Abstract
To investigate whether energy derived from glycolytic and oxidative metabolism are preferentially used for different functions in heart, tension, intracellular potential, and extracellular [K+] and pH (using triple barrel K/pH electrodes, tip diameter 0.5 mm) were monitored in isolated arterially perfused rabbit interventricular septa during exposure to hypoxia and metabolic inhibitors. Myocardial content of high energy phosphates, lactate, and glycogen were determined under the same conditions. Inhibiting oxidative metabolism with hypoxia, dinitrophenol (10(-5)M), or Na-azide (10(-3)M) caused marked suppression of tension (by 73 +/- 5, 65 +/- 8, and 50 +/- 14%, respectively) and a small increase in [K+]0 (0.8 +/- 0.4, 0.5 +/- 0.25, and 0.4 +/- 0.2 mM, respectively) after 10 min. Inhibiting glycolysis with iodoacetate (IAA) (10(-3)M) had a much smaller suppressant effect on tension (28 +/- 24%) but markedly increased [K+]0 accumulation (by 1.8 +/- 1.1 mM) at 10 min. These differences, when IAA was compared individually to the other interventions, were highly significant. The shortening of action potential duration was not significantly different for the four interventions. pH0 increased slightly during IAA (+0.04-+0.06 U) and fell during the other interventions (-0.10--0.16 U), but this did not account for the differences in [K+]0 accumulation and tension between inhibition of glycolytic vs. oxidative metabolism. Except for hypoxia, total cellular content of high energy phosphates was not significantly depressed under the various conditions. This data suggests that energy from glycolysis is preferentially used to support sarcolemmal function (as manifested by K+ loss), whereas oxidatively derived energy preferentially supports contractile function. Indexing terms: cardiac metabolism, extracellular pH, metabolic inhibitors, hypoxia, extracellular K+ accumulation, and glycolysis.
Full text
PDF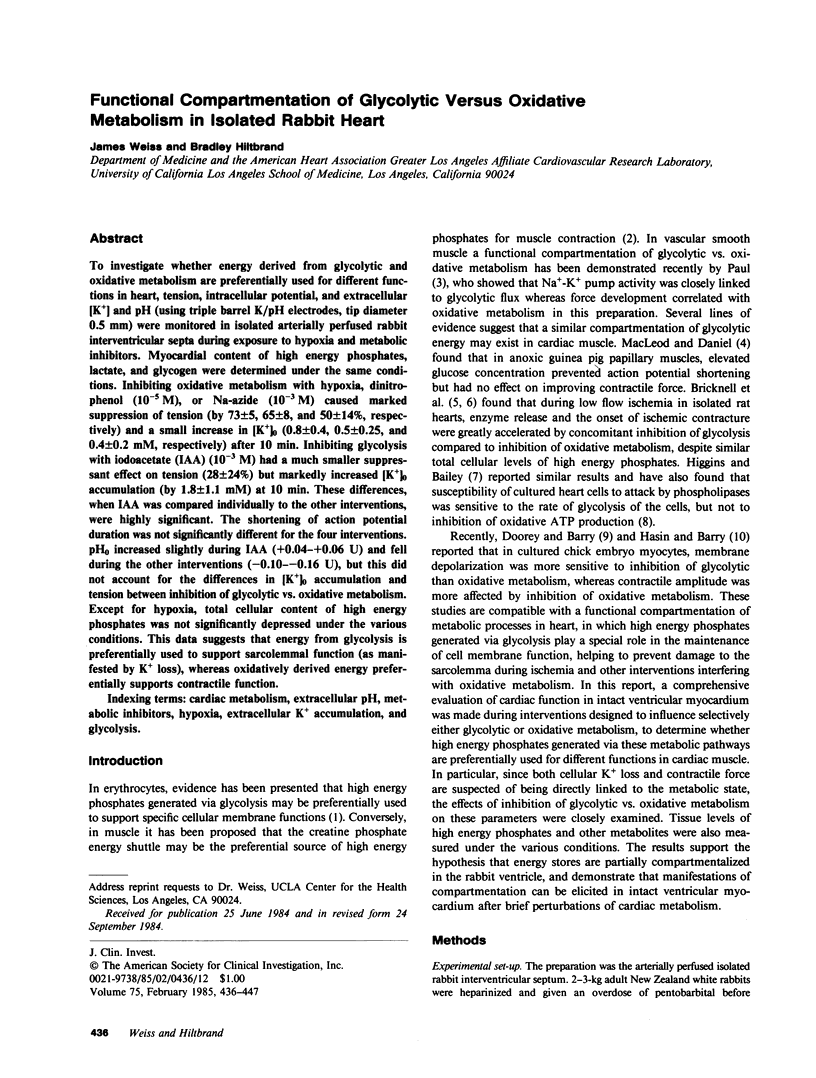
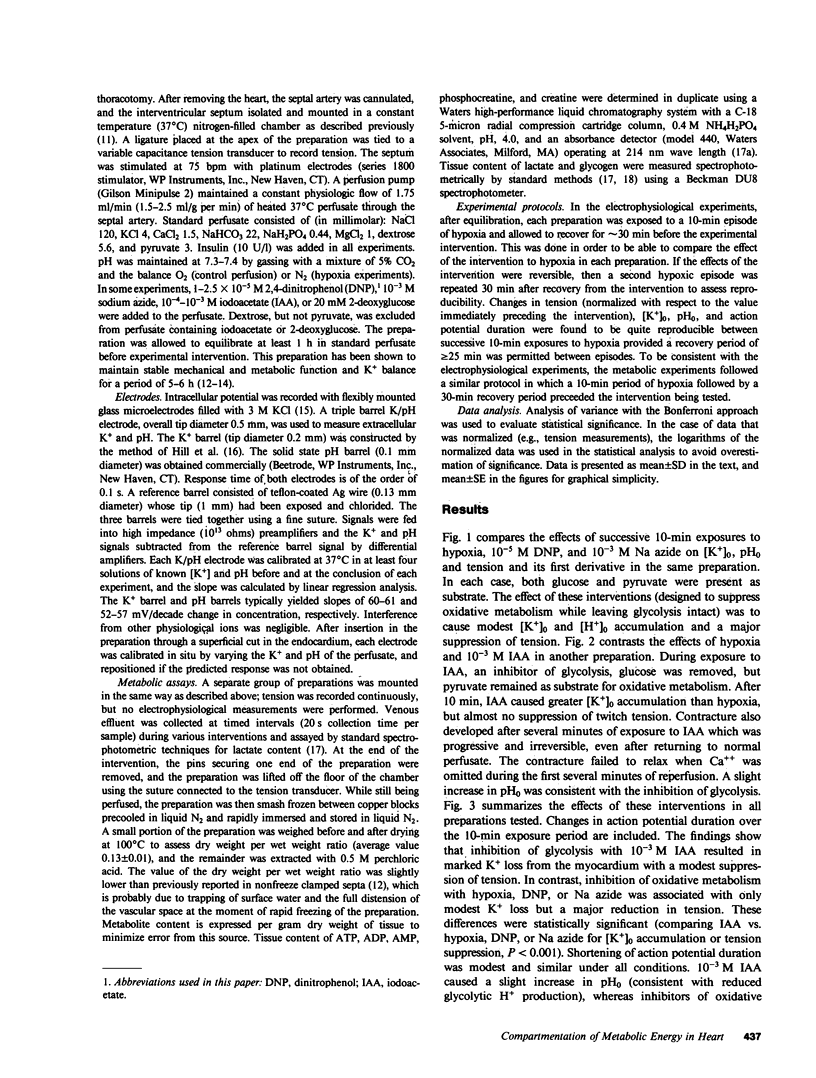
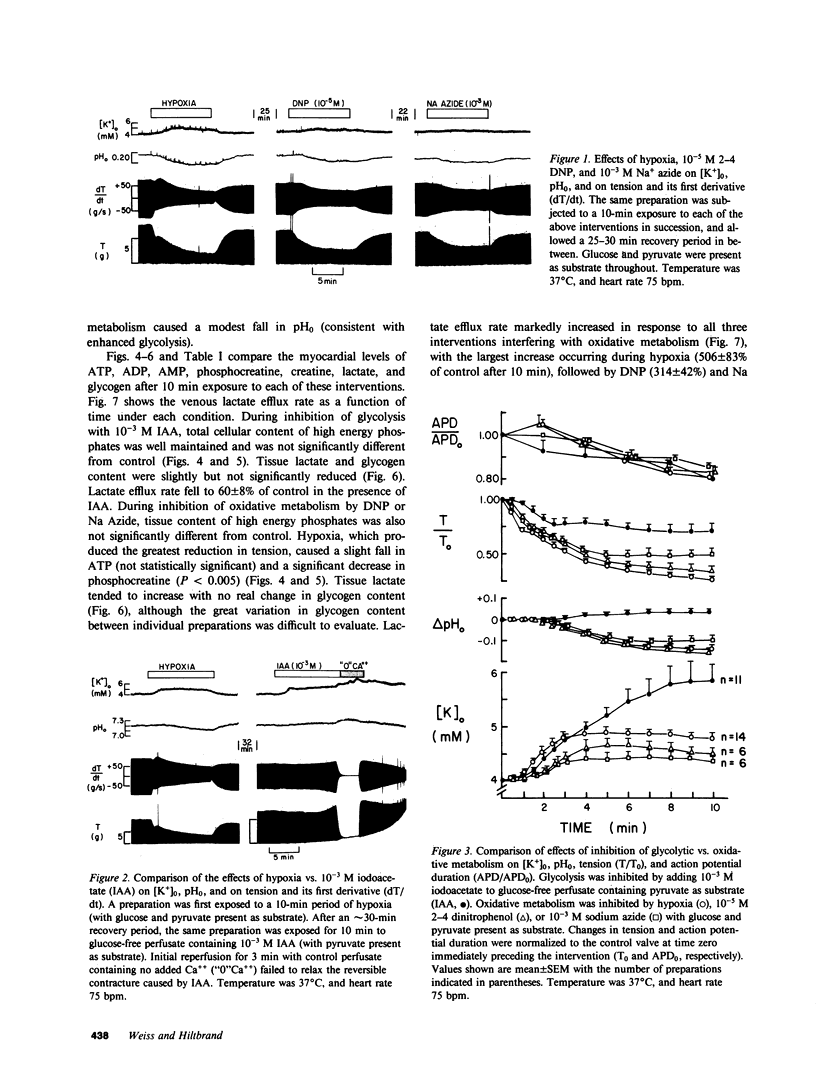
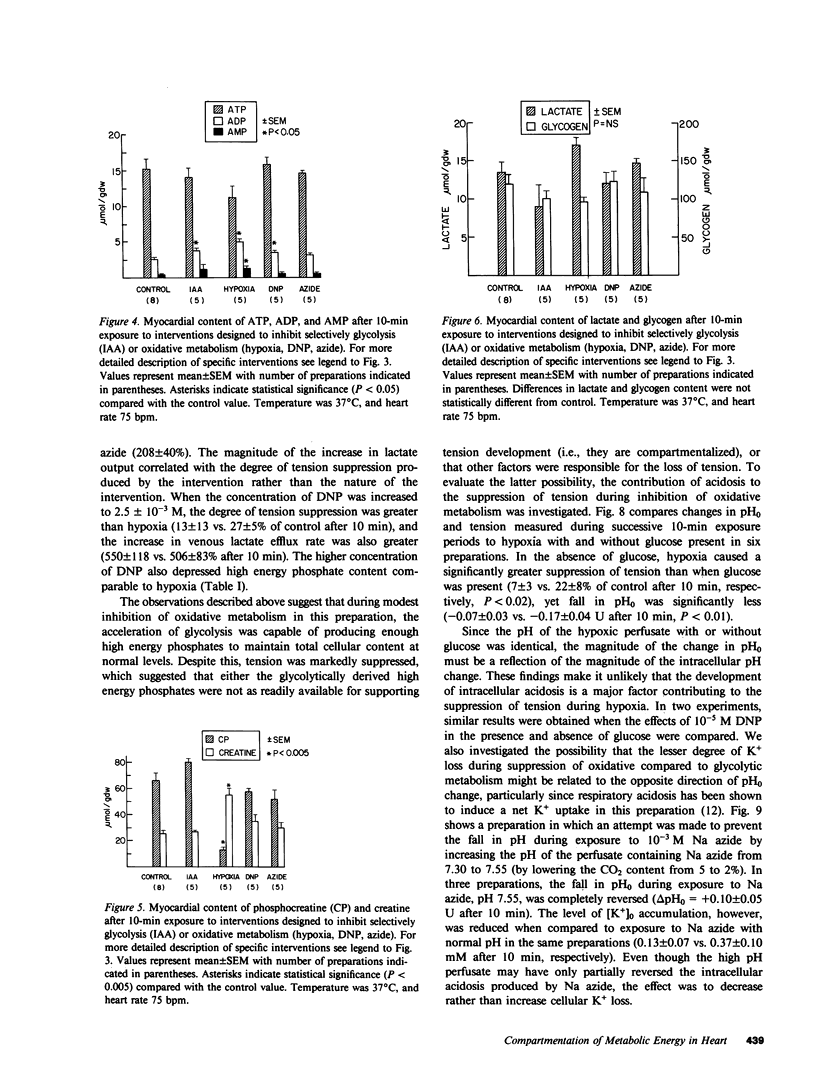
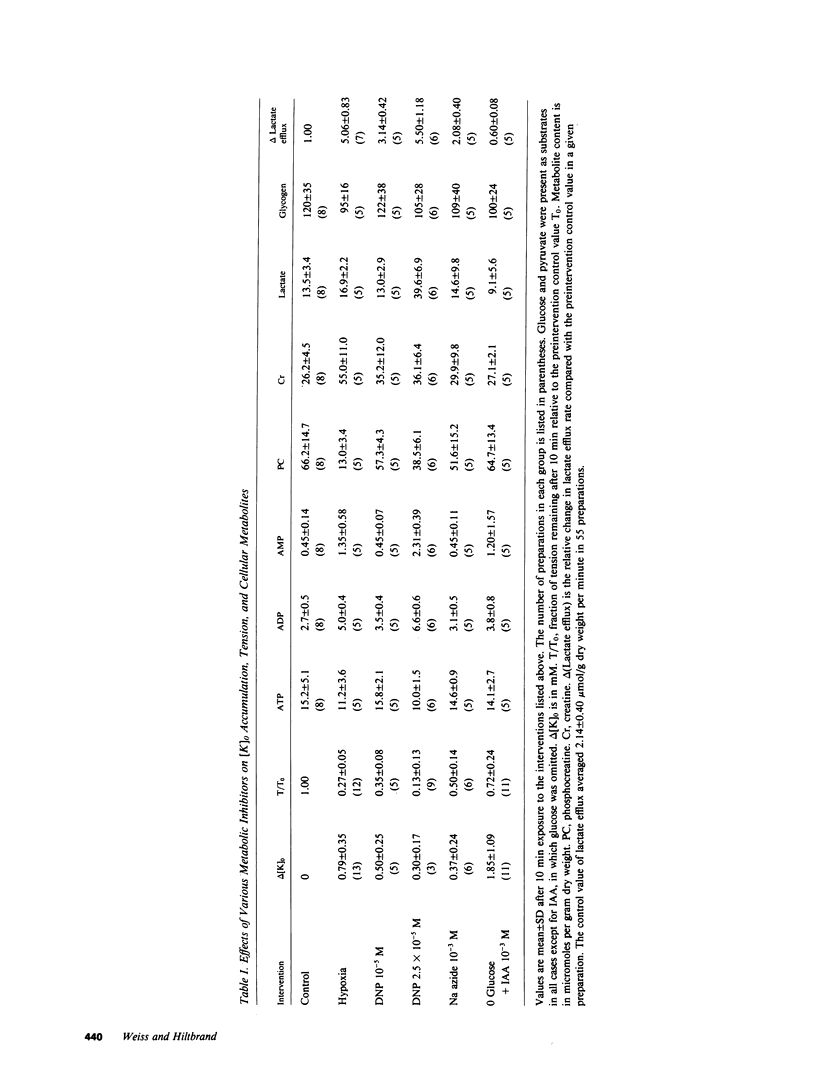
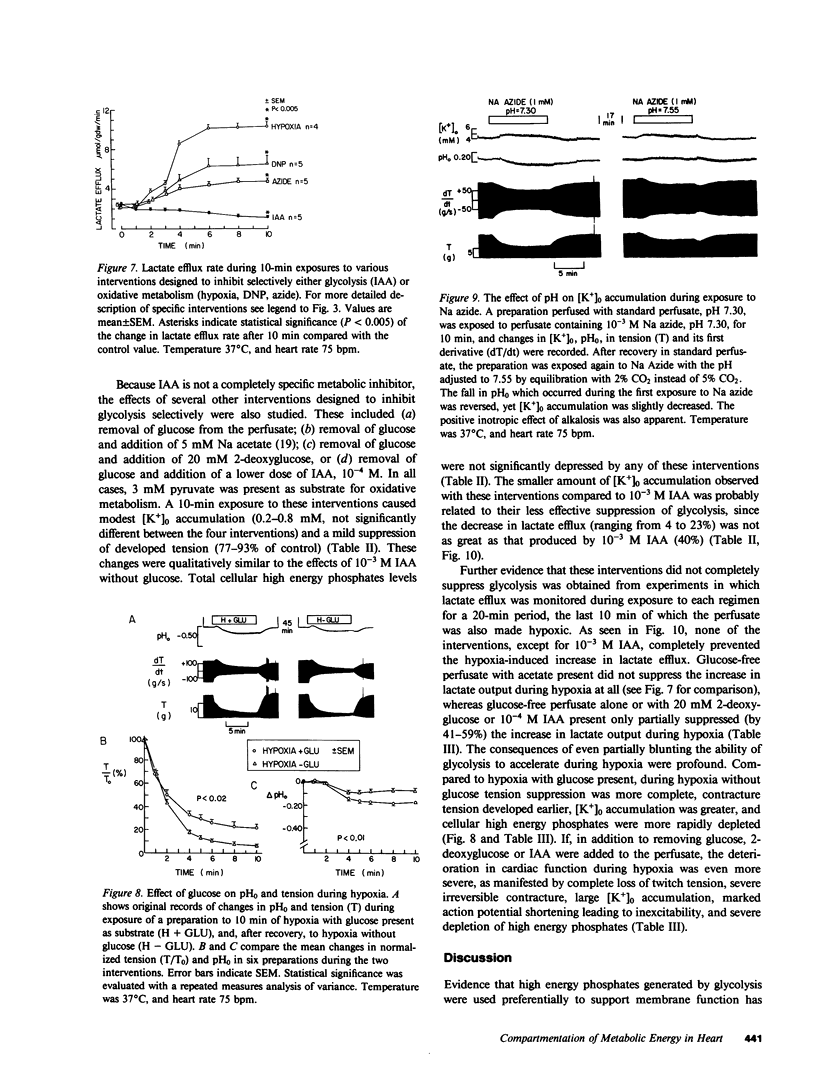
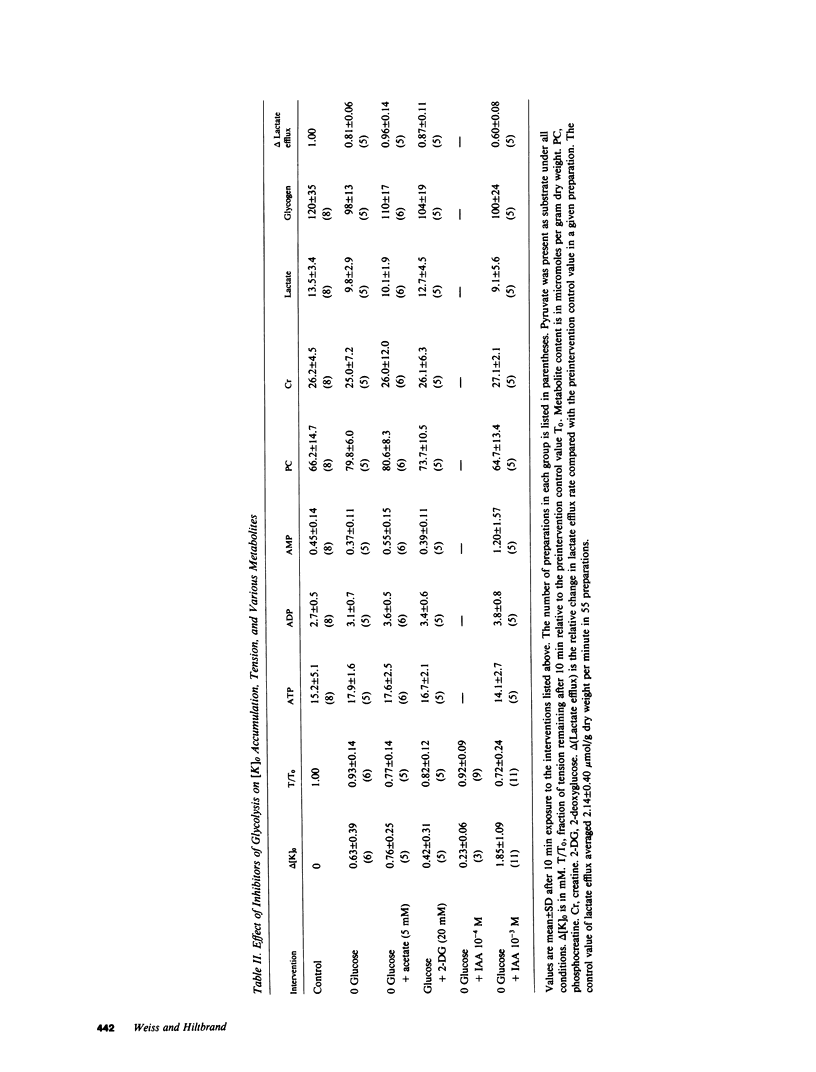
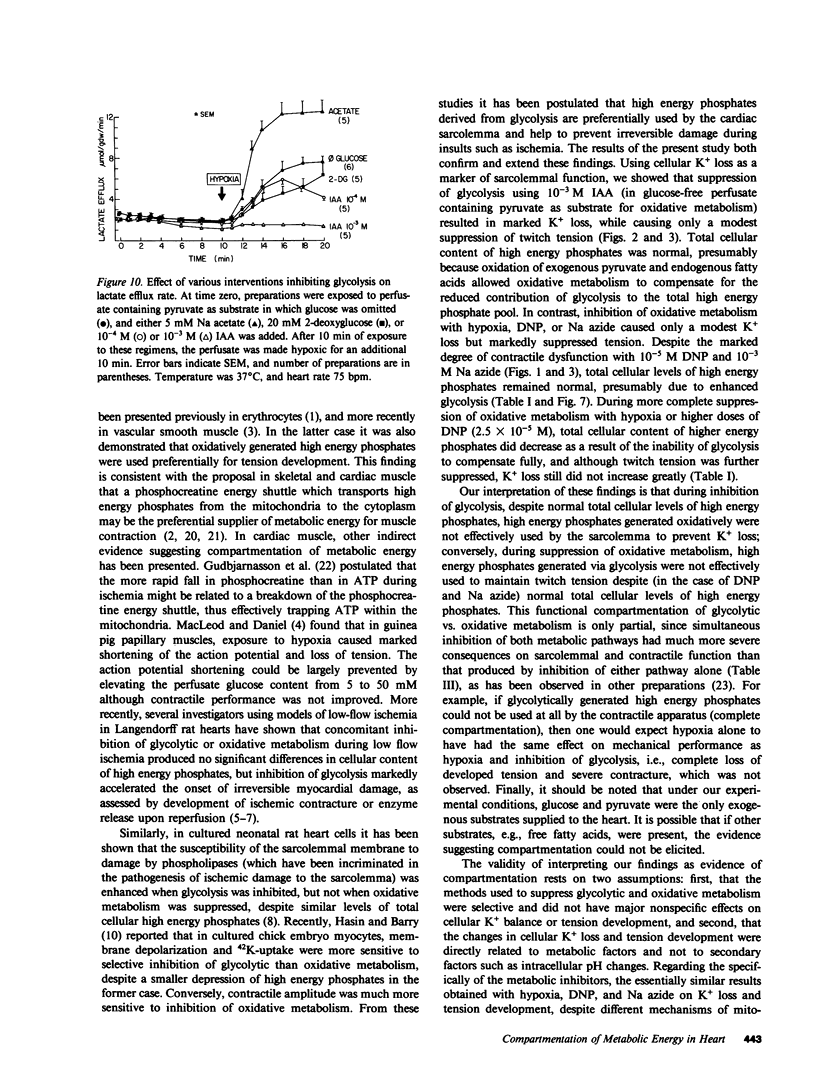
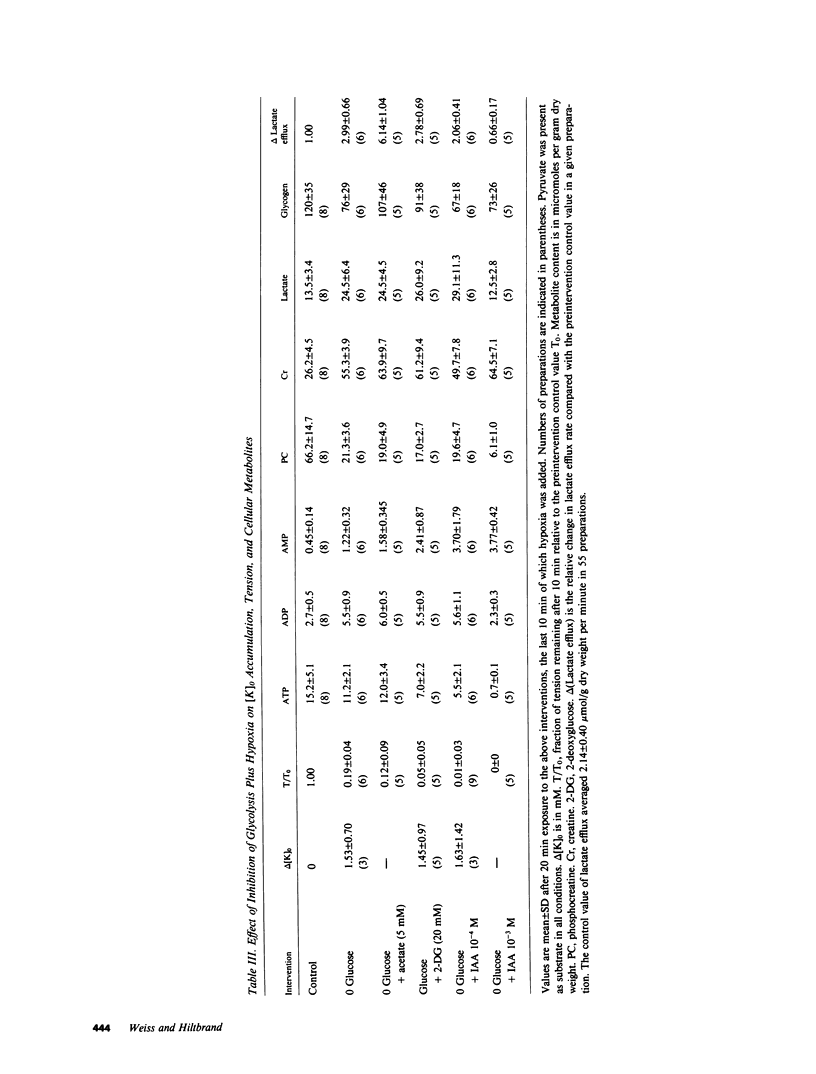
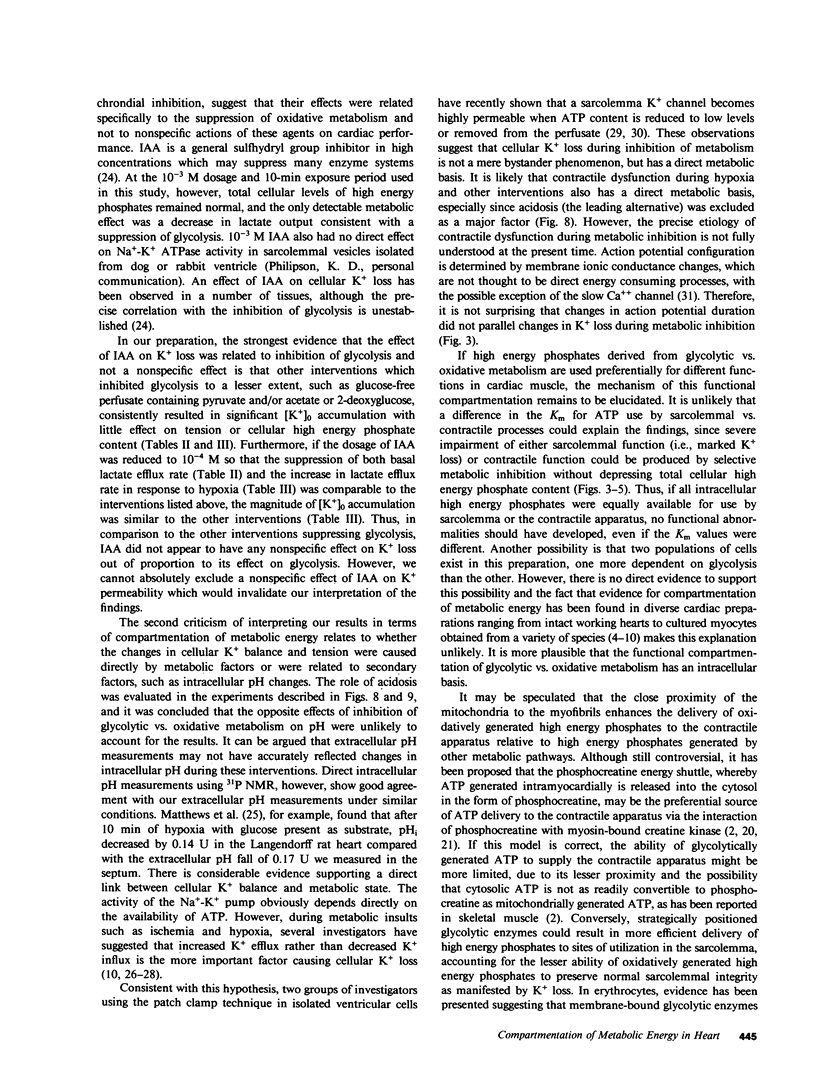
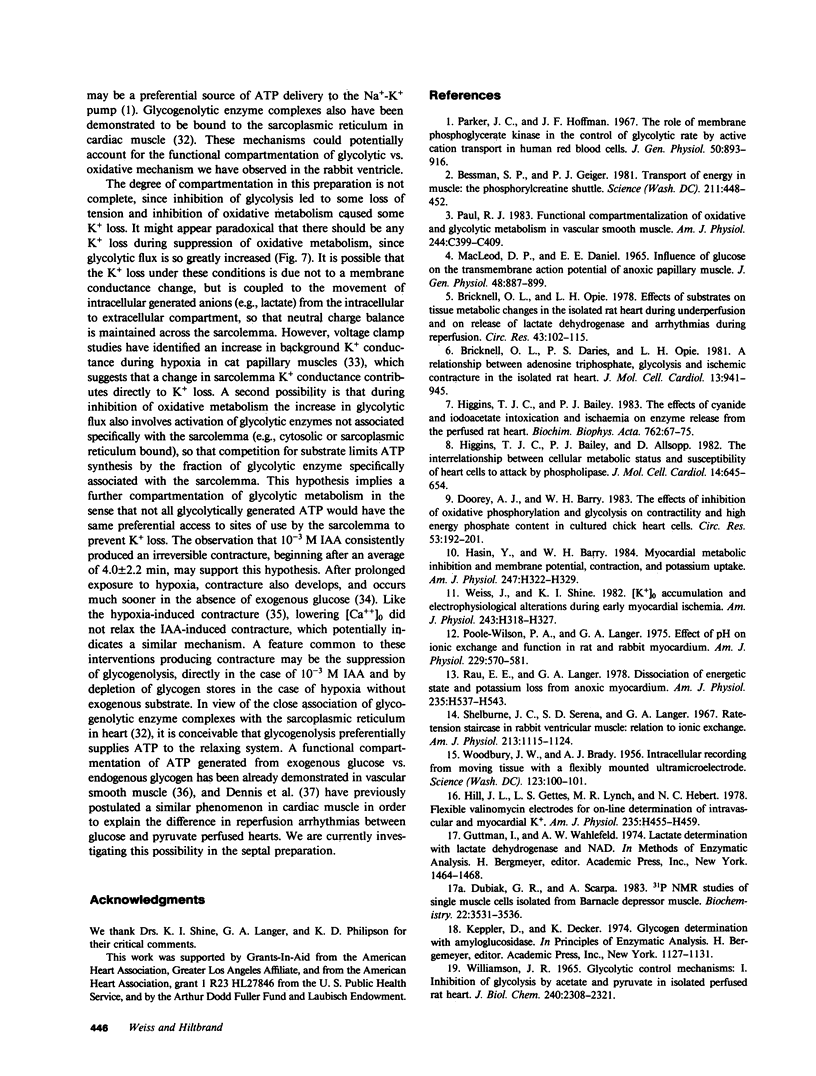
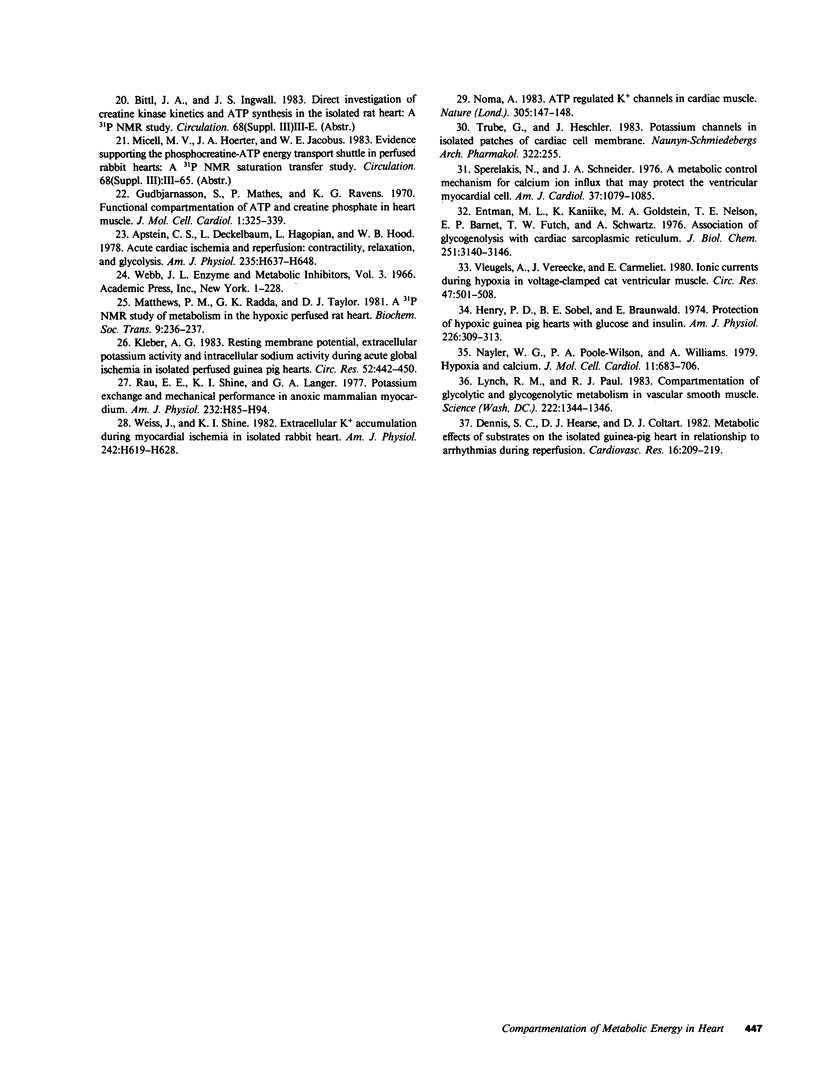
Selected References
These references are in PubMed. This may not be the complete list of references from this article.
- Apstein C. S., Deckelbaum L., Hagopian L., Hood W. B., Jr Acute cardiac ischemia and reperfusion: contractility, relaxation, and glycolysis. Am J Physiol. 1978 Dec;235(6):H637–H648. doi: 10.1152/ajpheart.1978.235.6.H637. [DOI] [PubMed] [Google Scholar]
- Bessman S. P., Geiger P. J. Transport of energy in muscle: the phosphorylcreatine shuttle. Science. 1981 Jan 30;211(4481):448–452. doi: 10.1126/science.6450446. [DOI] [PubMed] [Google Scholar]
- Bricknell O. L., Daries P. S., Opie L. H. A relationship between adenosine triphosphate, glycolysis and ischaemic contracture in the isolated rat heart. J Mol Cell Cardiol. 1981 Oct;13(10):941–945. doi: 10.1016/0022-2828(81)90292-3. [DOI] [PubMed] [Google Scholar]
- Bricknell O. L., Opie L. H. Effects of substrates on tissue metabolic changes in the isolated rat heart during underperfusion and on release of lactate dehydrogenase and arrhythmias during reperfusion. Circ Res. 1978 Jul;43(1):102–115. doi: 10.1161/01.res.43.1.102. [DOI] [PubMed] [Google Scholar]
- Dennis S. C., Hearse D. J., Coltart D. J. Metabolic effects of substrates on the isolated guinea-pig heart in relation to arrhythmias during reperfusion. Cardiovasc Res. 1982 Apr;16(4):209–219. doi: 10.1093/cvr/16.4.209. [DOI] [PubMed] [Google Scholar]
- Doorey A. J., Barry W. H. The effects of inhibition of oxidative phosphorylation and glycolysis on contractility and high-energy phosphate content in cultured chick heart cells. Circ Res. 1983 Aug;53(2):192–201. doi: 10.1161/01.res.53.2.192. [DOI] [PubMed] [Google Scholar]
- Dubyak G. R., Scarpa A. Phosphorus-31 nuclear magnetic resonance studies of single muscle cells isolated from barnacle depressor muscle. Biochemistry. 1983 Jul 5;22(14):3531–3536. doi: 10.1021/bi00283a035. [DOI] [PubMed] [Google Scholar]
- Entam M. L., Kanike K., Goldstein M. A., Nelson T. E., Bornet E. P., Futch T. W., Schwartz A. Association of gylcogenolysis with cardiac sarcoplasmic reticulum. J Biol Chem. 1976 May 25;251(10):3140–3146. [PubMed] [Google Scholar]
- Gudbjarnason S., Mathes P., Ravens K. G. Functional compartmentation of ATP and creatine phosphate in heart muscle. J Mol Cell Cardiol. 1970 Sep;1(3):325–339. doi: 10.1016/0022-2828(70)90009-x. [DOI] [PubMed] [Google Scholar]
- Hasin Y., Barry W. H. Myocardial metabolic inhibition and membrane potential, contraction, and potassium uptake. Am J Physiol. 1984 Aug;247(2 Pt 2):H322–H329. doi: 10.1152/ajpheart.1984.247.2.H322. [DOI] [PubMed] [Google Scholar]
- Henry P. D., Sobel B. E., Braunwald E. Protection of hypoxic guinea pig hearts with glucose and insulin. Am J Physiol. 1974 Feb;226(2):309–313. doi: 10.1152/ajplegacy.1974.226.2.309. [DOI] [PubMed] [Google Scholar]
- Higgins T. J., Bailey P. J., Allsopp D. Interrelationship between cellular metabolic status and susceptibility of heart cells to attack by phospholipase. J Mol Cell Cardiol. 1982 Nov;14(11):645–654. doi: 10.1016/0022-2828(82)90162-6. [DOI] [PubMed] [Google Scholar]
- Higgins T. J., Bailey P. J. The effects of cyanide and iodoacetate intoxication and ischaemia on enzyme release from the perfused rat heart. Biochim Biophys Acta. 1983 Feb 16;762(1):67–75. doi: 10.1016/0167-4889(83)90118-0. [DOI] [PubMed] [Google Scholar]
- Hill J. L., Gettes L. S., Lynch M. R., Hebert N. C. Flexible valinomycin electrodes for on-line determination of intravascular and myocardial K+. Am J Physiol. 1978 Oct;235(4):H455–H459. doi: 10.1152/ajpheart.1978.235.4.H455. [DOI] [PubMed] [Google Scholar]
- Kléber A. G. Resting membrane potential, extracellular potassium activity, and intracellular sodium activity during acute global ischemia in isolated perfused guinea pig hearts. Circ Res. 1983 Apr;52(4):442–450. doi: 10.1161/01.res.52.4.442. [DOI] [PubMed] [Google Scholar]
- Lynch R. M., Paul R. J. Compartmentation of glycolytic and glycogenolytic metabolism in vascular smooth muscle. Science. 1983 Dec 23;222(4630):1344–1346. doi: 10.1126/science.6658455. [DOI] [PubMed] [Google Scholar]
- MACLEOD D. P., DANIEL E. E. INFLUENCE OF GLUCOSE ON THE TRANSMEMBRANE ACTION POTENTIAL OF ANOXIC PAPILLARY MUSCLE. J Gen Physiol. 1965 May;48:887–899. doi: 10.1085/jgp.48.5.887. [DOI] [PMC free article] [PubMed] [Google Scholar]
- Nayler W. G., Poole-Wilson P. A., Williams A. Hypoxia and calcium. J Mol Cell Cardiol. 1979 Jul;11(7):683–706. doi: 10.1016/0022-2828(79)90381-x. [DOI] [PubMed] [Google Scholar]
- Noma A. ATP-regulated K+ channels in cardiac muscle. Nature. 1983 Sep 8;305(5930):147–148. doi: 10.1038/305147a0. [DOI] [PubMed] [Google Scholar]
- Parker J. C., Hoffman J. F. The role of membrane phosphoglycerate kinase in the control of glycolytic rate by active cation transport in human red blood cells. J Gen Physiol. 1967 Mar;50(4):893–916. doi: 10.1085/jgp.50.4.893. [DOI] [PMC free article] [PubMed] [Google Scholar]
- Paul R. J. Functional compartmentalization of oxidative and glycolytic metabolism in vascular smooth muscle. Am J Physiol. 1983 May;244(5):C399–C409. doi: 10.1152/ajpcell.1983.244.5.C399. [DOI] [PubMed] [Google Scholar]
- Poole-Wilson P. A., Langer G. A. Effect of pH on ionic exchange and function in rat and rabbit myocardium. Am J Physiol. 1975 Sep;229(3):570–581. doi: 10.1152/ajplegacy.1975.229.3.570. [DOI] [PubMed] [Google Scholar]
- Rau E. E., Langer G. A. Dissociation of energetic state and potassium loss from anoxic myocardium. Am J Physiol. 1978 Nov;235(5):H537–H543. doi: 10.1152/ajpheart.1978.235.5.H537. [DOI] [PubMed] [Google Scholar]
- Rau E. E., Shine K. I., Langer G. A. Potassium exchange and mechanical performance in anoxic mammalian myocardium. Am J Physiol. 1977 Jan;232(1):H85–H94. doi: 10.1152/ajpheart.1977.232.1.H85. [DOI] [PubMed] [Google Scholar]
- Shelburne J. C., Serena S. D., Langer G. A. Rate-tension staircase in rabbit ventricular muscle: relation to ionic exchange. Am J Physiol. 1967 Nov;213(5):1115–1124. doi: 10.1152/ajplegacy.1967.213.5.1115. [DOI] [PubMed] [Google Scholar]
- Sperelakis N., Schneider J. A. A metabolic control mechanism for calcium ion influx that may protect the ventricular myocardial cell. Am J Cardiol. 1976 Jun;37(7):1079–1085. doi: 10.1016/0002-9149(76)90428-8. [DOI] [PubMed] [Google Scholar]
- Vleugels A., Vereecke J., Carmeliet E. Ionic currents during hypoxia in voltage-clamped cat ventricular muscle. Circ Res. 1980 Oct;47(4):501–508. doi: 10.1161/01.res.47.4.501. [DOI] [PubMed] [Google Scholar]
- WILLIAMSON J. R. GLYCOLYTIC CONTROL MECHANISMS. I. INHIBITION OF GLYCOLYSIS BY ACETATE AND PYRUVATE IN THE ISOLATED, PERFUSED RAT HEART. J Biol Chem. 1965 Jun;240:2308–2321. [PubMed] [Google Scholar]
- WOODBURY J. W., BRADY A. J. Intracellular recording from moving tissues with a flexibly mounted ultramicroelectrode. Science. 1956 Jan 20;123(3186):100–101. doi: 10.1126/science.123.3186.100-a. [DOI] [PubMed] [Google Scholar]
- Weiss J., Shine K. I. Extracellular K+ accumulation during myocardial ischemia in isolated rabbit heart. Am J Physiol. 1982 Apr;242(4):H619–H628. doi: 10.1152/ajpheart.1982.242.4.H619. [DOI] [PubMed] [Google Scholar]
- Weiss J., Shine K. I. [K+]o accumulation and electrophysiological alterations during early myocardial ischemia. Am J Physiol. 1982 Aug;243(2):H318–H327. doi: 10.1152/ajpheart.1982.243.2.H318. [DOI] [PubMed] [Google Scholar]