Abstract
To examine the relative roles of apical and basolateral membrane transport mechanisms in the regulation of cell pH in the proximal convoluted tubule, cell pH was measured in the in vivo microperfused rat tubule using fluorescence. Decreasing luminal pH by 0.7 pH units caused cell pH to decrease by 0.08 pH units, whereas a similar decrease in peritubular pH caused cell pH to decrease by 0.32 pH units. Inhibition of basolateral membrane bicarbonate transport with peritubular 4-acetamido-4'-isothiocyanostilbene-2,2'-disulfonate (SITS) enhanced the response to luminal fluid acidification. Removal of luminal sodium caused a small transient acidification which was followed by a late alkalinization. Peritubular SITS increased the magnitude of the transient acidification, and eliminated the late alkalinization. The acidification was partially inhibited by luminal amiloride. The results demonstrate sodium-coupled processes on both the apical (Na/H antiport) and basolateral (Na/HCO3 symport) membranes. Basolateral membrane transporters are more important determinants of cell pH.
Full text
PDF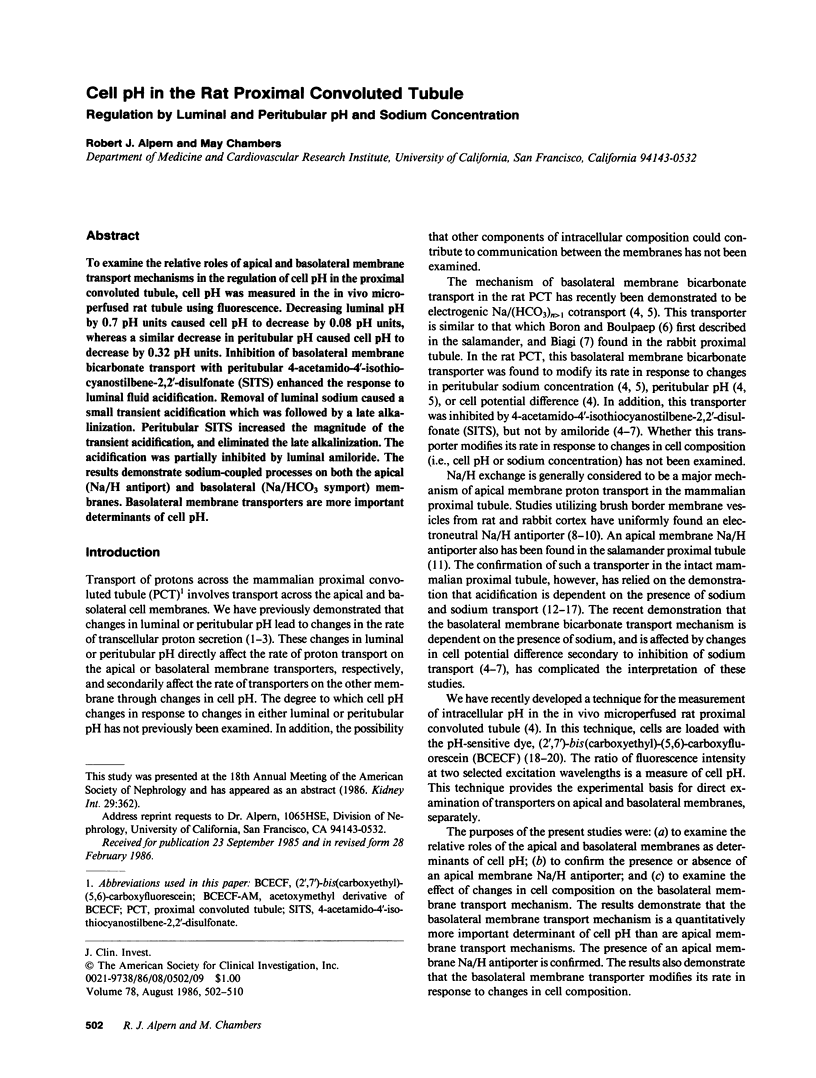
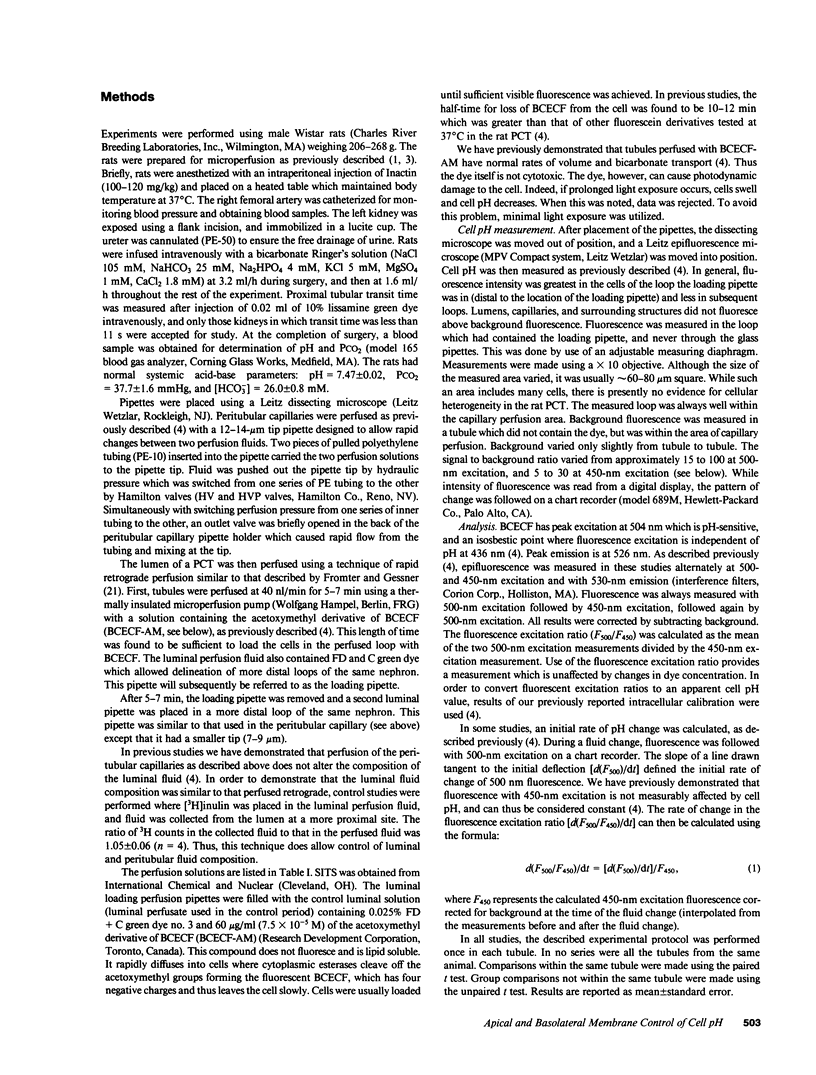
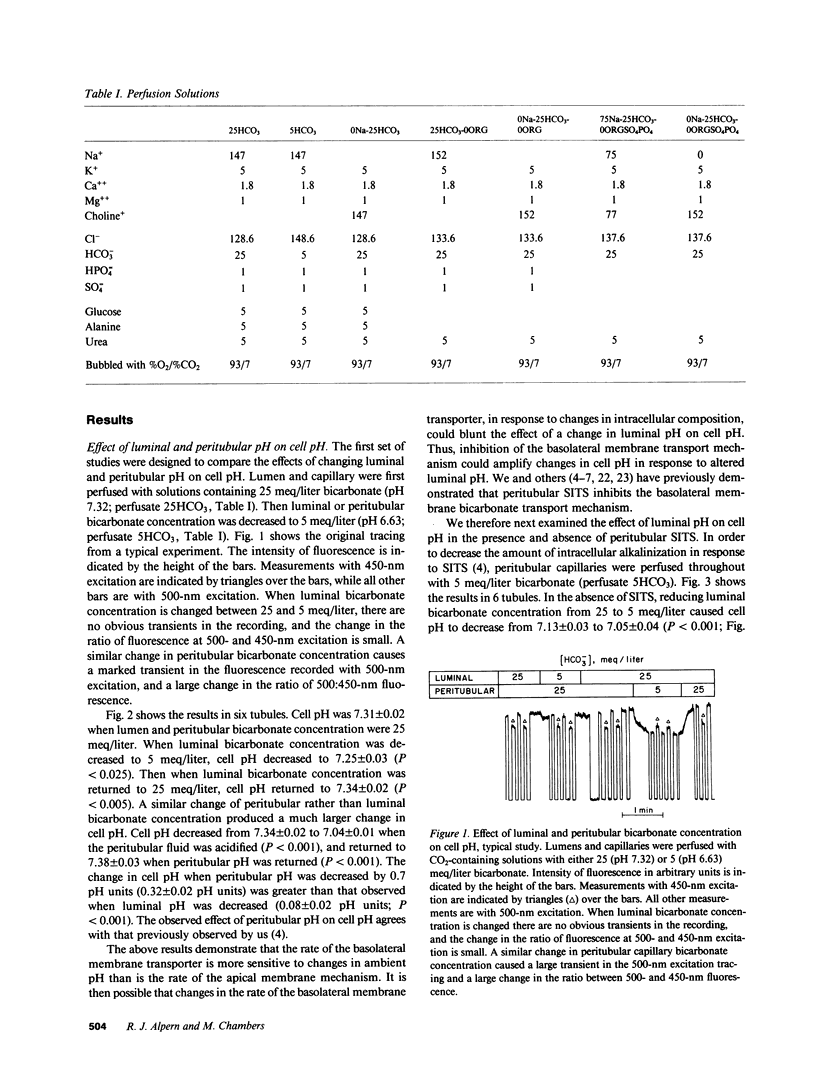
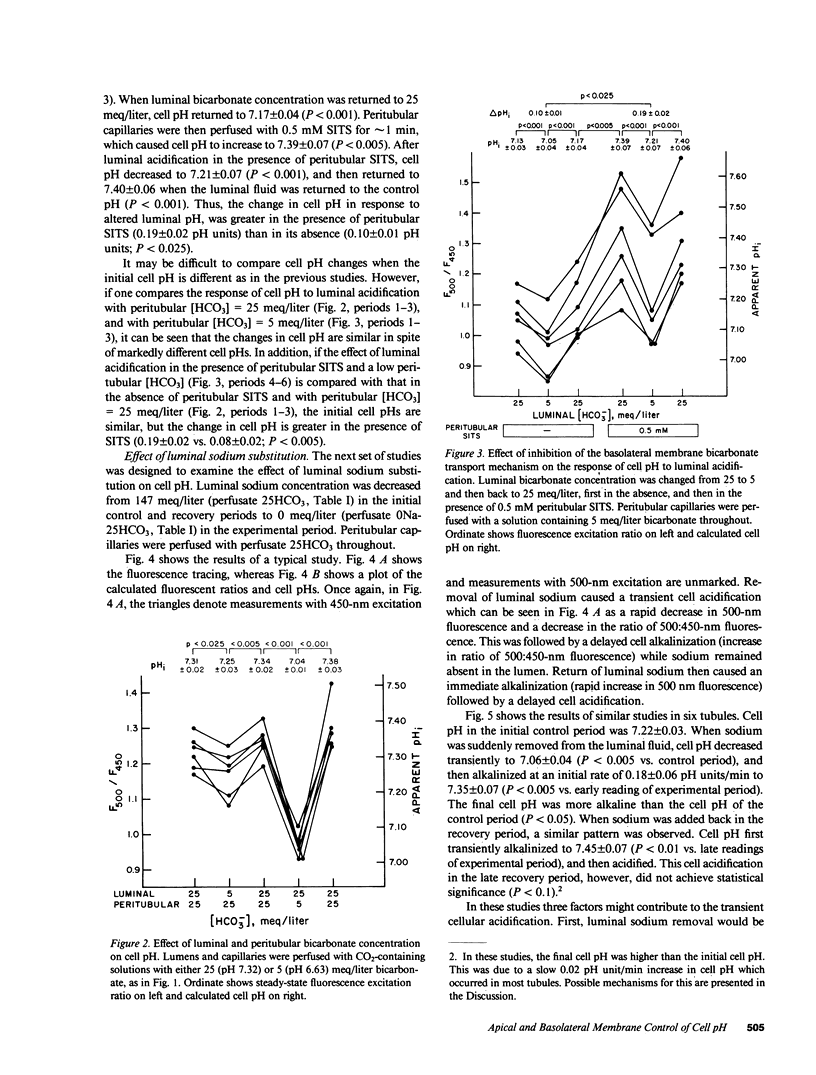
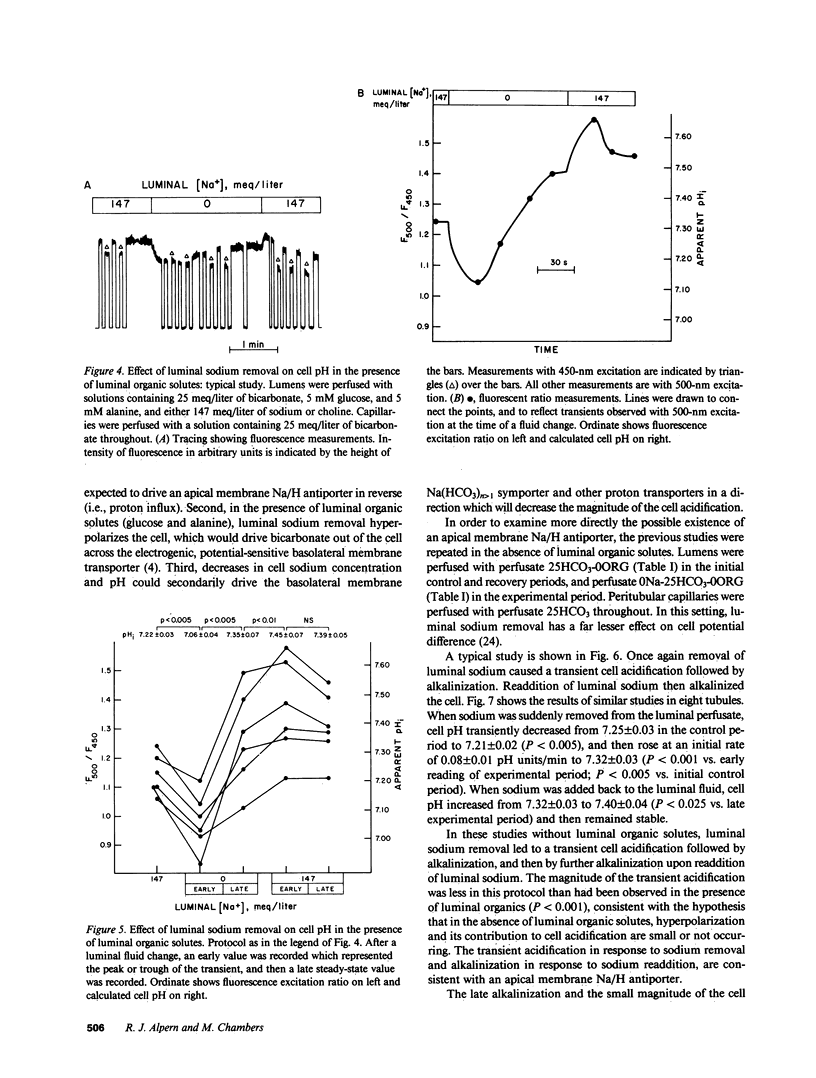
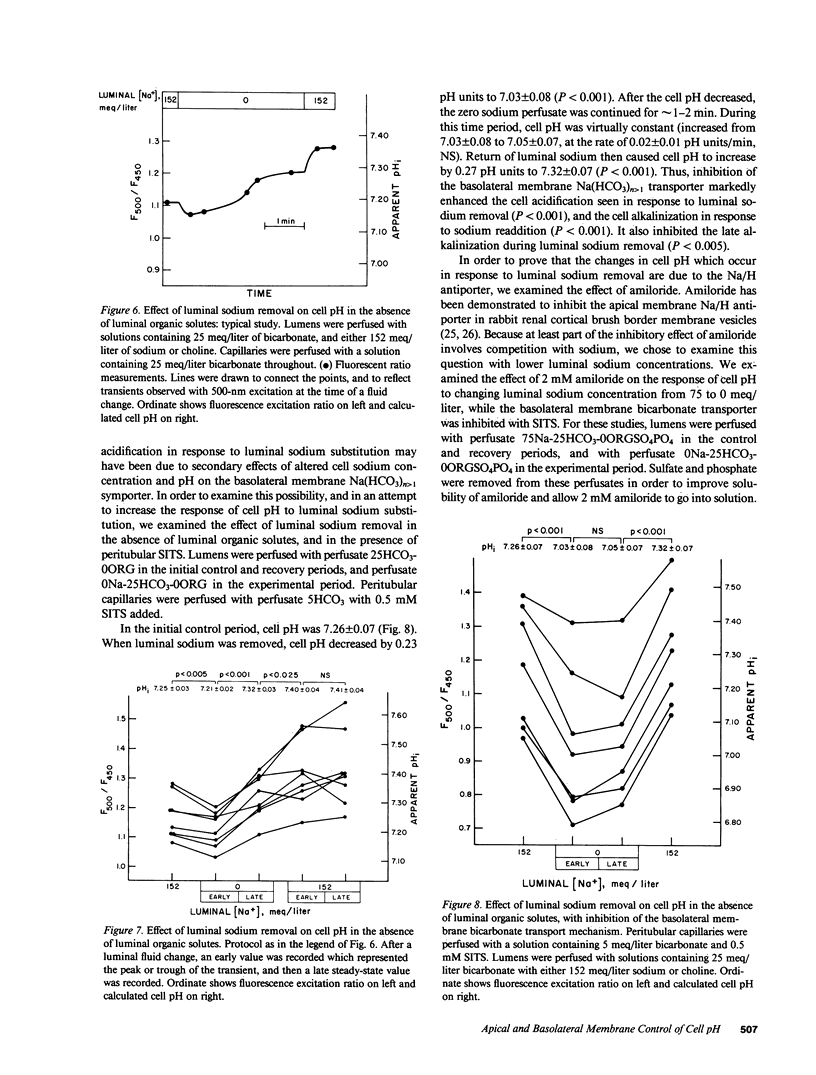
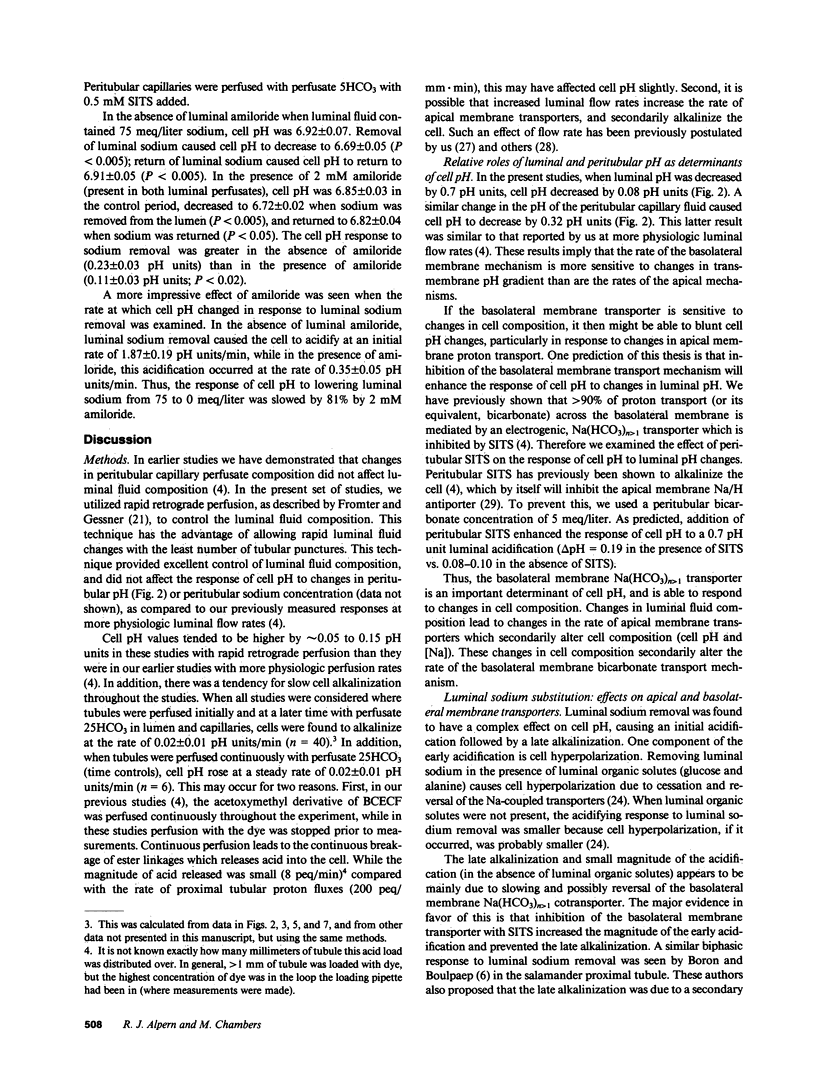

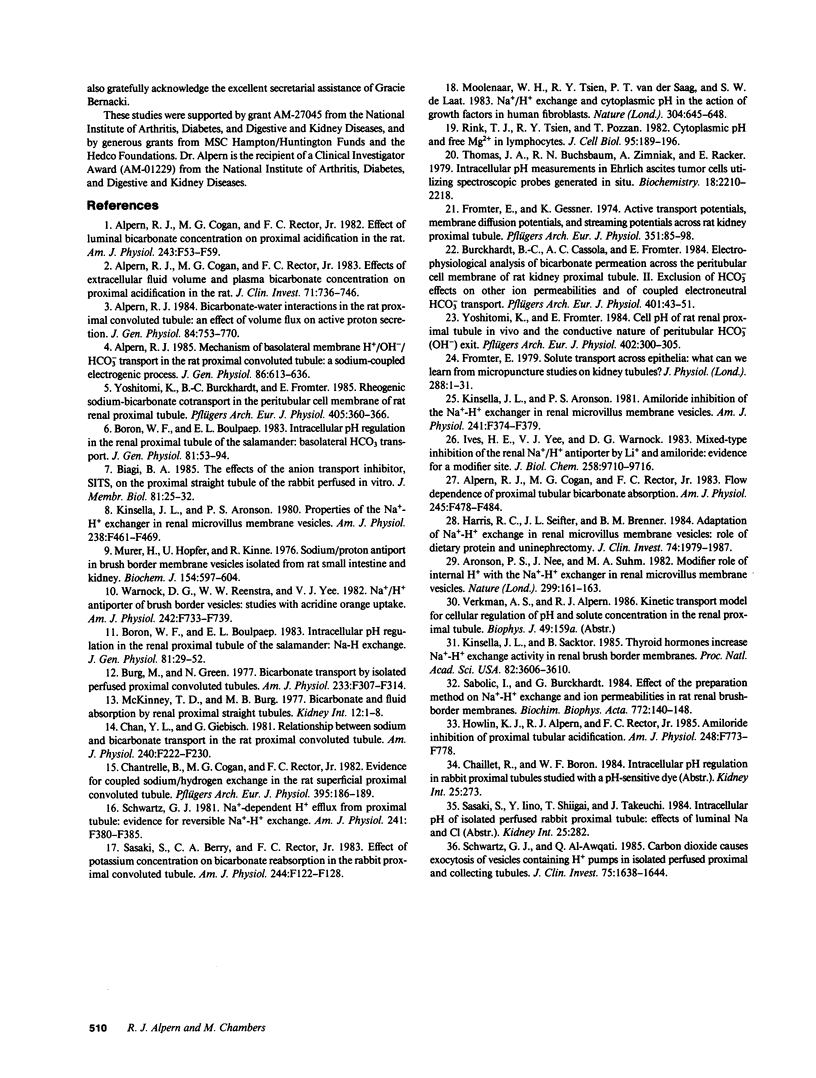
Selected References
These references are in PubMed. This may not be the complete list of references from this article.
- Alpern R. J. Bicarbonate-water interactions in the rat proximal convoluted tubule. An effect of volume flux on active proton secretion. J Gen Physiol. 1984 Nov;84(5):753–770. doi: 10.1085/jgp.84.5.753. [DOI] [PMC free article] [PubMed] [Google Scholar]
- Alpern R. J., Cogan M. G., Rector F. C., Jr Effect of luminal bicarbonate concentration on proximal acidification in the rat. Am J Physiol. 1982 Jul;243(1):F53–F59. doi: 10.1152/ajprenal.1982.243.1.F53. [DOI] [PubMed] [Google Scholar]
- Alpern R. J., Cogan M. G., Rector F. C., Jr Effects of extracellular fluid volume and plasma bicarbonate concentration on proximal acidification in the rat. J Clin Invest. 1983 Mar;71(3):736–746. doi: 10.1172/JCI110821. [DOI] [PMC free article] [PubMed] [Google Scholar]
- Alpern R. J., Cogan M. G., Rector F. C., Jr Flow dependence of proximal tubular bicarbonate absorption. Am J Physiol. 1983 Oct;245(4):F478–F484. doi: 10.1152/ajprenal.1983.245.4.F478. [DOI] [PubMed] [Google Scholar]
- Alpern R. J. Mechanism of basolateral membrane H+/OH-/HCO-3 transport in the rat proximal convoluted tubule. A sodium-coupled electrogenic process. J Gen Physiol. 1985 Nov;86(5):613–636. doi: 10.1085/jgp.86.5.613. [DOI] [PMC free article] [PubMed] [Google Scholar]
- Aronson P. S., Nee J., Suhm M. A. Modifier role of internal H+ in activating the Na+-H+ exchanger in renal microvillus membrane vesicles. Nature. 1982 Sep 9;299(5879):161–163. doi: 10.1038/299161a0. [DOI] [PubMed] [Google Scholar]
- Biagi B. A. Effects of the anion transport inhibitor, SITS, on the proximal straight tubule of the rabbit perfused in vitro. J Membr Biol. 1985;88(1):25–31. doi: 10.1007/BF01871210. [DOI] [PubMed] [Google Scholar]
- Boron W. F., Boulpaep E. L. Intracellular pH regulation in the renal proximal tubule of the salamander. Basolateral HCO3- transport. J Gen Physiol. 1983 Jan;81(1):53–94. doi: 10.1085/jgp.81.1.53. [DOI] [PMC free article] [PubMed] [Google Scholar]
- Boron W. F., Boulpaep E. L. Intracellular pH regulation in the renal proximal tubule of the salamander. Na-H exchange. J Gen Physiol. 1983 Jan;81(1):29–52. doi: 10.1085/jgp.81.1.29. [DOI] [PMC free article] [PubMed] [Google Scholar]
- Burckhardt B. C., Cassola A. C., Frömter E. Electrophysiological analysis of bicarbonate permeation across the peritubular cell membrane of rat kidney proximal tubule. II. Exclusion of HCO3(-)-effects on other ion permeabilities and of coupled electroneutral HCO3(-)-transport. Pflugers Arch. 1984 May;401(1):43–51. doi: 10.1007/BF00581531. [DOI] [PubMed] [Google Scholar]
- Burg M., Green N. Bicarbonate transport by isolated perfused rabbit proximal convoluted tubules. Am J Physiol. 1977 Oct;233(4):F307–F314. doi: 10.1152/ajprenal.1977.233.4.F307. [DOI] [PubMed] [Google Scholar]
- Chan Y. L., Giebisch G. Relationship between sodium and bicarbonate transport in the rat proximal convoluted tubule. Am J Physiol. 1981 Mar;240(3):F222–F230. doi: 10.1152/ajprenal.1981.240.3.F222. [DOI] [PubMed] [Google Scholar]
- Chantrelle B., Cogan M. G., Rector F. C., Jr Evidence for coupled sodium/hydrogen exchange in the rat superficial proximal convoluted tubule. Pflugers Arch. 1982 Nov 11;395(3):186–189. doi: 10.1007/BF00584807. [DOI] [PubMed] [Google Scholar]
- Frömter E., Gessner K. Active transport potentials, membrane diffusion potentials and streaming potentials across rat kidney proximal tubule. Pflugers Arch. 1974;351(1):85–98. doi: 10.1007/BF00603513. [DOI] [PubMed] [Google Scholar]
- Frömter E. The Feldberg Lecture 1976. Solute transport across epithelia: what can we learn from micropuncture studies in kidney tubules? J Physiol. 1979 Mar;288:1–31. [PMC free article] [PubMed] [Google Scholar]
- Harris R. C., Seifter J. L., Brenner B. M. Adaptation of Na+-H+ exchange in renal microvillus membrane vesicles. Role of dietary protein and uninephrectomy. J Clin Invest. 1984 Dec;74(6):1979–1987. doi: 10.1172/JCI111619. [DOI] [PMC free article] [PubMed] [Google Scholar]
- Howlin K. J., Alpern R. J., Rector F. C., Jr Amiloride inhibition of proximal tubular acidification. Am J Physiol. 1985 Jun;248(6 Pt 2):F773–F778. doi: 10.1152/ajprenal.1985.248.6.F773. [DOI] [PubMed] [Google Scholar]
- Ives H. E., Yee V. J., Warnock D. G. Mixed type inhibition of the renal Na+/H+ antiporter by Li+ and amiloride. Evidence for a modifier site. J Biol Chem. 1983 Aug 25;258(16):9710–9716. [PubMed] [Google Scholar]
- Kinsella J. L., Aronson P. S. Amiloride inhibition of the Na+-H+ exchanger in renal microvillus membrane vesicles. Am J Physiol. 1981 Oct;241(4):F374–F379. doi: 10.1152/ajprenal.1981.241.4.F374. [DOI] [PubMed] [Google Scholar]
- Kinsella J. L., Aronson P. S. Properties of the Na+-H+ exchanger in renal microvillus membrane vesicles. Am J Physiol. 1980 Jun;238(6):F461–F469. doi: 10.1152/ajprenal.1980.238.6.F461. [DOI] [PubMed] [Google Scholar]
- Kinsella J., Sacktor B. Thyroid hormones increase Na+-H+ exchange activity in renal brush border membranes. Proc Natl Acad Sci U S A. 1985 Jun;82(11):3606–3610. doi: 10.1073/pnas.82.11.3606. [DOI] [PMC free article] [PubMed] [Google Scholar]
- McKinney T. D., Burg M. B. Biocarbonate and fluid absorption by renal proximal straight tubules. Kidney Int. 1977 Jul;12(1):1–8. doi: 10.1038/ki.1977.72. [DOI] [PubMed] [Google Scholar]
- Moolenaar W. H., Tsien R. Y., van der Saag P. T., de Laat S. W. Na+/H+ exchange and cytoplasmic pH in the action of growth factors in human fibroblasts. Nature. 1983 Aug 18;304(5927):645–648. doi: 10.1038/304645a0. [DOI] [PubMed] [Google Scholar]
- Murer H., Hopfer U., Kinne R. Sodium/proton antiport in brush-border-membrane vesicles isolated from rat small intestine and kidney. Biochem J. 1976 Mar 15;154(3):597–604. [PMC free article] [PubMed] [Google Scholar]
- Rink T. J., Tsien R. Y., Pozzan T. Cytoplasmic pH and free Mg2+ in lymphocytes. J Cell Biol. 1982 Oct;95(1):189–196. doi: 10.1083/jcb.95.1.189. [DOI] [PMC free article] [PubMed] [Google Scholar]
- Sabolić I., Burckhardt G. Effect of the preparation method on Na+-H+ exchange and ion permeabilities in rat renal brush-border membranes. Biochim Biophys Acta. 1984 May 16;772(2):140–148. doi: 10.1016/0005-2736(84)90037-3. [DOI] [PubMed] [Google Scholar]
- Sasaki S., Berry C. A., Rector F. C., Jr Effect of potassium concentration on bicarbonate reabsorption in the rabbit proximal convoluted tubule. Am J Physiol. 1983 Feb;244(2):F122–F128. doi: 10.1152/ajprenal.1983.244.2.F122. [DOI] [PubMed] [Google Scholar]
- Schwartz G. J., Al-Awqati Q. Carbon dioxide causes exocytosis of vesicles containing H+ pumps in isolated perfused proximal and collecting tubules. J Clin Invest. 1985 May;75(5):1638–1644. doi: 10.1172/JCI111871. [DOI] [PMC free article] [PubMed] [Google Scholar]
- Schwartz G. J. Na+-dependent H+ efflux from proximal tubule: evidence for reversible Na+-H+ exchange. Am J Physiol. 1981 Oct;241(4):F380–F385. doi: 10.1152/ajprenal.1981.241.4.F380. [DOI] [PubMed] [Google Scholar]
- Thomas J. A., Buchsbaum R. N., Zimniak A., Racker E. Intracellular pH measurements in Ehrlich ascites tumor cells utilizing spectroscopic probes generated in situ. Biochemistry. 1979 May 29;18(11):2210–2218. doi: 10.1021/bi00578a012. [DOI] [PubMed] [Google Scholar]
- Warnock D. G., Reenstra W. W., Yee V. J. Na+/H+ antiporter of brush border vesicles: studies with acridine orange uptake. Am J Physiol. 1982 Jun;242(6):F733–F739. doi: 10.1152/ajprenal.1982.242.6.F733. [DOI] [PubMed] [Google Scholar]
- Yoshitomi K., Burckhardt B. C., Frömter E. Rheogenic sodium-bicarbonate cotransport in the peritubular cell membrane of rat renal proximal tubule. Pflugers Arch. 1985 Dec;405(4):360–366. doi: 10.1007/BF00595689. [DOI] [PubMed] [Google Scholar]
- Yoshitomi K., Frömter E. Cell pH of rat renal proximal tubule in vivo and the conductive nature of peritubular HCO3- (OH-) exit. Pflugers Arch. 1984 Nov;402(3):300–305. doi: 10.1007/BF00585513. [DOI] [PubMed] [Google Scholar]