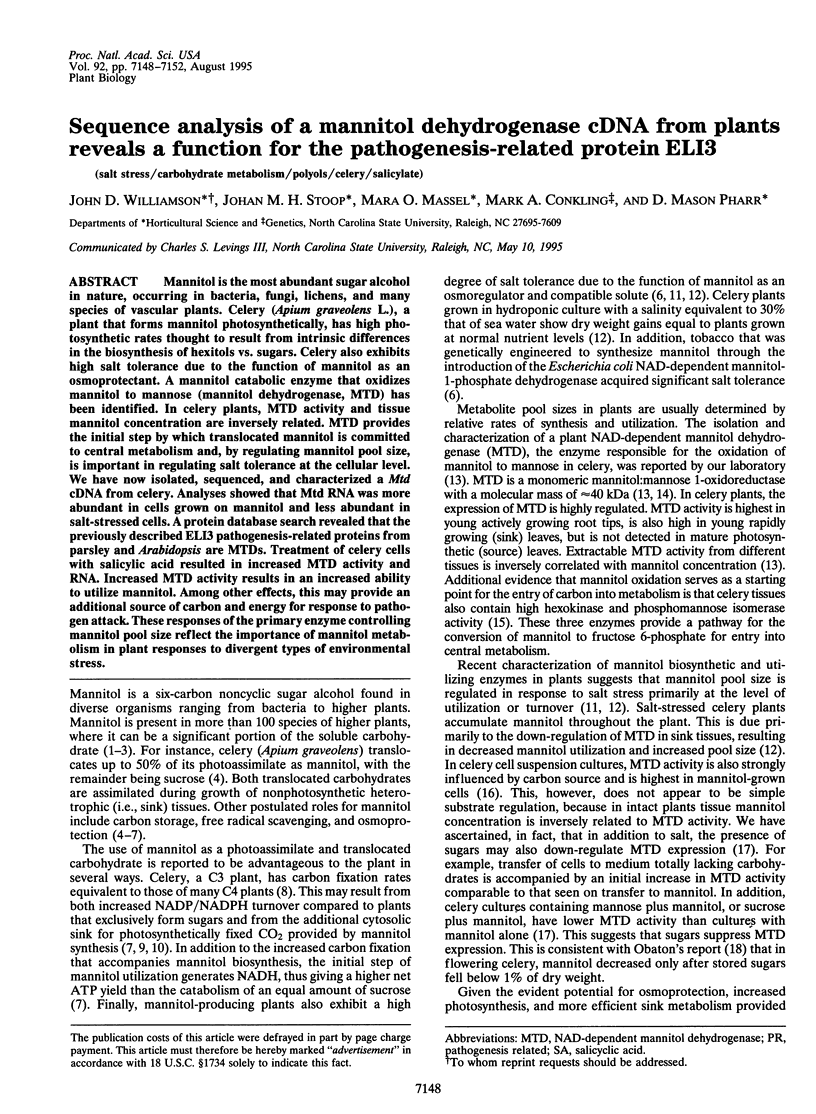
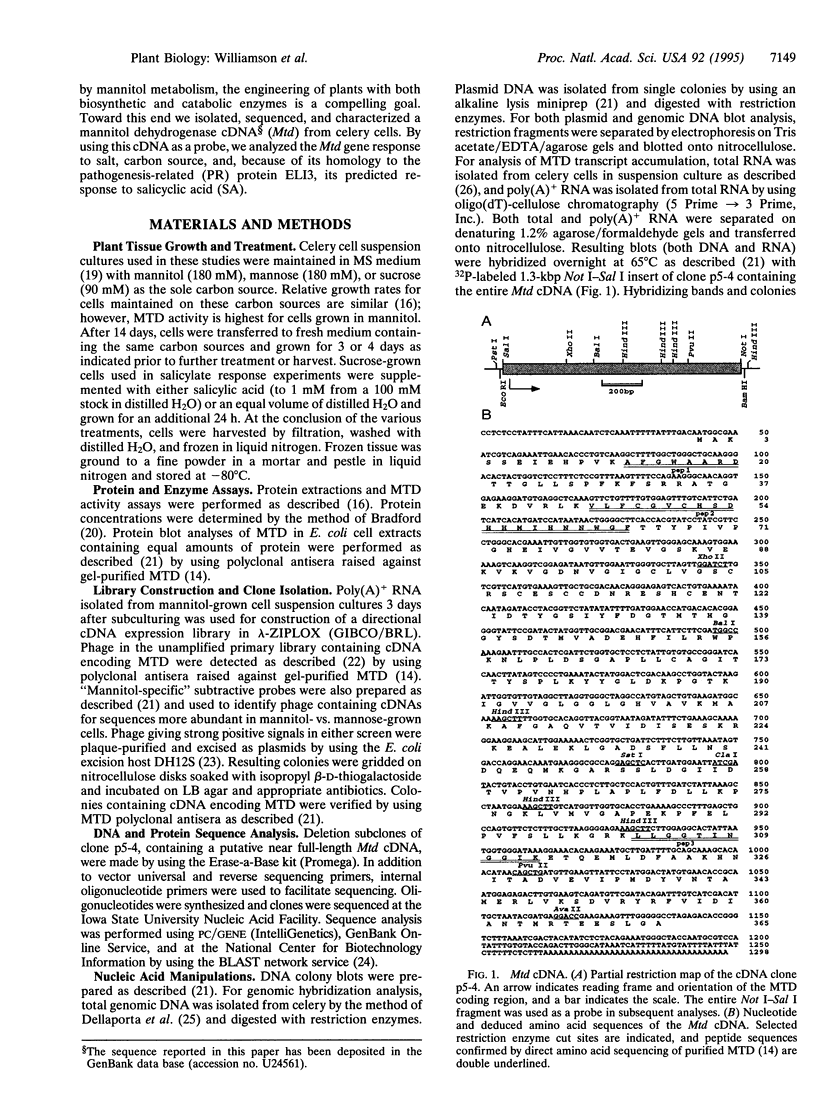
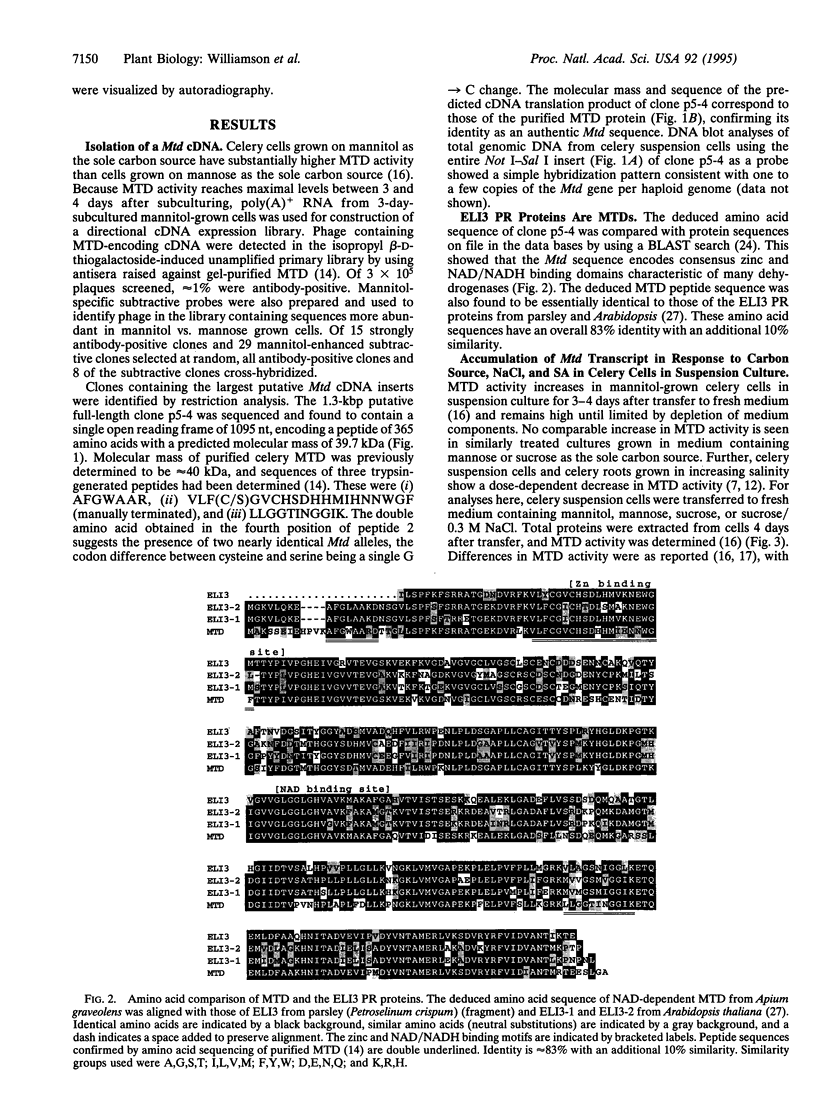
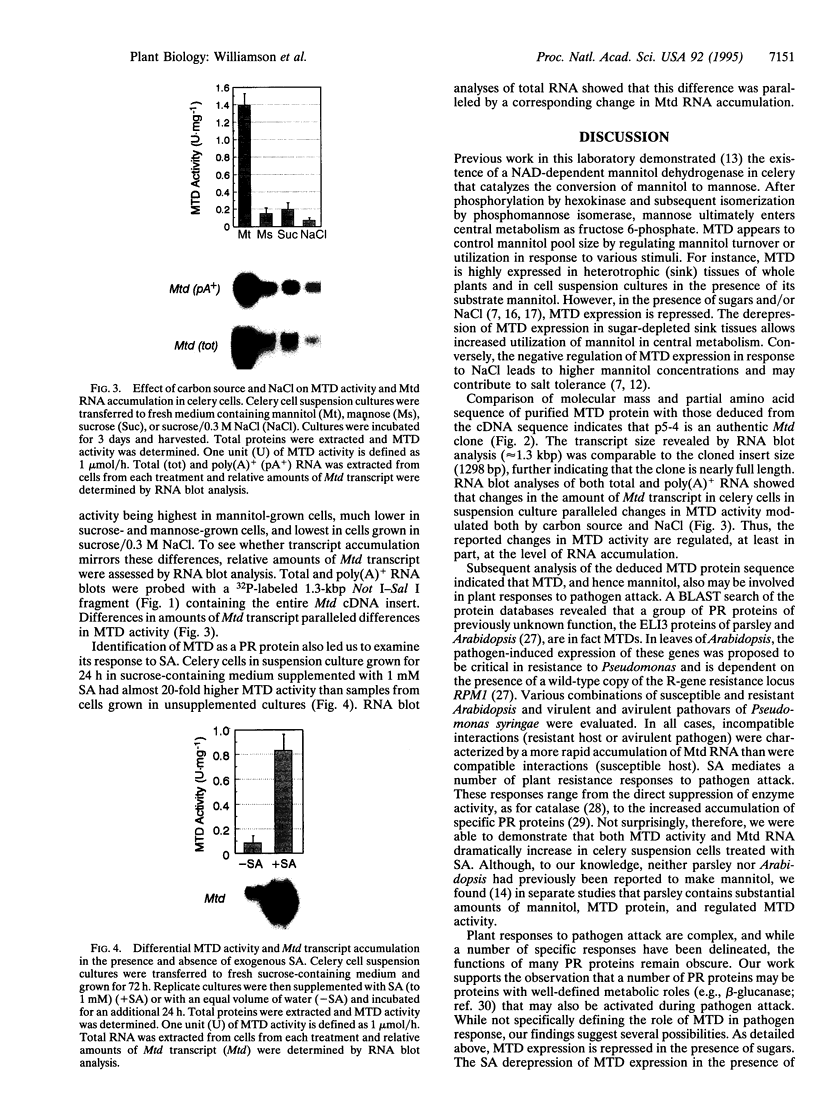
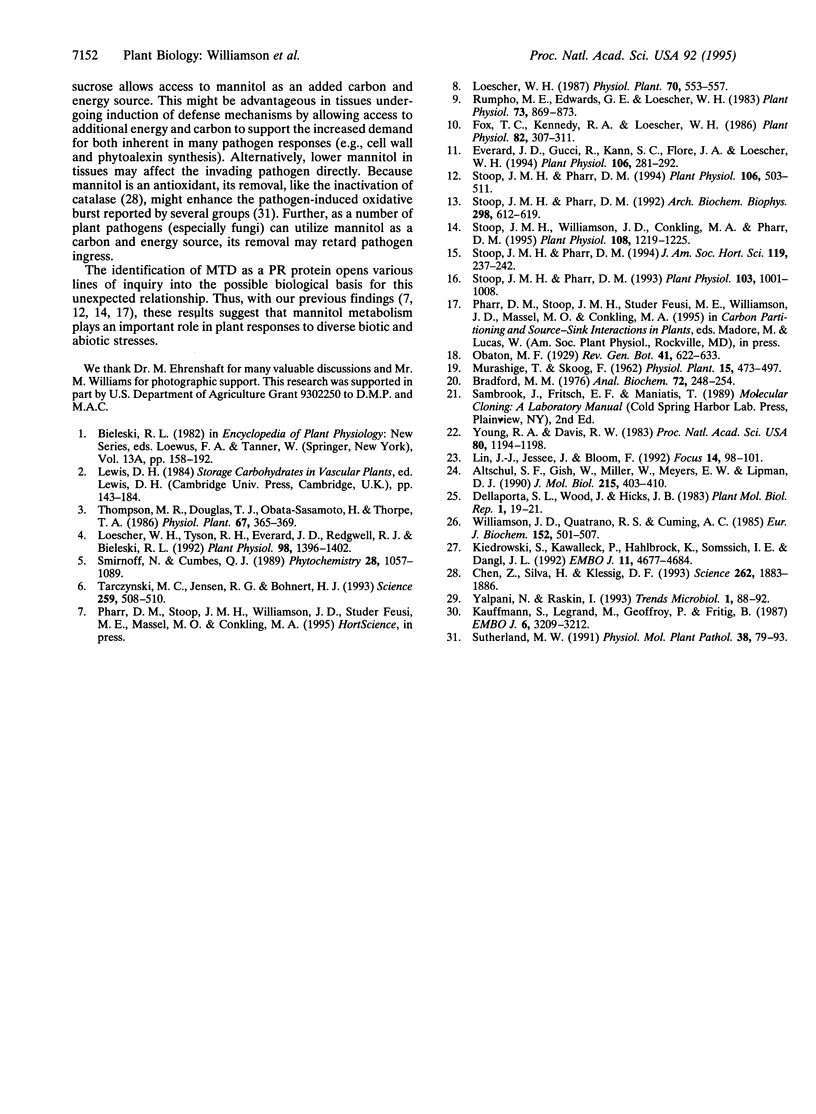
Images in this article
Selected References
These references are in PubMed. This may not be the complete list of references from this article.
- Altschul S. F., Gish W., Miller W., Myers E. W., Lipman D. J. Basic local alignment search tool. J Mol Biol. 1990 Oct 5;215(3):403–410. doi: 10.1016/S0022-2836(05)80360-2. [DOI] [PubMed] [Google Scholar]
- Bradford M. M. A rapid and sensitive method for the quantitation of microgram quantities of protein utilizing the principle of protein-dye binding. Anal Biochem. 1976 May 7;72:248–254. doi: 10.1016/0003-2697(76)90527-3. [DOI] [PubMed] [Google Scholar]
- Chen Z., Silva H., Klessig D. F. Active oxygen species in the induction of plant systemic acquired resistance by salicylic acid. Science. 1993 Dec 17;262(5141):1883–1886. doi: 10.1126/science.8266079. [DOI] [PubMed] [Google Scholar]
- Everard J. D., Gucci R., Kann S. C., Flore J. A., Loescher W. H. Gas Exchange and Carbon Partitioning in the Leaves of Celery (Apium graveolens L.) at Various Levels of Root Zone Salinity. Plant Physiol. 1994 Sep;106(1):281–292. doi: 10.1104/pp.106.1.281. [DOI] [PMC free article] [PubMed] [Google Scholar]
- Fox T. C., Kennedy R. A., Loescher W. H. Developmental Changes in Photosynthetic Gas Exchange in the Polyol-Synthesizing Species, Apium graveolens L. (Celery). Plant Physiol. 1986 Sep;82(1):307–311. doi: 10.1104/pp.82.1.307. [DOI] [PMC free article] [PubMed] [Google Scholar]
- Kauffmann S., Legrand M., Geoffroy P., Fritig B. Biological function of ;pathogenesis-related' proteins: four PR proteins of tobacco have 1,3-beta-glucanase activity. EMBO J. 1987 Nov;6(11):3209–3212. doi: 10.1002/j.1460-2075.1987.tb02637.x. [DOI] [PMC free article] [PubMed] [Google Scholar]
- Kiedrowski S., Kawalleck P., Hahlbrock K., Somssich I. E., Dangl J. L. Rapid activation of a novel plant defense gene is strictly dependent on the Arabidopsis RPM1 disease resistance locus. EMBO J. 1992 Dec;11(13):4677–4684. doi: 10.1002/j.1460-2075.1992.tb05572.x. [DOI] [PMC free article] [PubMed] [Google Scholar]
- Loescher W. H., Tyson R. H., Everard J. D., Redgwell R. J., Bieleski R. L. Mannitol Synthesis in Higher Plants : Evidence for the Role and Characterization of a NADPH-Dependent Mannose 6-Phosphate Reductase. Plant Physiol. 1992 Apr;98(4):1396–1402. doi: 10.1104/pp.98.4.1396. [DOI] [PMC free article] [PubMed] [Google Scholar]
- Rumpho M. E., Edwards G. E., Loescher W. H. A pathway for photosynthetic carbon flow to mannitol in celery leaves : activity and localization of key enzymes. Plant Physiol. 1983 Dec;73(4):869–873. doi: 10.1104/pp.73.4.869. [DOI] [PMC free article] [PubMed] [Google Scholar]
- Stoop J. M., Pharr D. M. Partial purification and characterization of mannitol: mannose 1-oxidoreductase from celeriac (Apium graveolens var. rapaceum) roots. Arch Biochem Biophys. 1992 Nov 1;298(2):612–619. doi: 10.1016/0003-9861(92)90456-7. [DOI] [PubMed] [Google Scholar]
- Stoop J. M., Williamson J. D., Conkling M. A., Pharr D. M. Purification of NAD-dependent mannitol dehydrogenase from celery suspension cultures. Plant Physiol. 1995 Jul;108(3):1219–1225. doi: 10.1104/pp.108.3.1219. [DOI] [PMC free article] [PubMed] [Google Scholar]
- Stoop JMH., Pharr D. M. Effect of Different Carbon Sources on Relative Growth Rate, Internal Carbohydrates, and Mannitol 1-Oxidoreductase Activity in Celery Suspension Cultures. Plant Physiol. 1993 Nov;103(3):1001–1008. doi: 10.1104/pp.103.3.1001. [DOI] [PMC free article] [PubMed] [Google Scholar]
- Stoop JMH., Pharr D. M. Mannitol Metabolism in Celery Stressed by Excess Macronutrients. Plant Physiol. 1994 Oct;106(2):503–511. doi: 10.1104/pp.106.2.503. [DOI] [PMC free article] [PubMed] [Google Scholar]
- Tarczynski M. C., Jensen R. G., Bohnert H. J. Stress protection of transgenic tobacco by production of the osmolyte mannitol. Science. 1993 Jan 22;259(5094):508–510. doi: 10.1126/science.259.5094.508. [DOI] [PubMed] [Google Scholar]
- Williamson J. D., Quatrano R. S., Cuming A. C. Em polypeptide and its messenger RNA levels are modulated by abscisic acid during embryogenesis in wheat. Eur J Biochem. 1985 Oct 15;152(2):501–507. doi: 10.1111/j.1432-1033.1985.tb09224.x. [DOI] [PubMed] [Google Scholar]
- Yalpani N., Raskin I. Salicylic acid: a systemic signal in induced plant disease resistance. Trends Microbiol. 1993 Jun;1(3):88–92. doi: 10.1016/0966-842x(93)90113-6. [DOI] [PubMed] [Google Scholar]
- Young R. A., Davis R. W. Efficient isolation of genes by using antibody probes. Proc Natl Acad Sci U S A. 1983 Mar;80(5):1194–1198. doi: 10.1073/pnas.80.5.1194. [DOI] [PMC free article] [PubMed] [Google Scholar]