Abstract
Binding and signaling proteins based on Escherichia coli alkaline phosphatase (AP; EC 3.1.3.1) were designed for the detection of antibodies. Hybrid proteins were constructed by using wild-type AP and point mutants of AP [Asp-101 --> Ser (D101S) and Asp-153 --> Gly (D153G)]. The binding function of the hybrid proteins is provided by a peptide epitope inserted between amino acids 407 and 408 in AP. Binding of anti-epitope antibodies to the hybrid proteins modulates the enzyme activity of the hybrids; upon antibody binding, enzyme activity can increase to as much as 300% of the level of activity in the absence of antibody or can decrease as much as 40%, depending on the presence or absence of the point mutations in AP. The fact that modulation is altered from inhibition to activation by single amino acid changes in the active site of AP suggests that the mechanism for modulation is due to structural alterations upon antibody binding. Modulation is a general phenomenon. The properties of the system are demonstrated by using two epitopes, one from the V3 loop of human immunodeficiency virus type 1 gp120 protein and one from hepatitis C virus core protein, and corresponding monoclonal antibodies. The trend of modulation is consistent for all hybrids; those in wild-type AP are inhibited by antibody, while those in the AP mutants are activated by antibody. This demonstrates that modulation of enzyme activity of the AP-epitope hybrid proteins is not specific to either a particular epitope sequence or a particular antibody-epitope combination.
Full text
PDF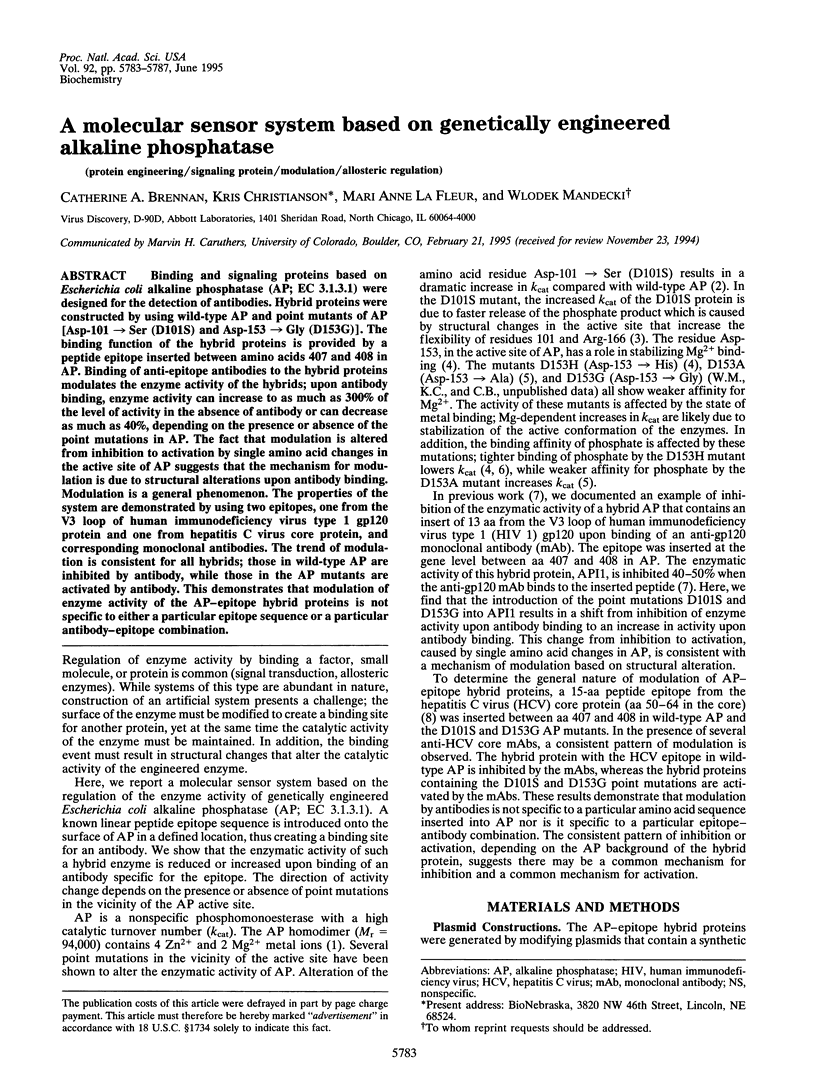
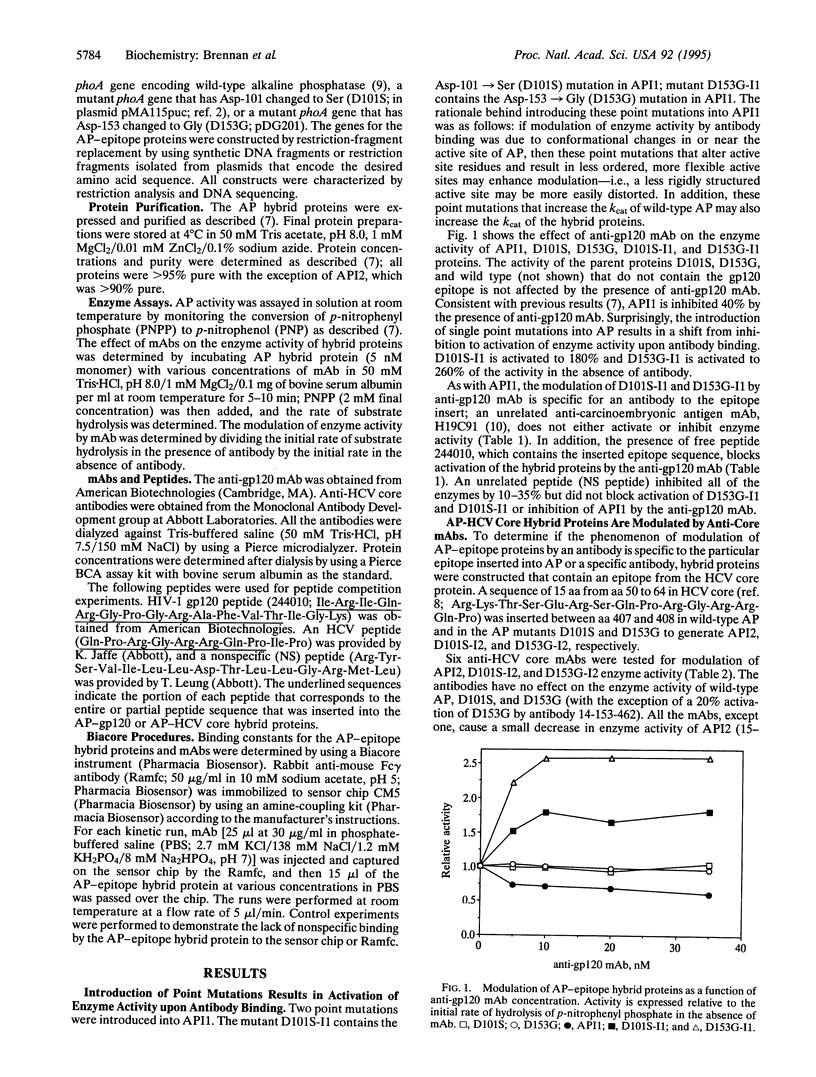
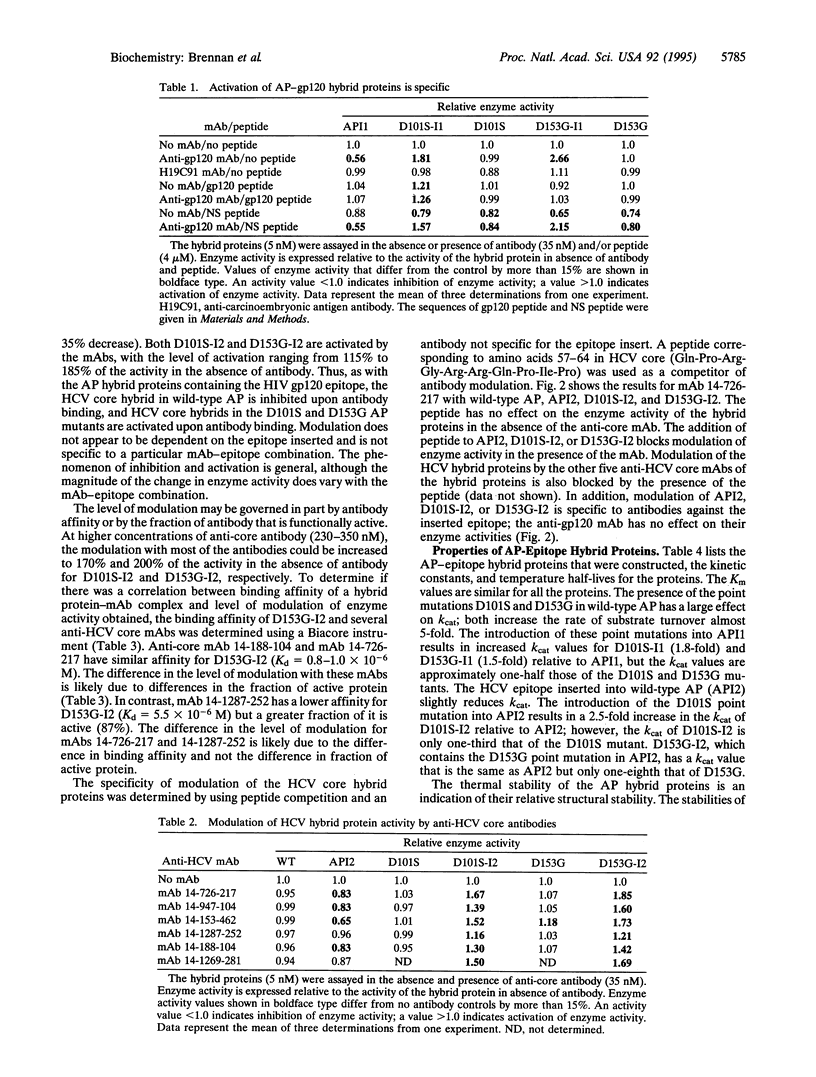
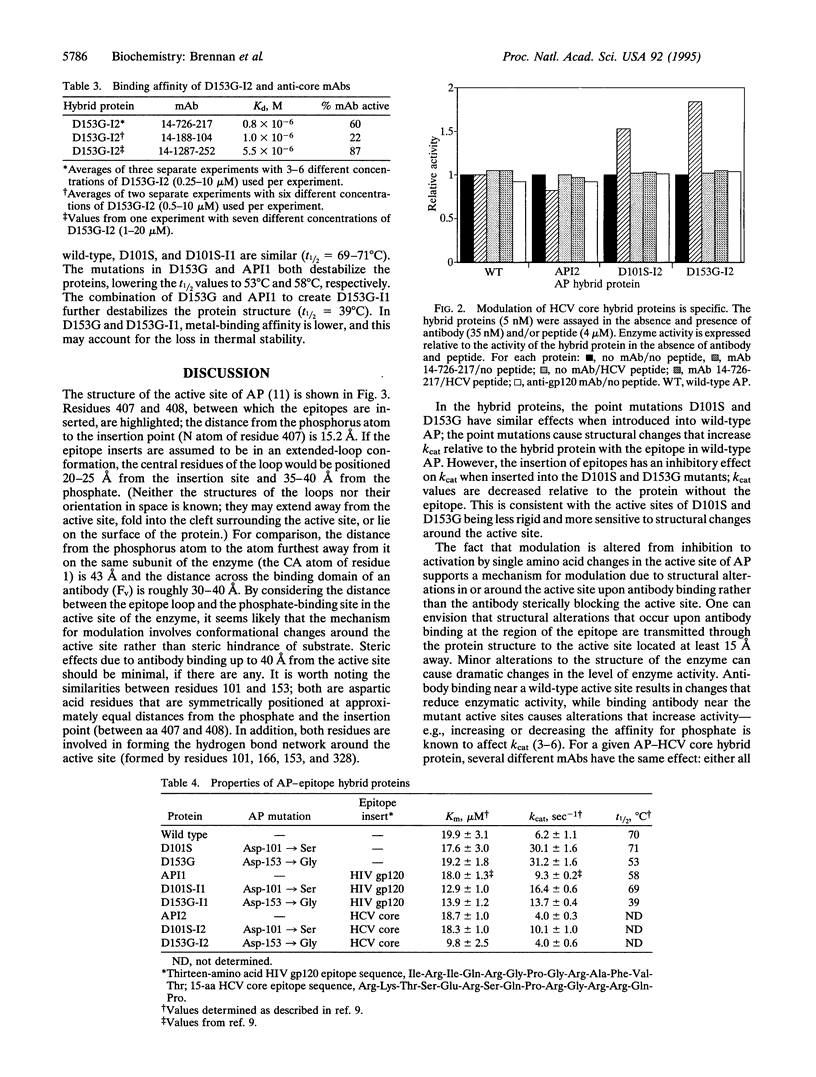
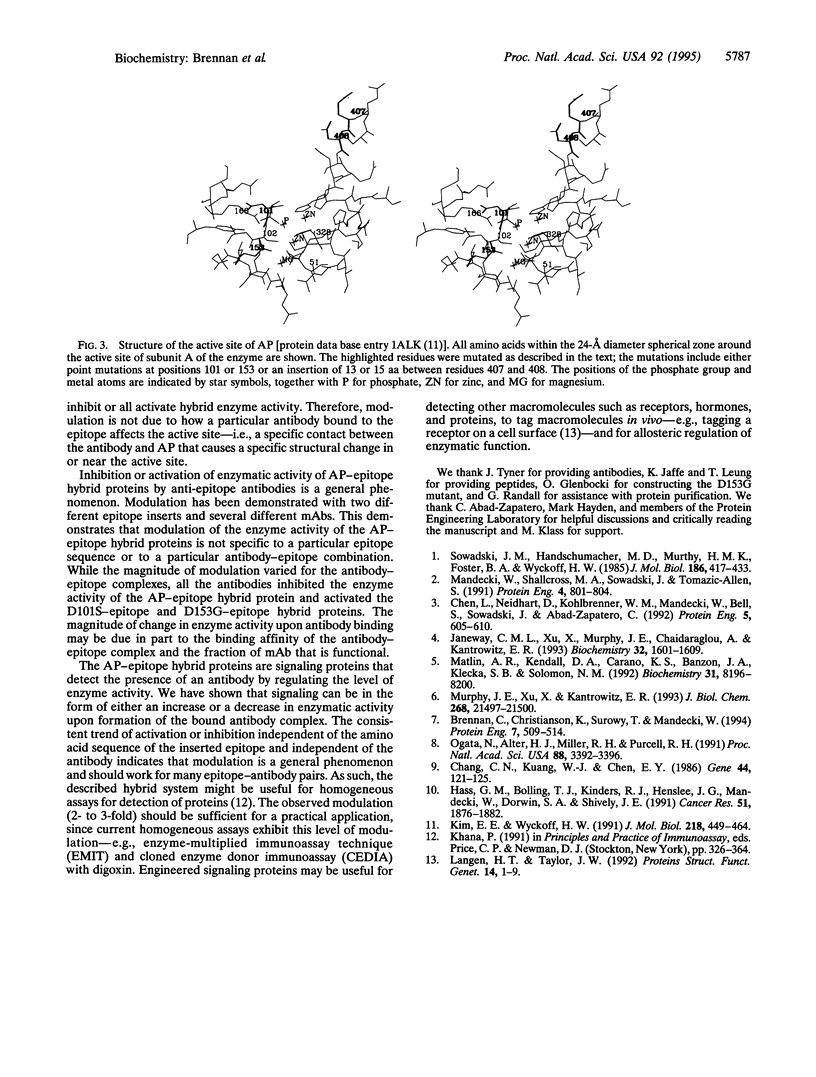
Selected References
These references are in PubMed. This may not be the complete list of references from this article.
- Brennan C., Christianson K., Surowy T., Mandecki W. Modulation of enzyme activity by antibody binding to an alkaline phosphatase-epitope hybrid protein. Protein Eng. 1994 Apr;7(4):509–514. doi: 10.1093/protein/7.4.509. [DOI] [PubMed] [Google Scholar]
- Chang C. N., Kuang W. J., Chen E. Y. Nucleotide sequence of the alkaline phosphatase gene of Escherichia coli. Gene. 1986;44(1):121–125. doi: 10.1016/0378-1119(86)90050-8. [DOI] [PubMed] [Google Scholar]
- Chen L., Neidhart D., Kohlbrenner W. M., Mandecki W., Bell S., Sowadski J., Abad-Zapatero C. 3-D structure of a mutant (Asp101-->Ser) of E.coli alkaline phosphatase with higher catalytic activity. Protein Eng. 1992 Oct;5(7):605–610. doi: 10.1093/protein/5.7.605. [DOI] [PubMed] [Google Scholar]
- Hass G. M., Bolling T. J., Kinders R. J., Henslee J. G., Mandecki W., Dorwin S. A., Shively J. E. Preparation of synthetic polypeptide domains of carcinoembryonic antigen and their use in epitope mapping. Cancer Res. 1991 Apr 1;51(7):1876–1882. [PubMed] [Google Scholar]
- Janeway C. M., Xu X., Murphy J. E., Chaidaroglou A., Kantrowitz E. R. Magnesium in the active site of Escherichia coli alkaline phosphatase is important for both structural stabilization and catalysis. Biochemistry. 1993 Feb 16;32(6):1601–1609. doi: 10.1021/bi00057a026. [DOI] [PubMed] [Google Scholar]
- Kim E. E., Wyckoff H. W. Reaction mechanism of alkaline phosphatase based on crystal structures. Two-metal ion catalysis. J Mol Biol. 1991 Mar 20;218(2):449–464. doi: 10.1016/0022-2836(91)90724-k. [DOI] [PubMed] [Google Scholar]
- Mandecki W., Shallcross M. A., Sowadski J., Tomazic-Allen S. Mutagenesis of conserved residues within the active site of Escherichia coli alkaline phosphatase yields enzymes with increased kcat. Protein Eng. 1991 Oct;4(7):801–804. doi: 10.1093/protein/4.7.801. [DOI] [PubMed] [Google Scholar]
- Matlin A. R., Kendall D. A., Carano K. S., Banzon J. A., Klecka S. B., Solomon N. M. Enhanced catalysis by active-site mutagenesis at aspartic acid 153 in Escherichia coli alkaline phosphatase. Biochemistry. 1992 Sep 8;31(35):8196–8200. doi: 10.1021/bi00150a011. [DOI] [PubMed] [Google Scholar]
- Murphy J. E., Xu X., Kantrowitz E. R. Conversion of a magnesium binding site into a zinc binding site by a single amino acid substitution in Escherichia coli alkaline phosphatase. J Biol Chem. 1993 Oct 15;268(29):21497–21500. doi: 10.2210/pdb1anh/pdb. [DOI] [PubMed] [Google Scholar]
- Ogata N., Alter H. J., Miller R. H., Purcell R. H. Nucleotide sequence and mutation rate of the H strain of hepatitis C virus. Proc Natl Acad Sci U S A. 1991 Apr 15;88(8):3392–3396. doi: 10.1073/pnas.88.8.3392. [DOI] [PMC free article] [PubMed] [Google Scholar]
- Sowadski J. M., Handschumacher M. D., Murthy H. M., Foster B. A., Wyckoff H. W. Refined structure of alkaline phosphatase from Escherichia coli at 2.8 A resolution. J Mol Biol. 1985 Nov 20;186(2):417–433. doi: 10.1016/0022-2836(85)90115-9. [DOI] [PubMed] [Google Scholar]