Abstract
Motor domains of the Drosophila minus-end-directed microtubule (MT) motor protein ncd, were found to saturate microtubule binding sites at a stoichiometry of approximately one motor domain per tubulin dimer. To determine the tubulin subunit(s) involved in binding to ncd, mixtures of ncd motor domain and MTs were treated with the zero-length cross-linker 1-ethyl-3-(3-dimethylaminopropyl-carbodiimide) (EDC). EDC treatment generated covalently cross-linked products of ncd and alpha-tubulin and of ncd and beta-tubulin, indicating that the ncd motor domain interacts with both alpha- and beta-tubulin. When the Drosophila kinesin motor domain protein was substituted for the ncd motor domain, cross-linked products of kinesin and alpha-tubulin and of kinesin and beta-tubulin were produced. EDC treatment of mixtures of ncd motor domain and unassembled tubulin dimers or of kinesin motor domain and unassembled tubulin dimers produced the same motor-tubulin products generated in the presence of MTs. These results indicate that kinesin family motors of opposite polarity interact with both tubulin monomers and support a model in which some portion of each protein's motor domain overlaps adjacent alpha- and beta-tubulin subunits.
Full text
PDF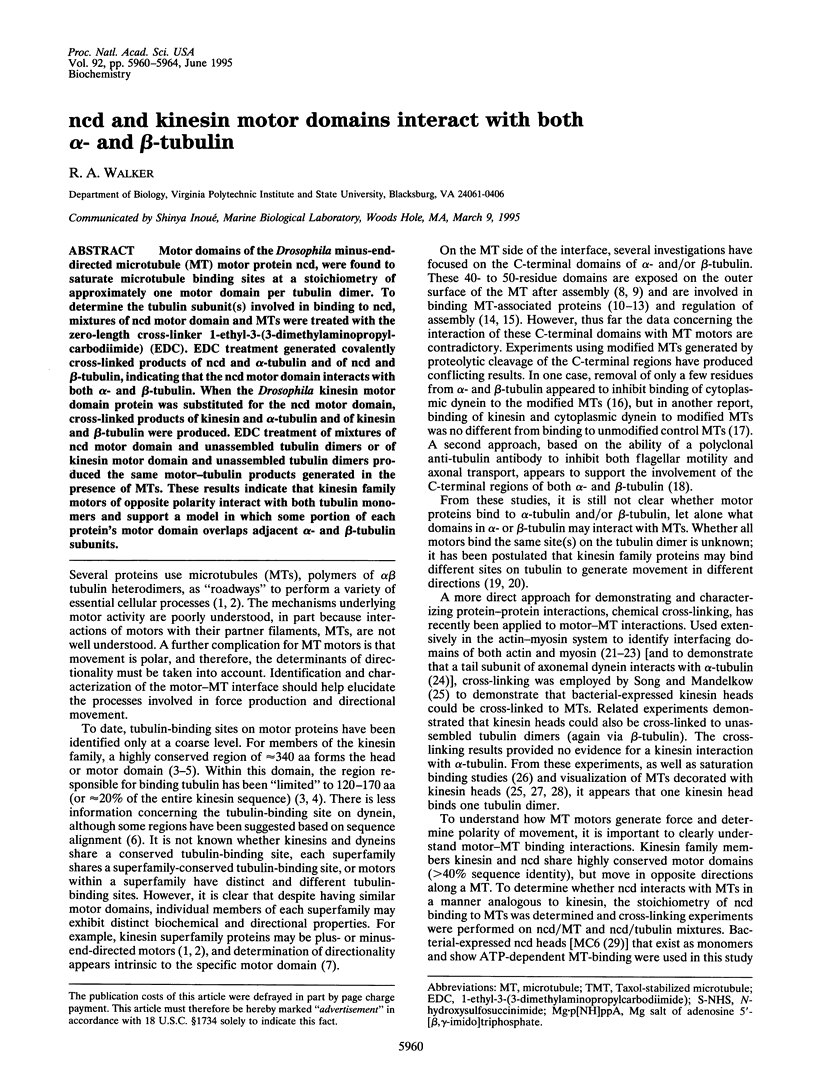
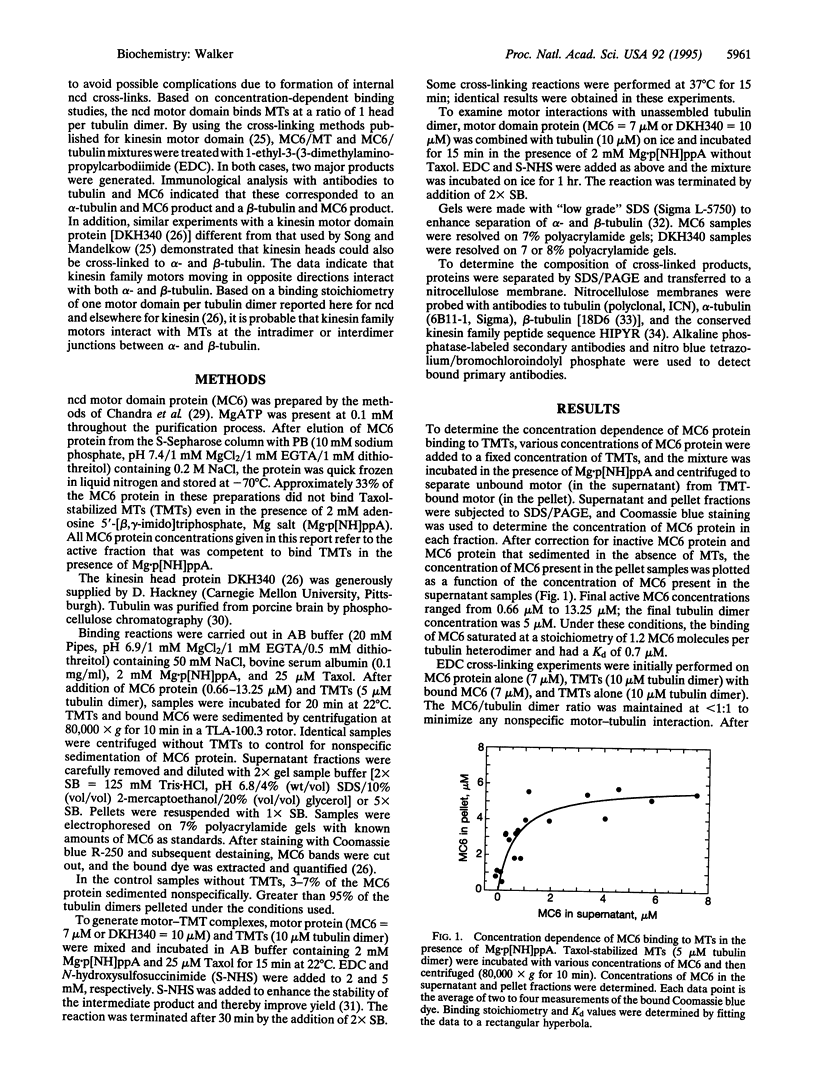
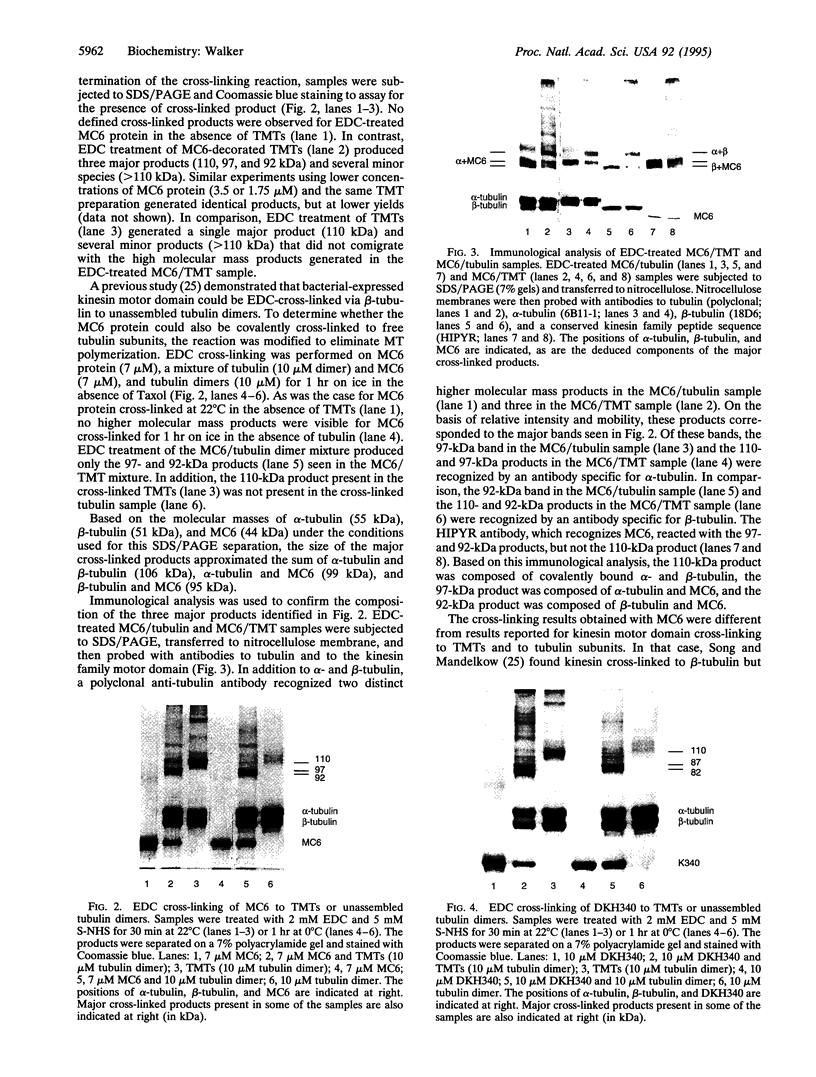
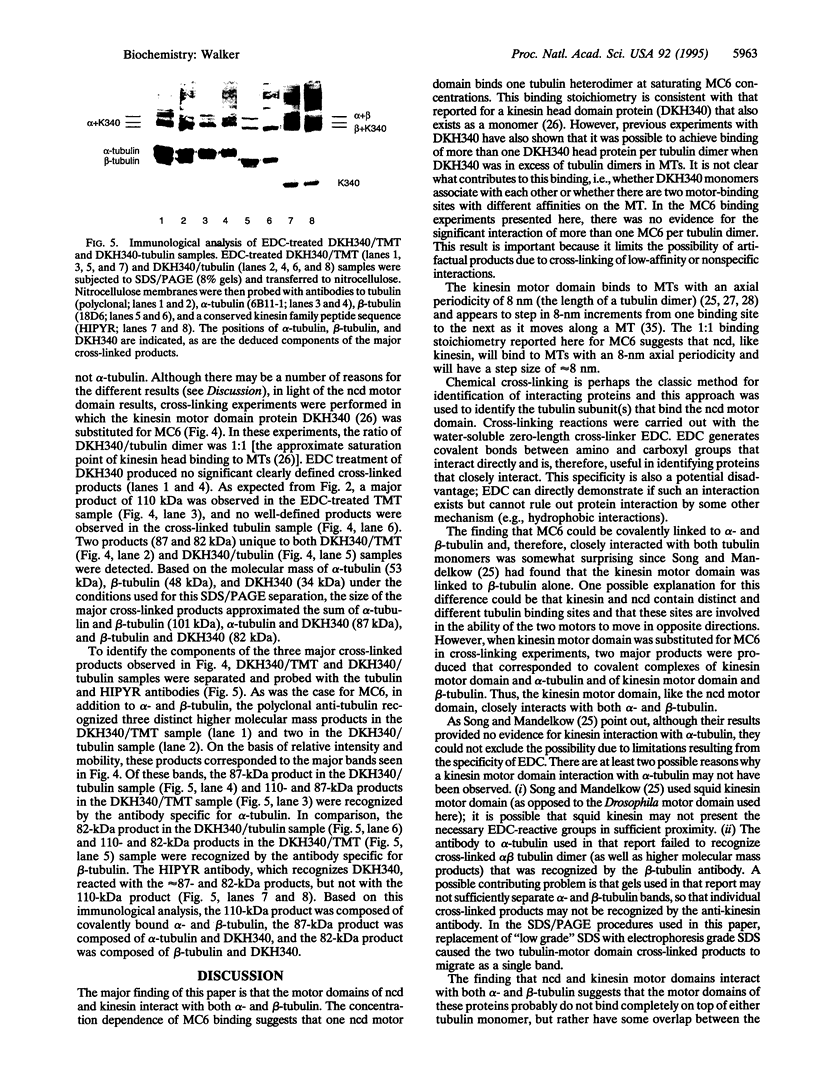
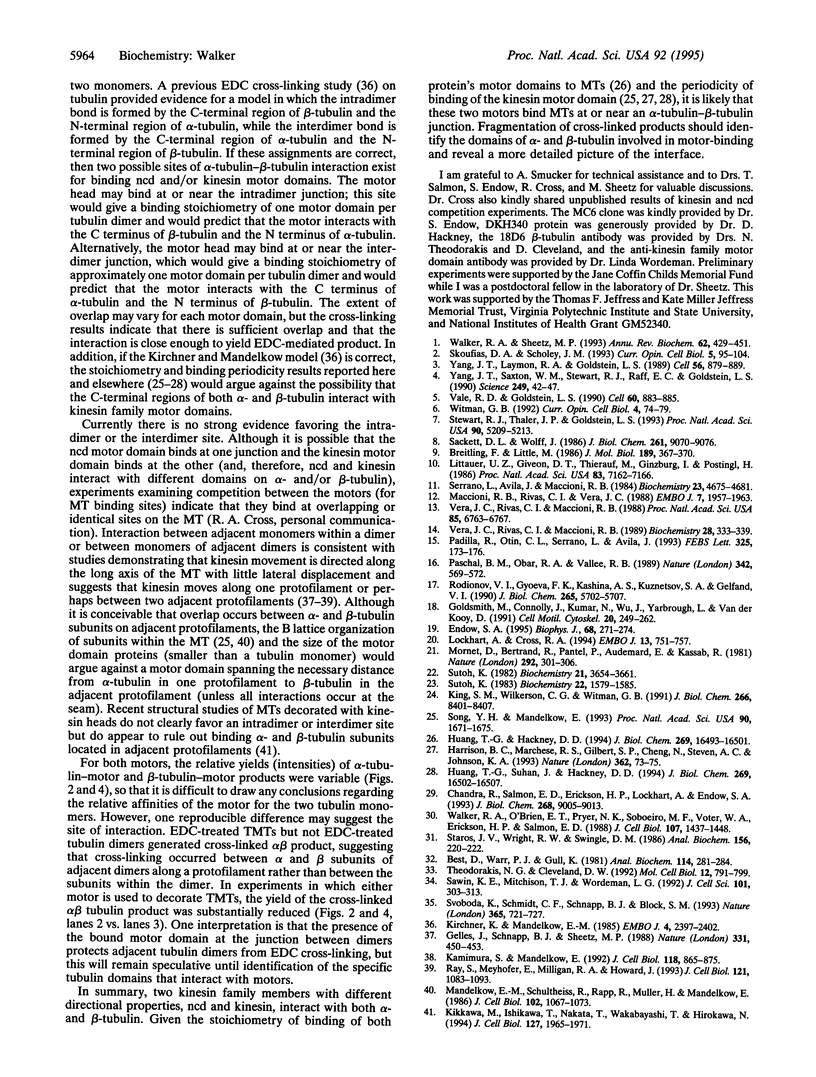
Images in this article
Selected References
These references are in PubMed. This may not be the complete list of references from this article.
- Best D., Warr P. J., Gull K. Influence of the composition of commercial sodium dodecyl sulfate preparations on the separation of alpha- and beta-tubulin during polyacrylamide gel electrophoresis. Anal Biochem. 1981 Jul 1;114(2):281–284. doi: 10.1016/0003-2697(81)90481-4. [DOI] [PubMed] [Google Scholar]
- Breitling F., Little M. Carboxy-terminal regions on the surface of tubulin and microtubules. Epitope locations of YOL1/34, DM1A and DM1B. J Mol Biol. 1986 May 20;189(2):367–370. doi: 10.1016/0022-2836(86)90517-6. [DOI] [PubMed] [Google Scholar]
- Chandra R., Salmon E. D., Erickson H. P., Lockhart A., Endow S. A. Structural and functional domains of the Drosophila ncd microtubule motor protein. J Biol Chem. 1993 Apr 25;268(12):9005–9013. [PubMed] [Google Scholar]
- Gelles J., Schnapp B. J., Sheetz M. P. Tracking kinesin-driven movements with nanometre-scale precision. Nature. 1988 Feb 4;331(6155):450–453. doi: 10.1038/331450a0. [DOI] [PubMed] [Google Scholar]
- Goldsmith M., Connolly J. A., Kumar N., Wu J., Yarbrough L. R., van der Kooy D. Conserved beta-tubulin binding domain for the microtubule-associated motors underlying sperm motility and fast axonal transport. Cell Motil Cytoskeleton. 1991;20(3):249–262. doi: 10.1002/cm.970200308. [DOI] [PubMed] [Google Scholar]
- Harrison B. C., Marchese-Ragona S. P., Gilbert S. P., Cheng N., Steven A. C., Johnson K. A. Decoration of the microtubule surface by one kinesin head per tubulin heterodimer. Nature. 1993 Mar 4;362(6415):73–75. doi: 10.1038/362073a0. [DOI] [PubMed] [Google Scholar]
- Huang T. G., Hackney D. D. Drosophila kinesin minimal motor domain expressed in Escherichia coli. Purification and kinetic characterization. J Biol Chem. 1994 Jun 10;269(23):16493–16501. [PubMed] [Google Scholar]
- Huang T. G., Suhan J., Hackney D. D. Drosophila kinesin motor domain extending to amino acid position 392 is dimeric when expressed in Escherichia coli. J Biol Chem. 1994 Jun 10;269(23):16502–16507. [PubMed] [Google Scholar]
- Kamimura S., Mandelkow E. Tubulin protofilaments and kinesin-dependent motility. J Cell Biol. 1992 Aug;118(4):865–875. doi: 10.1083/jcb.118.4.865. [DOI] [PMC free article] [PubMed] [Google Scholar]
- Kikkawa M., Ishikawa T., Nakata T., Wakabayashi T., Hirokawa N. Direct visualization of the microtubule lattice seam both in vitro and in vivo. J Cell Biol. 1994 Dec;127(6 Pt 2):1965–1971. doi: 10.1083/jcb.127.6.1965. [DOI] [PMC free article] [PubMed] [Google Scholar]
- King S. M., Wilkerson C. G., Witman G. B. The Mr 78,000 intermediate chain of Chlamydomonas outer arm dynein interacts with alpha-tubulin in situ. J Biol Chem. 1991 May 5;266(13):8401–8407. [PubMed] [Google Scholar]
- Kirchner K., Mandelkow E. M. Tubulin domains responsible for assembly of dimers and protofilaments. EMBO J. 1985 Sep;4(9):2397–2402. doi: 10.1002/j.1460-2075.1985.tb03945.x. [DOI] [PMC free article] [PubMed] [Google Scholar]
- Littauer U. Z., Giveon D., Thierauf M., Ginzburg I., Ponstingl H. Common and distinct tubulin binding sites for microtubule-associated proteins. Proc Natl Acad Sci U S A. 1986 Oct;83(19):7162–7166. doi: 10.1073/pnas.83.19.7162. [DOI] [PMC free article] [PubMed] [Google Scholar]
- Lockhart A., Cross R. A. Origins of reversed directionality in the ncd molecular motor. EMBO J. 1994 Feb 15;13(4):751–757. doi: 10.1002/j.1460-2075.1994.tb06317.x. [DOI] [PMC free article] [PubMed] [Google Scholar]
- Maccioni R. B., Rivas C. I., Vera J. C. Differential interaction of synthetic peptides from the carboxyl-terminal regulatory domain of tubulin with microtubule-associated proteins. EMBO J. 1988 Jul;7(7):1957–1963. doi: 10.1002/j.1460-2075.1988.tb03033.x. [DOI] [PMC free article] [PubMed] [Google Scholar]
- Mandelkow E. M., Schultheiss R., Rapp R., Müller M., Mandelkow E. On the surface lattice of microtubules: helix starts, protofilament number, seam, and handedness. J Cell Biol. 1986 Mar;102(3):1067–1073. doi: 10.1083/jcb.102.3.1067. [DOI] [PMC free article] [PubMed] [Google Scholar]
- Mornet D., Bertrand R., Pantel P., Audemard E., Kassab R. Structure of the actin-myosin interface. Nature. 1981 Jul 23;292(5821):301–306. doi: 10.1038/292301a0. [DOI] [PubMed] [Google Scholar]
- Padilla R., López Otin C., Serrano L., Avila J. Role of the carboxy terminal region of beta tubulin on microtubule dynamics through its interaction with the GTP phosphate binding region. FEBS Lett. 1993 Jul 5;325(3):173–176. doi: 10.1016/0014-5793(93)81067-a. [DOI] [PubMed] [Google Scholar]
- Paschal B. M., Obar R. A., Vallee R. B. Interaction of brain cytoplasmic dynein and MAP2 with a common sequence at the C terminus of tubulin. Nature. 1989 Nov 30;342(6249):569–572. doi: 10.1038/342569a0. [DOI] [PubMed] [Google Scholar]
- Ray S., Meyhöfer E., Milligan R. A., Howard J. Kinesin follows the microtubule's protofilament axis. J Cell Biol. 1993 Jun;121(5):1083–1093. doi: 10.1083/jcb.121.5.1083. [DOI] [PMC free article] [PubMed] [Google Scholar]
- Rodionov V. I., Gyoeva F. K., Kashina A. S., Kuznetsov S. A., Gelfand V. I. Microtubule-associated proteins and microtubule-based translocators have different binding sites on tubulin molecule. J Biol Chem. 1990 Apr 5;265(10):5702–5707. [PubMed] [Google Scholar]
- Sackett D. L., Wolff J. Proteolysis of tubulin and the substructure of the tubulin dimer. J Biol Chem. 1986 Jul 5;261(19):9070–9076. [PubMed] [Google Scholar]
- Sawin K. E., Mitchison T. J., Wordeman L. G. Evidence for kinesin-related proteins in the mitotic apparatus using peptide antibodies. J Cell Sci. 1992 Feb;101(Pt 2):303–313. doi: 10.1242/jcs.101.2.303. [DOI] [PubMed] [Google Scholar]
- Serrano L., Avila J., Maccioni R. B. Controlled proteolysis of tubulin by subtilisin: localization of the site for MAP2 interaction. Biochemistry. 1984 Sep 25;23(20):4675–4681. doi: 10.1021/bi00315a024. [DOI] [PubMed] [Google Scholar]
- Skoufias D. A., Scholey J. M. Cytoplasmic microtubule-based motor proteins. Curr Opin Cell Biol. 1993 Feb;5(1):95–104. doi: 10.1016/s0955-0674(05)80014-6. [DOI] [PubMed] [Google Scholar]
- Song Y. H., Mandelkow E. Recombinant kinesin motor domain binds to beta-tubulin and decorates microtubules with a B surface lattice. Proc Natl Acad Sci U S A. 1993 Mar 1;90(5):1671–1675. doi: 10.1073/pnas.90.5.1671. [DOI] [PMC free article] [PubMed] [Google Scholar]
- Staros J. V., Wright R. W., Swingle D. M. Enhancement by N-hydroxysulfosuccinimide of water-soluble carbodiimide-mediated coupling reactions. Anal Biochem. 1986 Jul;156(1):220–222. doi: 10.1016/0003-2697(86)90176-4. [DOI] [PubMed] [Google Scholar]
- Stewart R. J., Thaler J. P., Goldstein L. S. Direction of microtubule movement is an intrinsic property of the motor domains of kinesin heavy chain and Drosophila ncd protein. Proc Natl Acad Sci U S A. 1993 Jun 1;90(11):5209–5213. doi: 10.1073/pnas.90.11.5209. [DOI] [PMC free article] [PubMed] [Google Scholar]
- Sutoh K. Identification of myosin-binding sites on the actin sequence. Biochemistry. 1982 Jul 20;21(15):3654–3661. doi: 10.1021/bi00258a020. [DOI] [PubMed] [Google Scholar]
- Sutoh K. Mapping of actin-binding sites on the heavy chain of myosin subfragment 1. Biochemistry. 1983 Mar 29;22(7):1579–1585. doi: 10.1021/bi00276a009. [DOI] [PubMed] [Google Scholar]
- Svoboda K., Schmidt C. F., Schnapp B. J., Block S. M. Direct observation of kinesin stepping by optical trapping interferometry. Nature. 1993 Oct 21;365(6448):721–727. doi: 10.1038/365721a0. [DOI] [PubMed] [Google Scholar]
- Theodorakis N. G., Cleveland D. W. Physical evidence for cotranslational regulation of beta-tubulin mRNA degradation. Mol Cell Biol. 1992 Feb;12(2):791–799. doi: 10.1128/mcb.12.2.791. [DOI] [PMC free article] [PubMed] [Google Scholar]
- Vale R. D., Goldstein L. S. One motor, many tails: an expanding repertoire of force-generating enzymes. Cell. 1990 Mar 23;60(6):883–885. doi: 10.1016/0092-8674(90)90334-b. [DOI] [PubMed] [Google Scholar]
- Vera J. C., Rivas C. I., Maccioni R. B. Antibodies to synthetic peptides from the tubulin regulatory domain interact with tubulin and microtubules. Proc Natl Acad Sci U S A. 1988 Sep;85(18):6763–6767. doi: 10.1073/pnas.85.18.6763. [DOI] [PMC free article] [PubMed] [Google Scholar]
- Vera J. C., Rivas C. I., Maccioni R. B. Biochemical dissection of the role of the one-kilodalton carboxyl-terminal moiety of tubulin in its assembly into microtubules. Biochemistry. 1989 Jan 10;28(1):333–339. doi: 10.1021/bi00427a045. [DOI] [PubMed] [Google Scholar]
- Walker R. A., O'Brien E. T., Pryer N. K., Soboeiro M. F., Voter W. A., Erickson H. P., Salmon E. D. Dynamic instability of individual microtubules analyzed by video light microscopy: rate constants and transition frequencies. J Cell Biol. 1988 Oct;107(4):1437–1448. doi: 10.1083/jcb.107.4.1437. [DOI] [PMC free article] [PubMed] [Google Scholar]
- Walker R. A., Sheetz M. P. Cytoplasmic microtubule-associated motors. Annu Rev Biochem. 1993;62:429–451. doi: 10.1146/annurev.bi.62.070193.002241. [DOI] [PubMed] [Google Scholar]
- Witman G. B. Axonemal dyneins. Curr Opin Cell Biol. 1992 Feb;4(1):74–79. doi: 10.1016/0955-0674(92)90061-g. [DOI] [PubMed] [Google Scholar]
- Yang J. T., Laymon R. A., Goldstein L. S. A three-domain structure of kinesin heavy chain revealed by DNA sequence and microtubule binding analyses. Cell. 1989 Mar 10;56(5):879–889. doi: 10.1016/0092-8674(89)90692-2. [DOI] [PubMed] [Google Scholar]
- Yang J. T., Saxton W. M., Stewart R. J., Raff E. C., Goldstein L. S. Evidence that the head of kinesin is sufficient for force generation and motility in vitro. Science. 1990 Jul 6;249(4964):42–47. doi: 10.1126/science.2142332. [DOI] [PubMed] [Google Scholar]