Abstract
Streptococcus mutans Ingbritt was grown anaerobically in a chemostat, at a rate (mean generation time, 13 h) similar to that in dental plaque, in a complex medium with excess glucose and at pH values of 6.5, 6.0, and 5.5. The yield of cells was constant at pH 6.5 and 6.0 (2.00 mg/ml) but fell to 1.25 at pH 5.5; Yglucose was relatively constant under all conditions. Lactic acid was the major end product. Amino acid analysis of the culture supernatants indicated that growth was probably limited by the availability of cysteine. Cells were harvested and monitored for their capacity to produce acid from endogenous polysaccharide and exogenous sugars in the presence and absence of NaF, as well as for their glucose phosphoenolpyruvate (PEP)-phosphotransferase activity. Surprisingly, cells grown at pH 5.5 possessed two to three times more glycolytic activity, as measured by the rate of acid production, than cells grown at pH 6.5 and 6.0 when incubated in a washed suspension at constant pH with a sugar source. Furthermore, the cells grown at pH 5.5 were about twice as resistant to the effect of NaF in reducing the rate of acid production in this system. Fluoride inhibition could be reversed by increasing the pH of the system. Cells grown at all three pH values showed significant acid production from endogenous reserves, despite the fact that the glucoamylase-specific glycogen content of the cells dropped from 33% of the total carbohydrate during pH 6.5 growth to only 3% after growth at pH 6.0 and 6.5. Incubation of washed cells for 18 h in phosphate buffer resulted in the loss of 62% of the total carbohydrate, indicating that nonglycogen cellular polysaccharide was metabolized. A comparison of the fluoride effect on endogenous and exogenous metabolism under pH fall conditions showed that, with pH 6.5- and 6.0-grown cells, the inhibitor was more effective in the presence of an exogenous carbon source than in its absence. This effect was not seen with pH 5.5-grown cells. The decreased sensitivity of the pH 5.5-grown cells to fluoride was probably associated with the decreased glucose PEP-phosphotransferase activity (11%) in these cells compared with the activity of those grown at pH 6.5. This evidence supports the hypothesis that S. mutans possesses at least two glucose transport systems, one of which is relatively fluoride insensitive.
Full text
PDF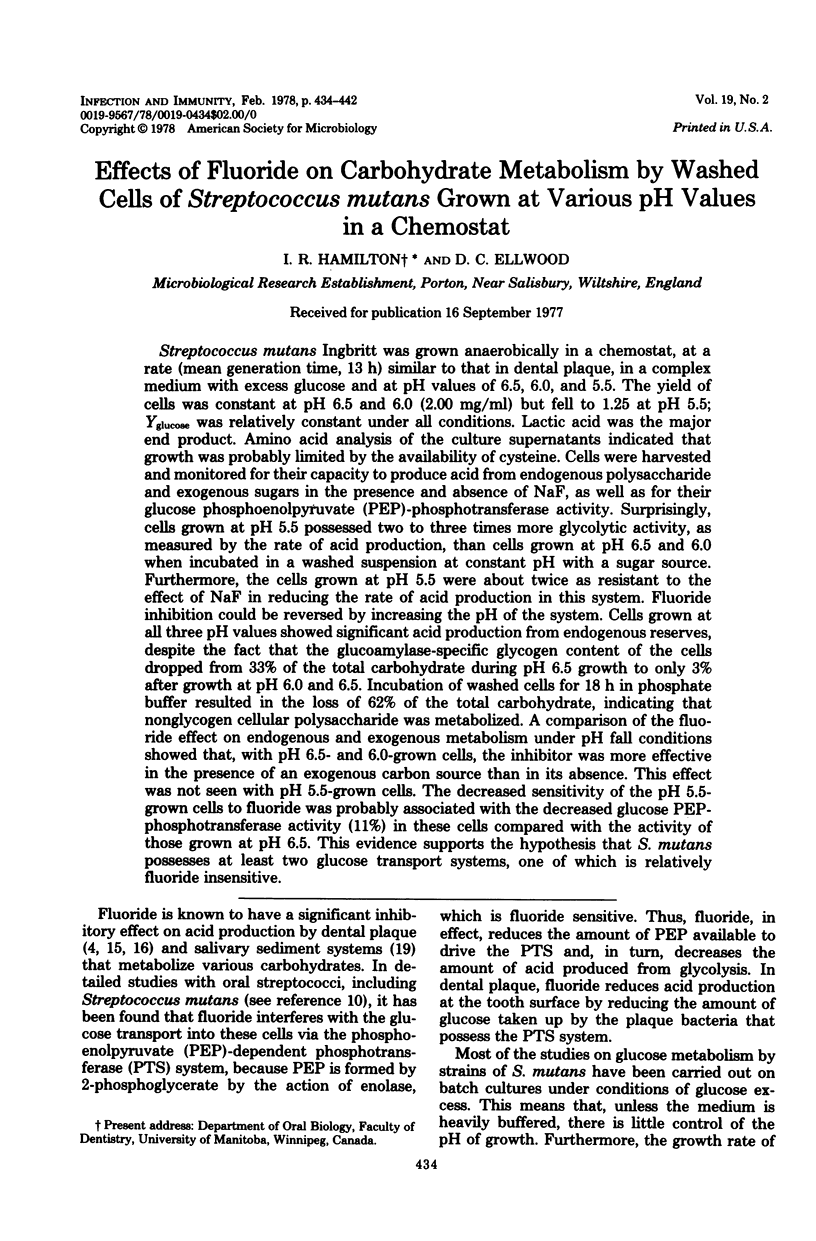
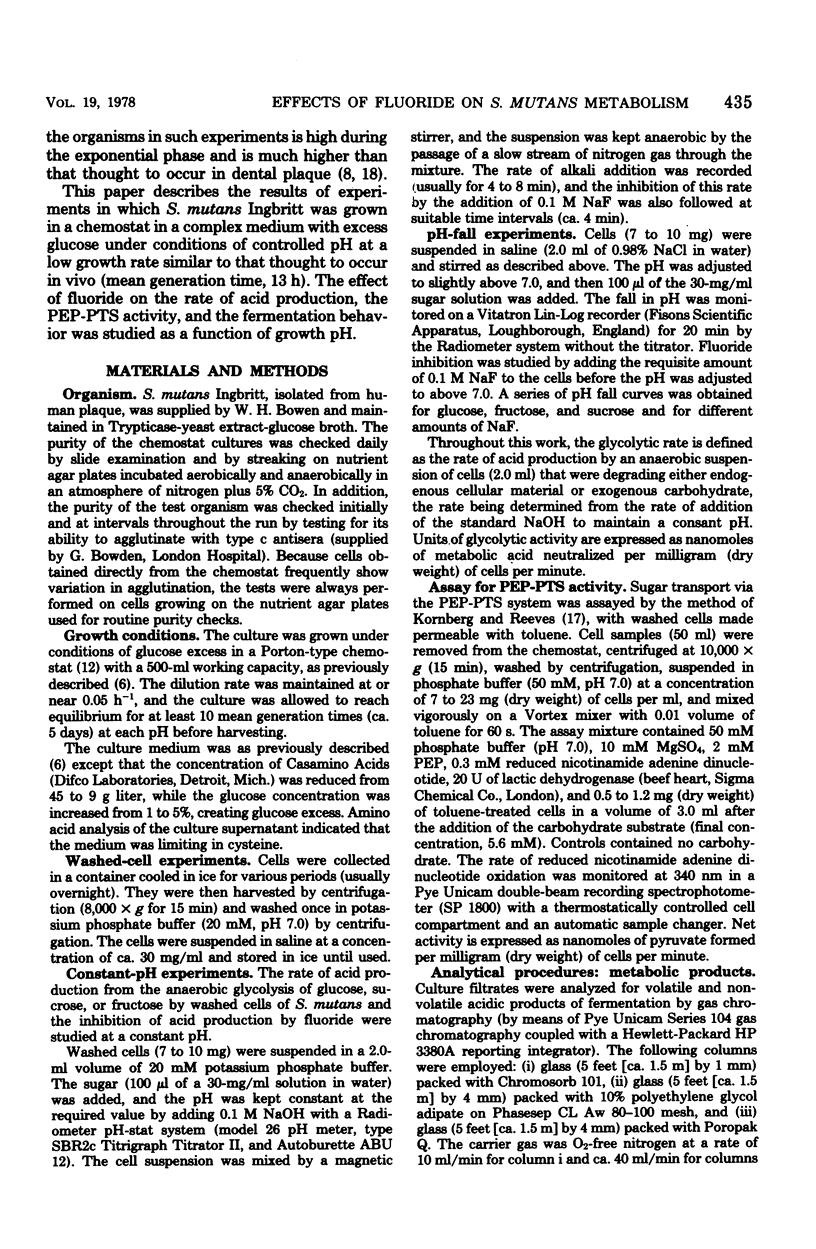
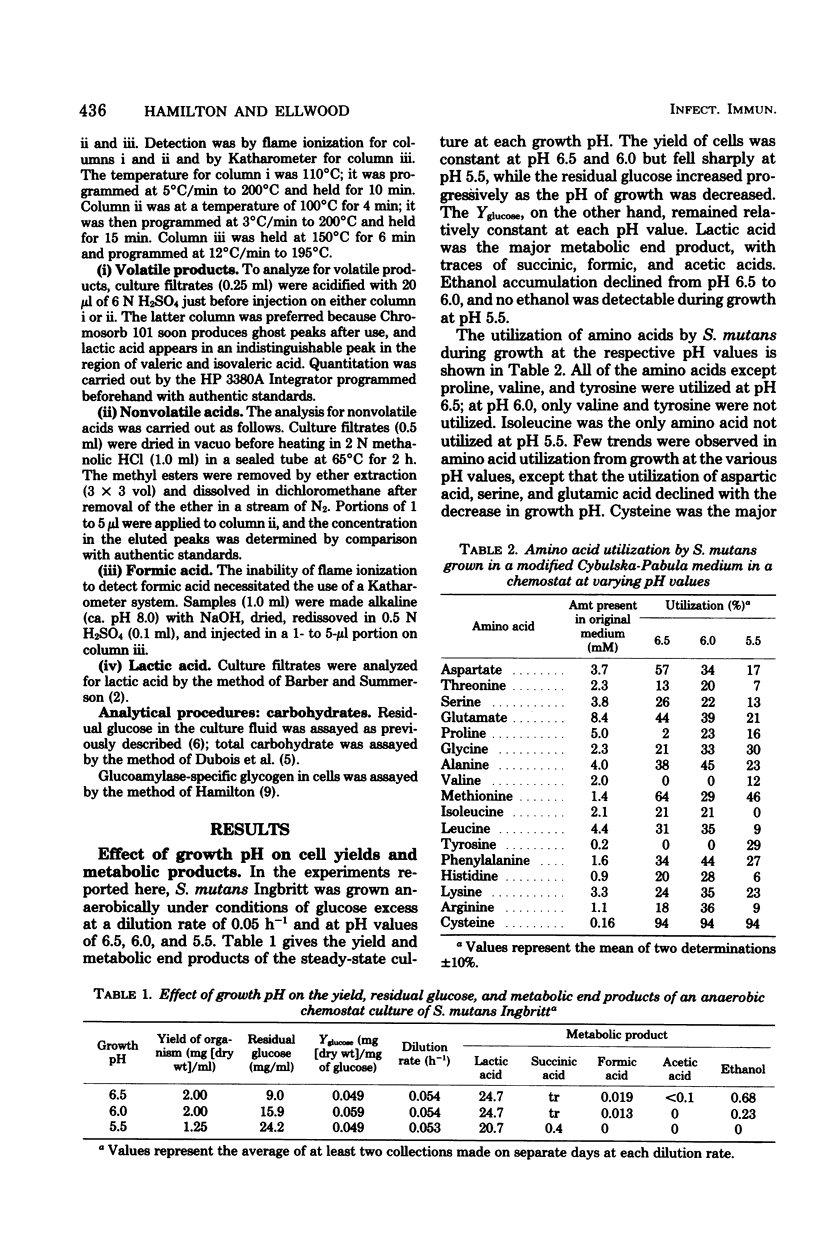
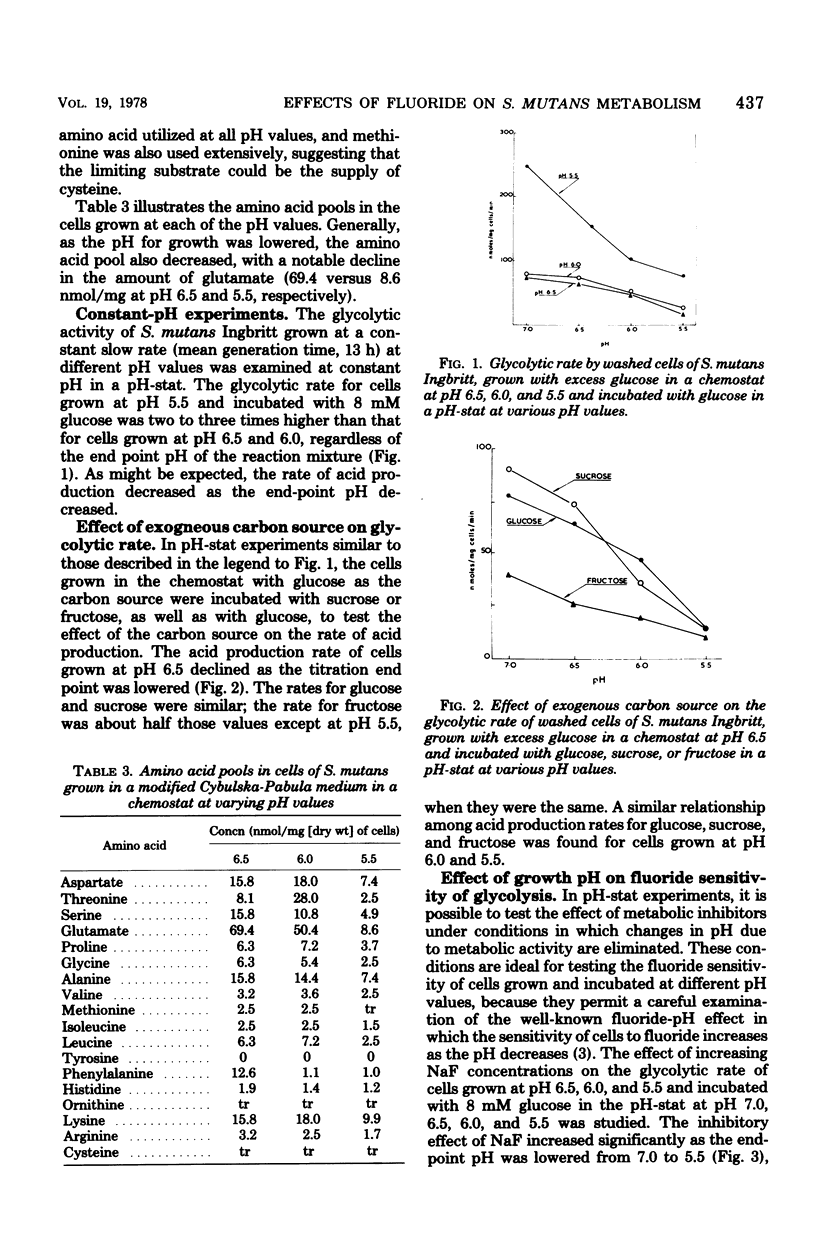
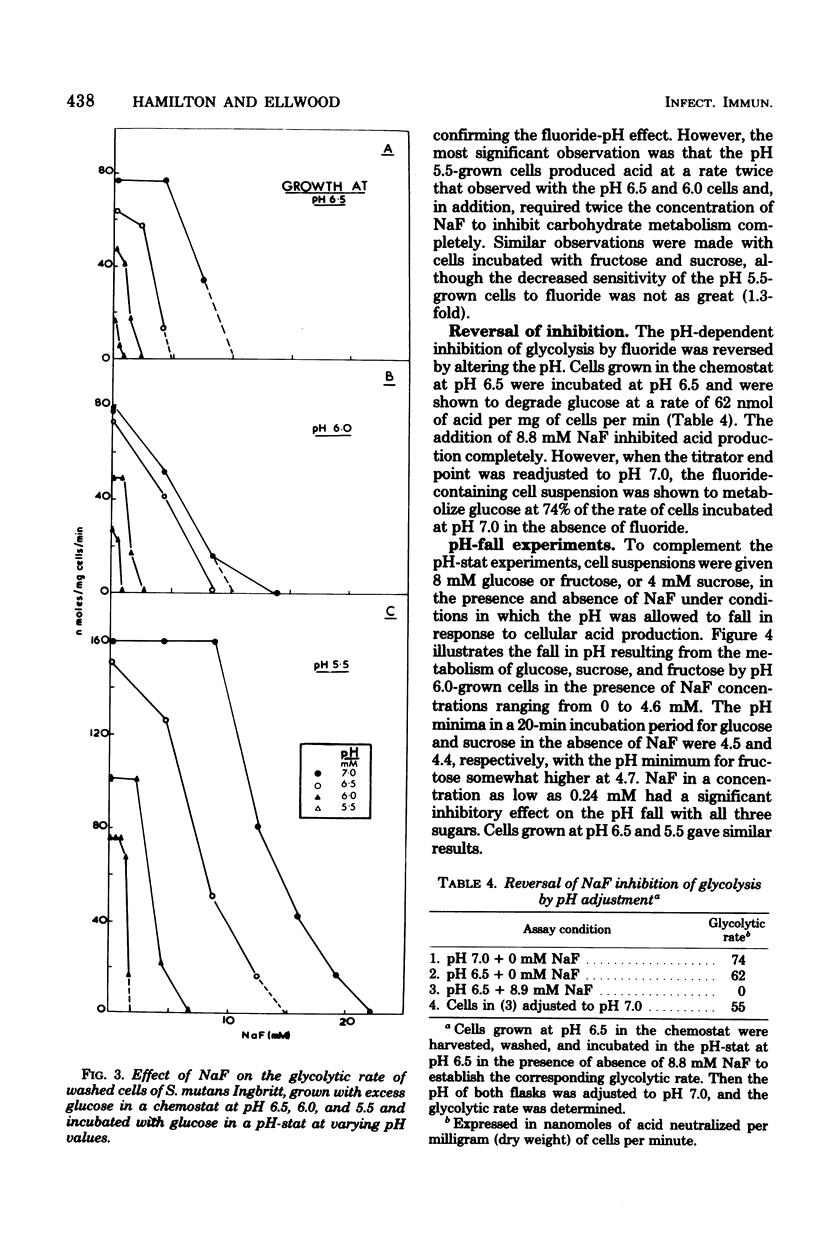
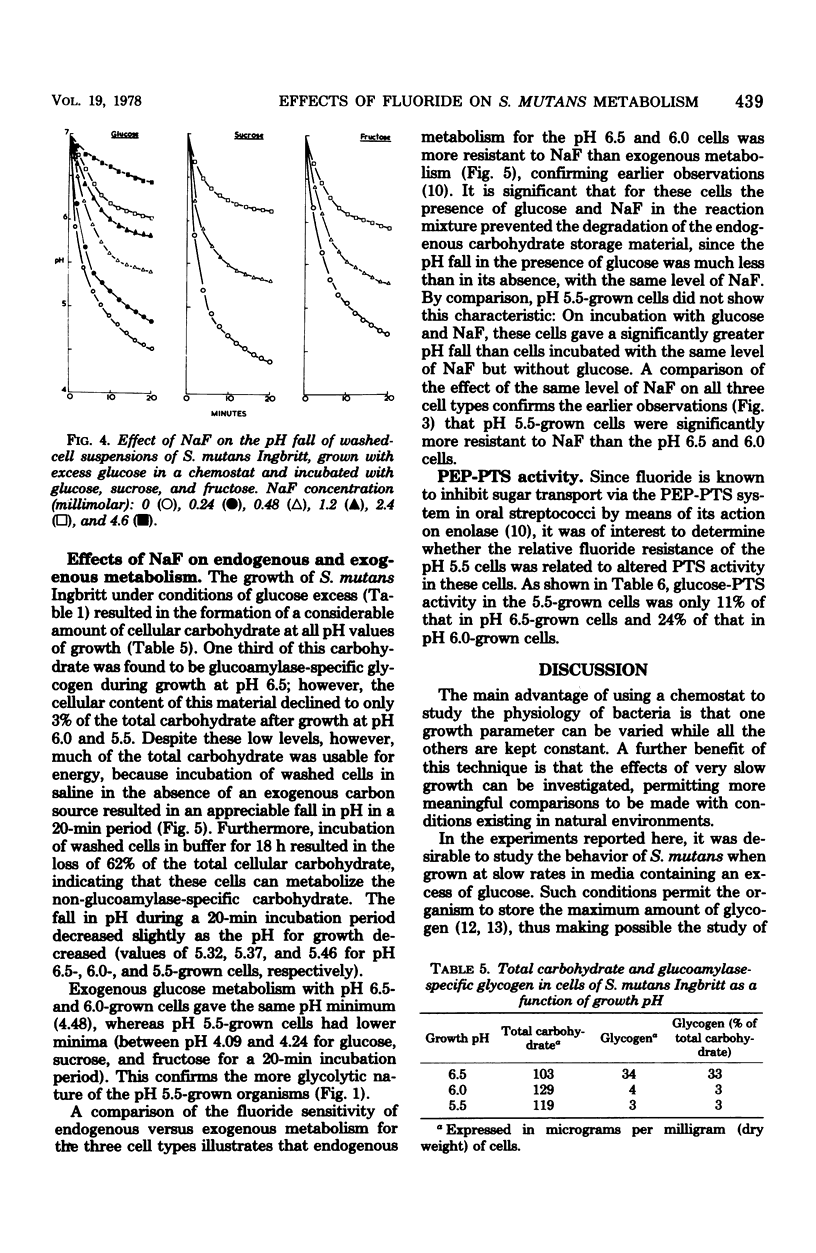
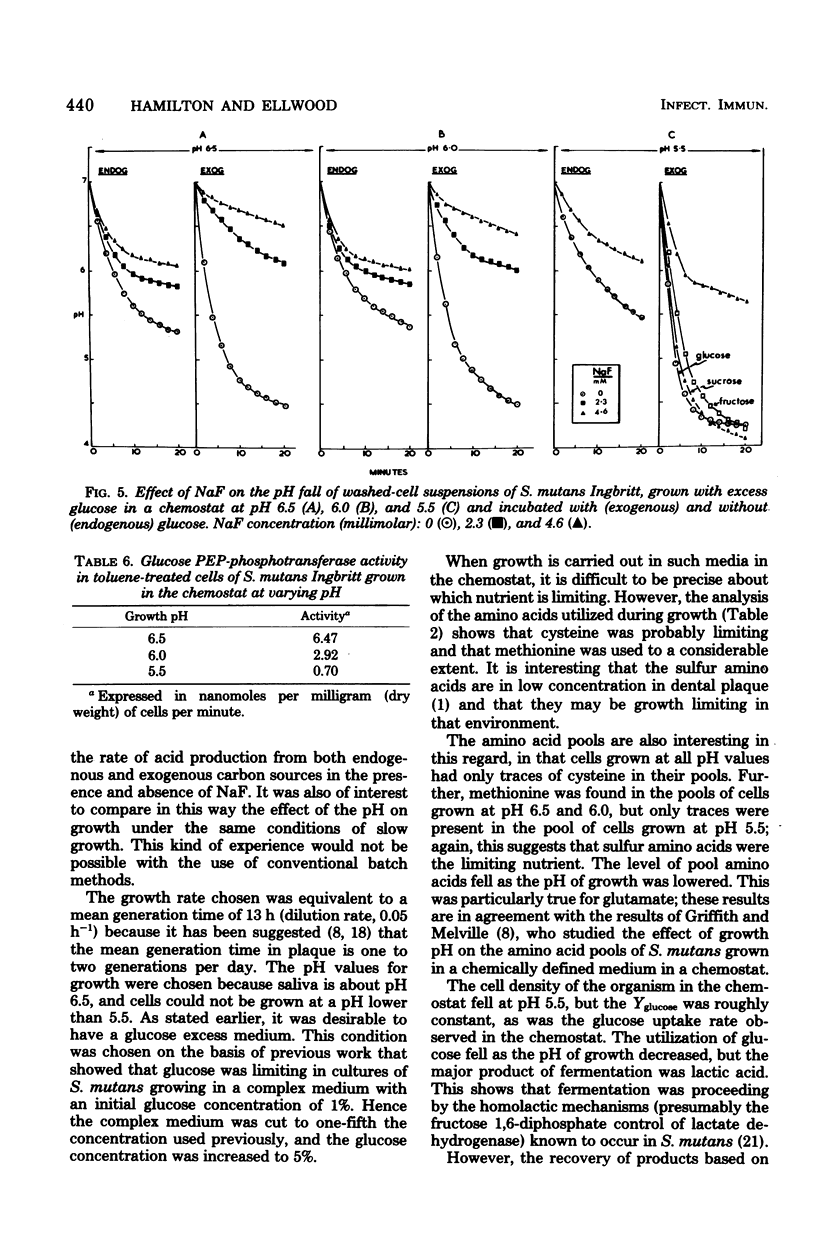
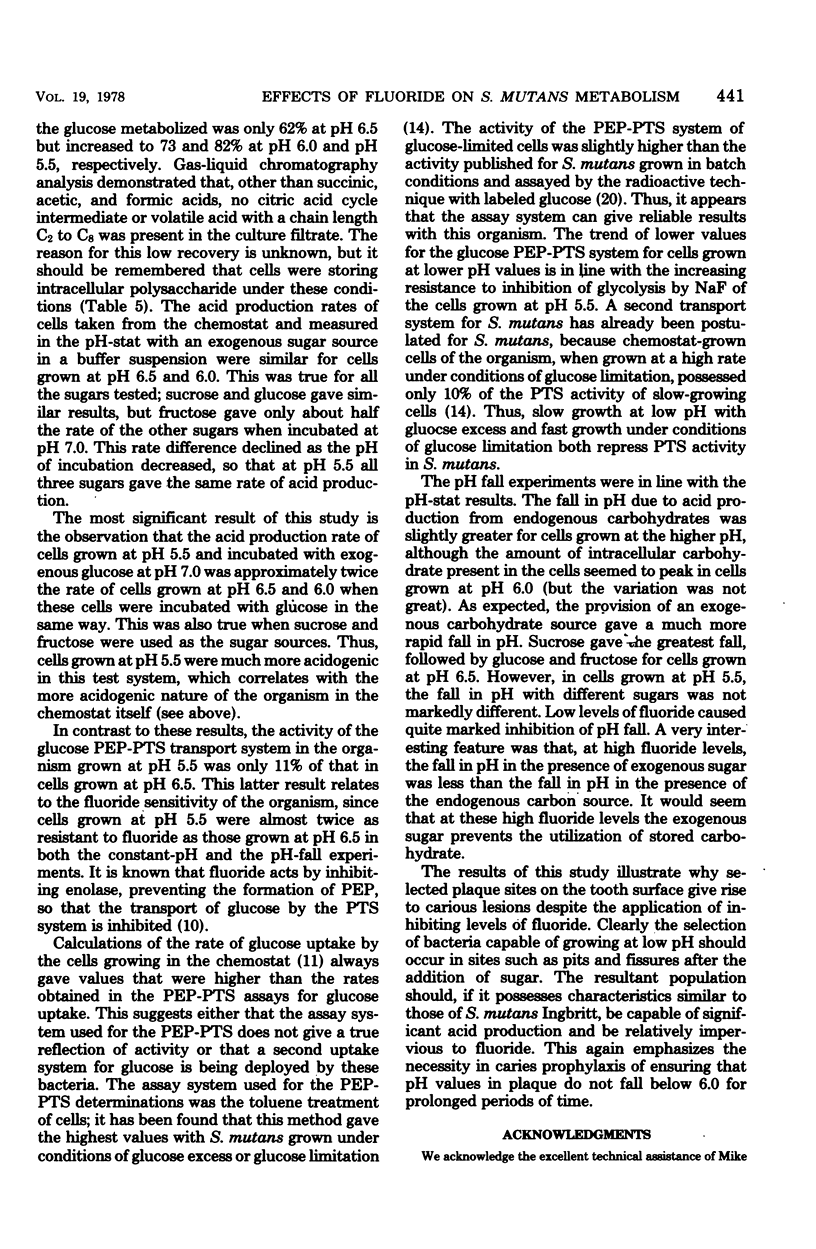
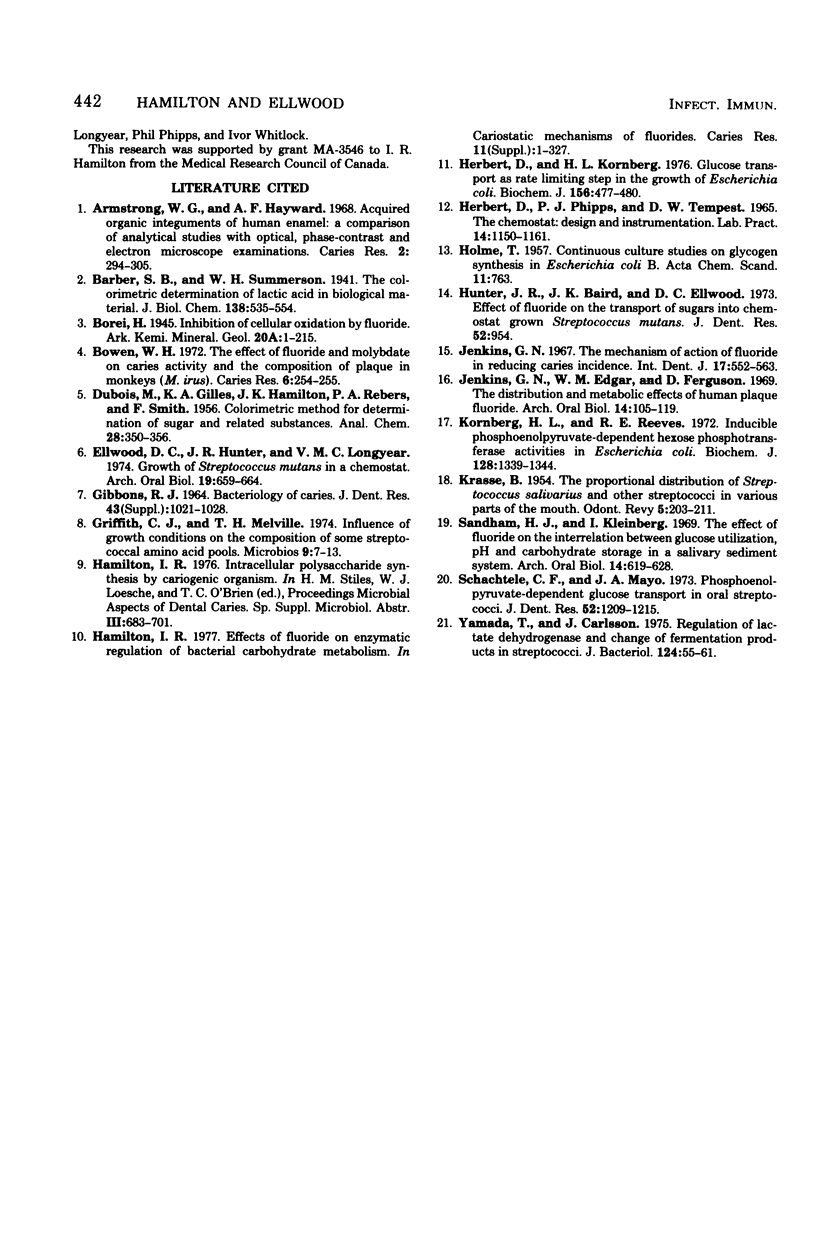
Selected References
These references are in PubMed. This may not be the complete list of references from this article.
- Armstrong W. G., Hayward A. F. Acquired organic integuments of human enamel: a comparison of analytical studies with optical, phase-contrast and electron microscope examinations. Caries Res. 1968;2(4):294–305. doi: 10.1159/000259568. [DOI] [PubMed] [Google Scholar]
- Cowman R. A., Fitzgerald R. J., Perrella M. M., Cornell A. H. Human saliva as a nitrogen source for oral streptococci. Caries Res. 1977;11(1):1–8. doi: 10.1159/000260242. [DOI] [PubMed] [Google Scholar]
- Ellwood D. C., Hunter J. R., Longyear V. M. Growth of Streptococcus mutans in a chemostat. Arch Oral Biol. 1974 Aug;19(8):659–664. doi: 10.1016/0003-9969(74)90134-4. [DOI] [PubMed] [Google Scholar]
- GIBBONS R. J. BACTERIOLOGY OF DENTAL CARIES. J Dent Res. 1964 Nov-Dec;43:SUPPL–SUPPL:1028. doi: 10.1177/00220345640430060301. [DOI] [PubMed] [Google Scholar]
- Griffith C. J., Melville T. H. Influence of growth conditions on the composition of some streptococcal amino acid pools. Microbios. 1974 Jan;9(33):7–13. [PubMed] [Google Scholar]
- Herbert D., Kornberg H. L. Glucose transport as rate-limiting step in the growth of Escherichia coli on glucose. Biochem J. 1976 May 15;156(2):477–480. doi: 10.1042/bj1560477. [DOI] [PMC free article] [PubMed] [Google Scholar]
- Herbert D., Phipps P. J., Tempest D. W. The chemostat: design and instrumentation. Lab Pract. 1965 Oct;14(10):1150–1161. [PubMed] [Google Scholar]
- Jenkins G. N., Edgar W. M. The distribution and metabolic effects of human plaque fluorine. Arch Oral Biol. 1969 Jan;14(1):105–119. doi: 10.1016/0003-9969(69)90025-9. [DOI] [PubMed] [Google Scholar]
- Jenkins G. N. The mechanism of action of fluoride in reducing caries incidence. Int Dent J. 1967 Sep;17(3):552–563. [PubMed] [Google Scholar]
- KRASSE B. The proportional distribution of Streptococcus salivarius and other streptococci in various parts of the mouth. Odontol Revy. 1954;5(3):203–211. [PubMed] [Google Scholar]
- Kornberg H. L., Reeves R. E. Inducible phosphoenolpyruvate-dependent hexose phosphotransferase activities in Escherichia coli. Biochem J. 1972 Aug;128(5):1339–1344. doi: 10.1042/bj1281339. [DOI] [PMC free article] [PubMed] [Google Scholar]
- Sandham H. J., Kleinberg I. The effect of fluoride on the interrelation between glucose utilization, pH and carbohydrate storage in a salivary sediment system. Arch Oral Biol. 1969 Jun;14(6):619–628. doi: 10.1016/0003-9969(69)90185-x. [DOI] [PubMed] [Google Scholar]
- Schachtele C. F., Mayo J. A. Phosphoenolpyruvate-dependent glucose transport in oral streptococci. J Dent Res. 1973 Nov-Dec;52(6):1209–1215. doi: 10.1177/00220345730520060801. [DOI] [PubMed] [Google Scholar]
- Yamada T., Carlsson J. Regulation of lactate dehydrogenase and change of fermentation products in streptococci. J Bacteriol. 1975 Oct;124(1):55–61. doi: 10.1128/jb.124.1.55-61.1975. [DOI] [PMC free article] [PubMed] [Google Scholar]