Abstract
Extracellular superoxide was detected in cultures of monkey and human arterial smooth muscle cells as indicated by superoxide dismutase inhibitable reduction of cytochrome c. Superoxide production by these cells in the presence of Fe or Cu resulted in modification of low density lipoprotein (LDL). The degree of LDL modification was directly proportional to the rate of superoxide production by cells. Superoxide dismutase (100 micrograms/ml), and the general free radical scavengers butylated hydroxytoluene and butylated hydroxyanisole (50 microM), inhibited Fe- and Cu-mediated modification of LDL by monkey smooth muscle cells, while catalase (100 micrograms/ml) and mannitol (25 mM) had no effect. The chelators desferrioxamine and diethylenetriamine pentaacetic acid completely inhibited Fe- and Cu-promoted modification of LDL, while EGTA had no inhibitory effect. EDTA stimulated Fe-promoted modification in the 1-100 microM range while inhibiting Cu-mediated modification of LDL. LDL modified by smooth muscle cells in the presence of 10 microM Fe or Cu stimulated [14C]oleate incorporation into cholesteryl ester by human macrophages and murine J774 cells to a degree comparable to that produced by acetylated LDL. LDL incubated with smooth muscle cells and metal ions in the presence of superoxide dismutase failed to enhance macrophage cholesteryl ester accumulation. Thus, arterial smooth muscle cells in culture generate superoxide and modify LDL by a superoxide-dependent, Fe or Cu catalyzed free radical process, resulting in enhanced uptake of the modified LDL by macrophages. Neither hydroxyl radicals nor H2O2 are likely to be involved. Superoxide-dependent lipid peroxidation may contribute to biological modification of LDL, resulting in foam cell formation and atherogenesis.
Full text
PDF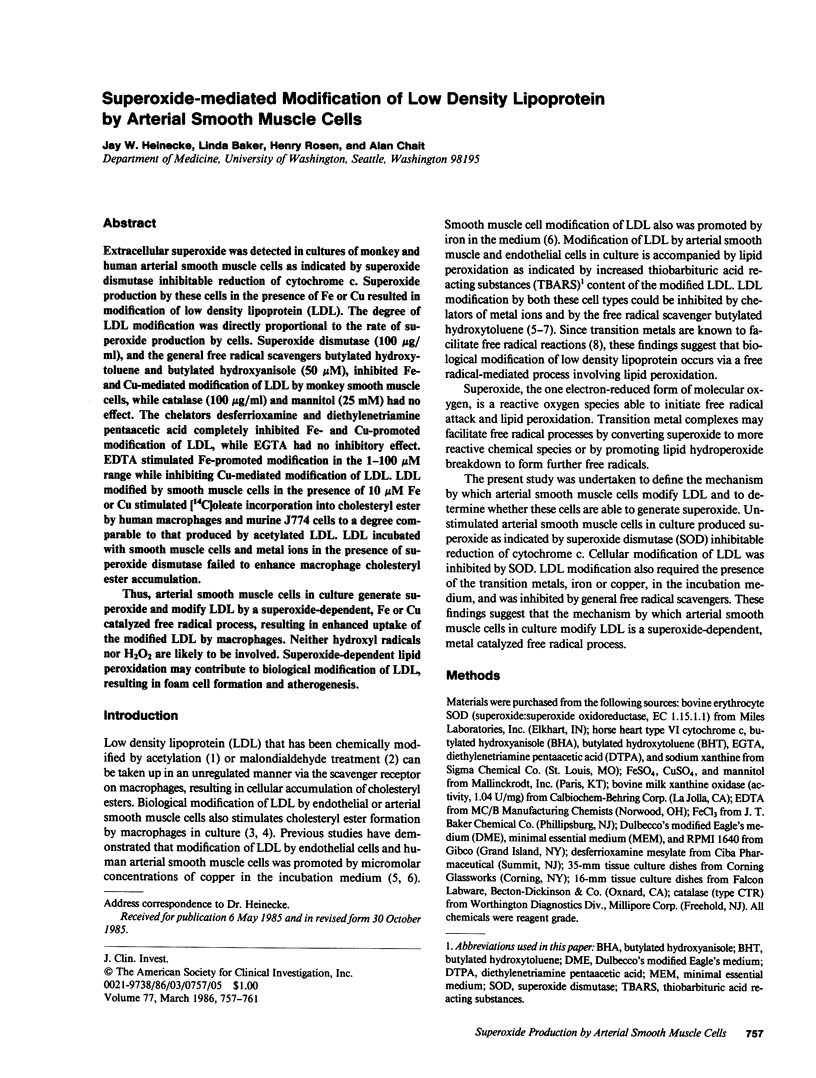
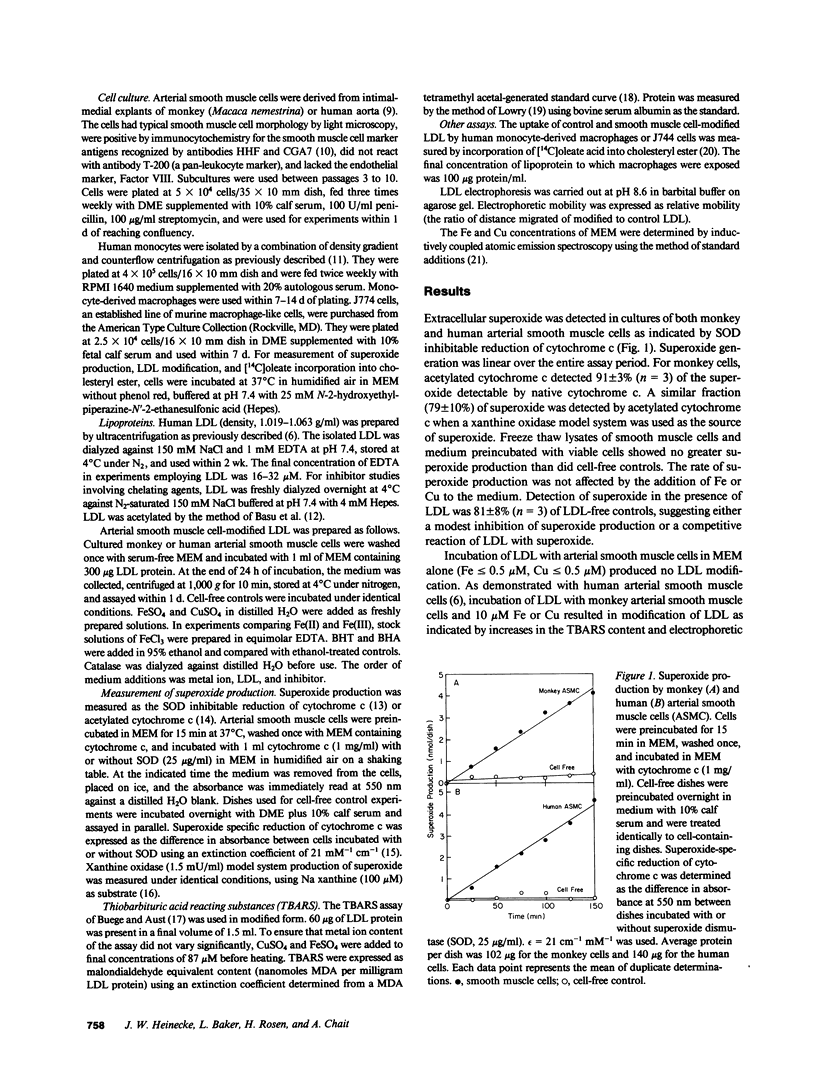
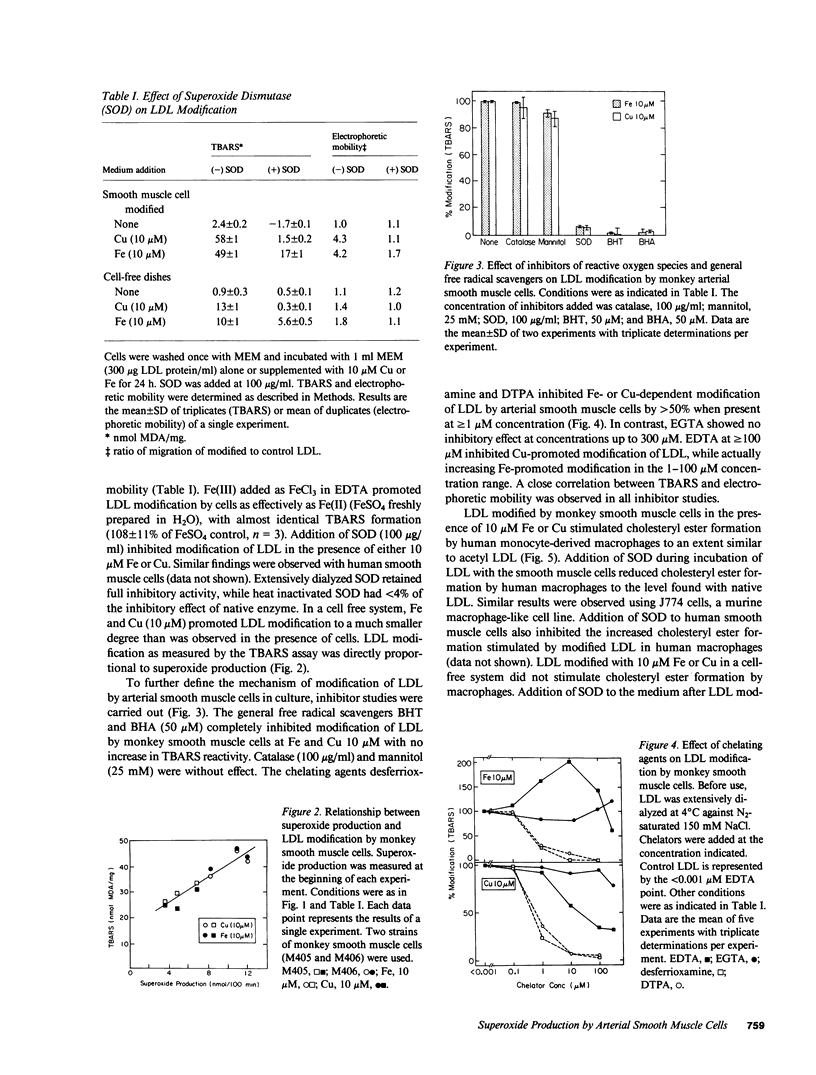
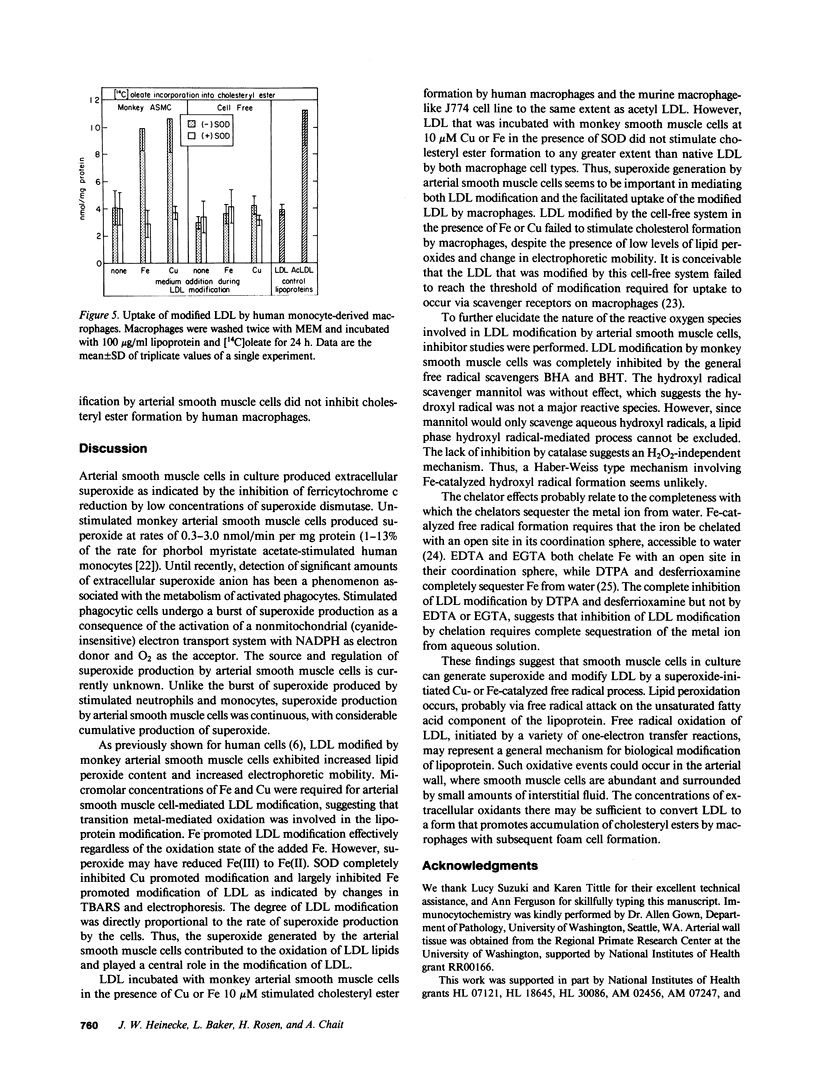
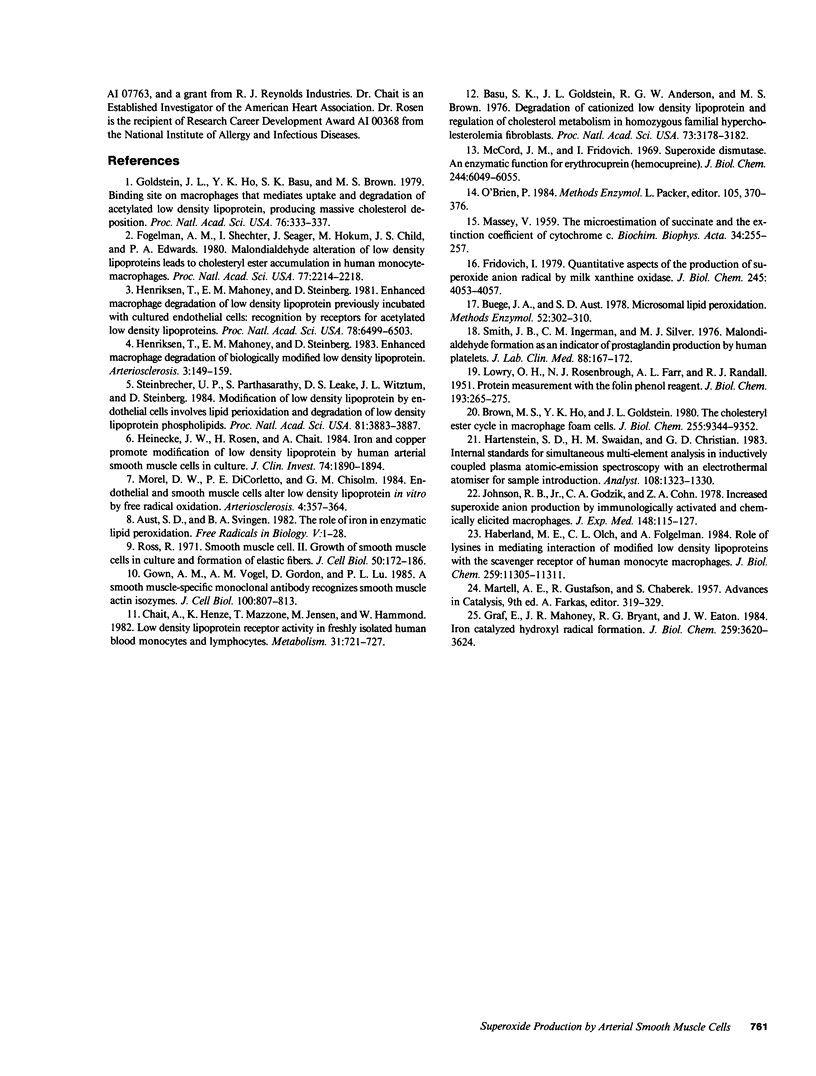
Selected References
These references are in PubMed. This may not be the complete list of references from this article.
- Basu S. K., Goldstein J. L., Anderson G. W., Brown M. S. Degradation of cationized low density lipoprotein and regulation of cholesterol metabolism in homozygous familial hypercholesterolemia fibroblasts. Proc Natl Acad Sci U S A. 1976 Sep;73(9):3178–3182. doi: 10.1073/pnas.73.9.3178. [DOI] [PMC free article] [PubMed] [Google Scholar]
- Brown M. S., Ho Y. K., Goldstein J. L. The cholesteryl ester cycle in macrophage foam cells. Continual hydrolysis and re-esterification of cytoplasmic cholesteryl esters. J Biol Chem. 1980 Oct 10;255(19):9344–9352. [PubMed] [Google Scholar]
- Buege J. A., Aust S. D. Microsomal lipid peroxidation. Methods Enzymol. 1978;52:302–310. doi: 10.1016/s0076-6879(78)52032-6. [DOI] [PubMed] [Google Scholar]
- Chait A., Henze K., Mazzone T., Jensen M., Hammond W. Low density lipoprotein receptor activity in freshly isolated human blood monocytes and lymphocytes. Metabolism. 1982 Jul;31(7):721–727. doi: 10.1016/0026-0495(82)90204-9. [DOI] [PubMed] [Google Scholar]
- Fogelman A. M., Shechter I., Seager J., Hokom M., Child J. S., Edwards P. A. Malondialdehyde alteration of low density lipoproteins leads to cholesteryl ester accumulation in human monocyte-macrophages. Proc Natl Acad Sci U S A. 1980 Apr;77(4):2214–2218. doi: 10.1073/pnas.77.4.2214. [DOI] [PMC free article] [PubMed] [Google Scholar]
- Fridovich I. Quantitative aspects of the production of superoxide anion radical by milk xanthine oxidase. J Biol Chem. 1970 Aug 25;245(16):4053–4057. [PubMed] [Google Scholar]
- Goldstein J. L., Ho Y. K., Basu S. K., Brown M. S. Binding site on macrophages that mediates uptake and degradation of acetylated low density lipoprotein, producing massive cholesterol deposition. Proc Natl Acad Sci U S A. 1979 Jan;76(1):333–337. doi: 10.1073/pnas.76.1.333. [DOI] [PMC free article] [PubMed] [Google Scholar]
- Gown A. M., Vogel A. M., Gordon D., Lu P. L. A smooth muscle-specific monoclonal antibody recognizes smooth muscle actin isozymes. J Cell Biol. 1985 Mar;100(3):807–813. doi: 10.1083/jcb.100.3.807. [DOI] [PMC free article] [PubMed] [Google Scholar]
- Graf E., Mahoney J. R., Bryant R. G., Eaton J. W. Iron-catalyzed hydroxyl radical formation. Stringent requirement for free iron coordination site. J Biol Chem. 1984 Mar 25;259(6):3620–3624. [PubMed] [Google Scholar]
- Haberland M. E., Olch C. L., Folgelman A. M. Role of lysines in mediating interaction of modified low density lipoproteins with the scavenger receptor of human monocyte macrophages. J Biol Chem. 1984 Sep 25;259(18):11305–11311. [PubMed] [Google Scholar]
- Heinecke J. W., Rosen H., Chait A. Iron and copper promote modification of low density lipoprotein by human arterial smooth muscle cells in culture. J Clin Invest. 1984 Nov;74(5):1890–1894. doi: 10.1172/JCI111609. [DOI] [PMC free article] [PubMed] [Google Scholar]
- Henriksen T., Mahoney E. M., Steinberg D. Enhanced macrophage degradation of biologically modified low density lipoprotein. Arteriosclerosis. 1983 Mar-Apr;3(2):149–159. doi: 10.1161/01.atv.3.2.149. [DOI] [PubMed] [Google Scholar]
- Henriksen T., Mahoney E. M., Steinberg D. Enhanced macrophage degradation of low density lipoprotein previously incubated with cultured endothelial cells: recognition by receptors for acetylated low density lipoproteins. Proc Natl Acad Sci U S A. 1981 Oct;78(10):6499–6503. doi: 10.1073/pnas.78.10.6499. [DOI] [PMC free article] [PubMed] [Google Scholar]
- Johnston R. B., Jr, Godzik C. A., Cohn Z. A. Increased superoxide anion production by immunologically activated and chemically elicited macrophages. J Exp Med. 1978 Jul 1;148(1):115–127. doi: 10.1084/jem.148.1.115. [DOI] [PMC free article] [PubMed] [Google Scholar]
- LOWRY O. H., ROSEBROUGH N. J., FARR A. L., RANDALL R. J. Protein measurement with the Folin phenol reagent. J Biol Chem. 1951 Nov;193(1):265–275. [PubMed] [Google Scholar]
- MASSEY V. The microestimation of succinate and the extinction coefficient of cytochrome c. Biochim Biophys Acta. 1959 Jul;34:255–256. doi: 10.1016/0006-3002(59)90259-8. [DOI] [PubMed] [Google Scholar]
- McCord J. M., Fridovich I. Superoxide dismutase. An enzymic function for erythrocuprein (hemocuprein). J Biol Chem. 1969 Nov 25;244(22):6049–6055. [PubMed] [Google Scholar]
- Morel D. W., DiCorleto P. E., Chisolm G. M. Endothelial and smooth muscle cells alter low density lipoprotein in vitro by free radical oxidation. Arteriosclerosis. 1984 Jul-Aug;4(4):357–364. doi: 10.1161/01.atv.4.4.357. [DOI] [PubMed] [Google Scholar]
- O'Brien P. J. Superoxide production. Methods Enzymol. 1984;105:370–378. doi: 10.1016/s0076-6879(84)05050-3. [DOI] [PubMed] [Google Scholar]
- Ross R. The smooth muscle cell. II. Growth of smooth muscle in culture and formation of elastic fibers. J Cell Biol. 1971 Jul;50(1):172–186. doi: 10.1083/jcb.50.1.172. [DOI] [PMC free article] [PubMed] [Google Scholar]
- Smith J. B., Ingerman C. M., Silver M. J. Malondialdehyde formation as an indicator of prostaglandin production by human platelets. J Lab Clin Med. 1976 Jul;88(1):167–172. [PubMed] [Google Scholar]
- Steinbrecher U. P., Parthasarathy S., Leake D. S., Witztum J. L., Steinberg D. Modification of low density lipoprotein by endothelial cells involves lipid peroxidation and degradation of low density lipoprotein phospholipids. Proc Natl Acad Sci U S A. 1984 Jun;81(12):3883–3887. doi: 10.1073/pnas.81.12.3883. [DOI] [PMC free article] [PubMed] [Google Scholar]