Abstract
To determine if ischemia induces alterations in renal proximal tubule surface membranes, brush border (BBM) and basolateral membranes (BLM) were isolated simultaneously from the same cortical homogenate after 50 min of renal pedicle clamping. Ischemia caused a selective decrease in the specific activity of BBM marker enzymes leucine aminopeptidase and alkaline phosphatase, but did not effect enrichment (15 times). Neither specific activity nor enrichment (10 times) of BLM NaK-ATPase was altered by ischemia. Contamination of BBM by intracellular organelles was also unchanged, but there was an increase in the specific activity (41.1 vs. 60.0, P less than 0.01) and enrichment (2.3 vs. 4.3, P less than 0.01) of NaK-ATPase in the ischemic BBM fraction. Ischemia increased BLM lysophosphatidylcholine (1.3 vs. 2.5%, P less than 0.05) and phosphatidic acid (0.4 vs. 1.3%, P less than 0.05). Ischemia also decreased BBM sphingomyelin (38.5 vs. 29.6%, P less than 0.01) and phosphatidylserine (16.1 vs. 11.4%, P less than 0.01), and increased phosphatidylcholine (17.2 vs. 29.7%, P less than 0.01), phosphatidylinositol (1.8 vs. 4.6%, P less than 0.01), and lysophosphatidylcholine (1.0 vs. 1.8%, P less than 0.05). The large changes in BBM phospholipids did not result from new phospholipid synthesis, since the specific activity (32P dpm/nmol Pi) of prelabeled individual and total phospholipids was unaltered by ischemia. We next evaluated if these changes were due to inability of ischemic cells to maintain surface membrane polarity. Cytochemical evaluation showed that while NaK-ATPase could be detected only in control BLM, specific deposits of reaction product were present in the BBM of ischemic kidneys. Furthermore, using continuous sucrose gradients, the enzymatic profile of ischemic BBM NaK-ATPase shifted away from ischemic BLM NaK-ATPase and toward the BBM enzymatic marker leucine aminopeptidase. Taken together, these data suggest that NaK-ATPase activity determined enzymatically and cytochemically was located within ischemic BBM. We propose that ischemia impairs the ability of cells to maintain surface membrane polarity, and also results in the accumulation of putative calcium ionophores.
Full text
PDF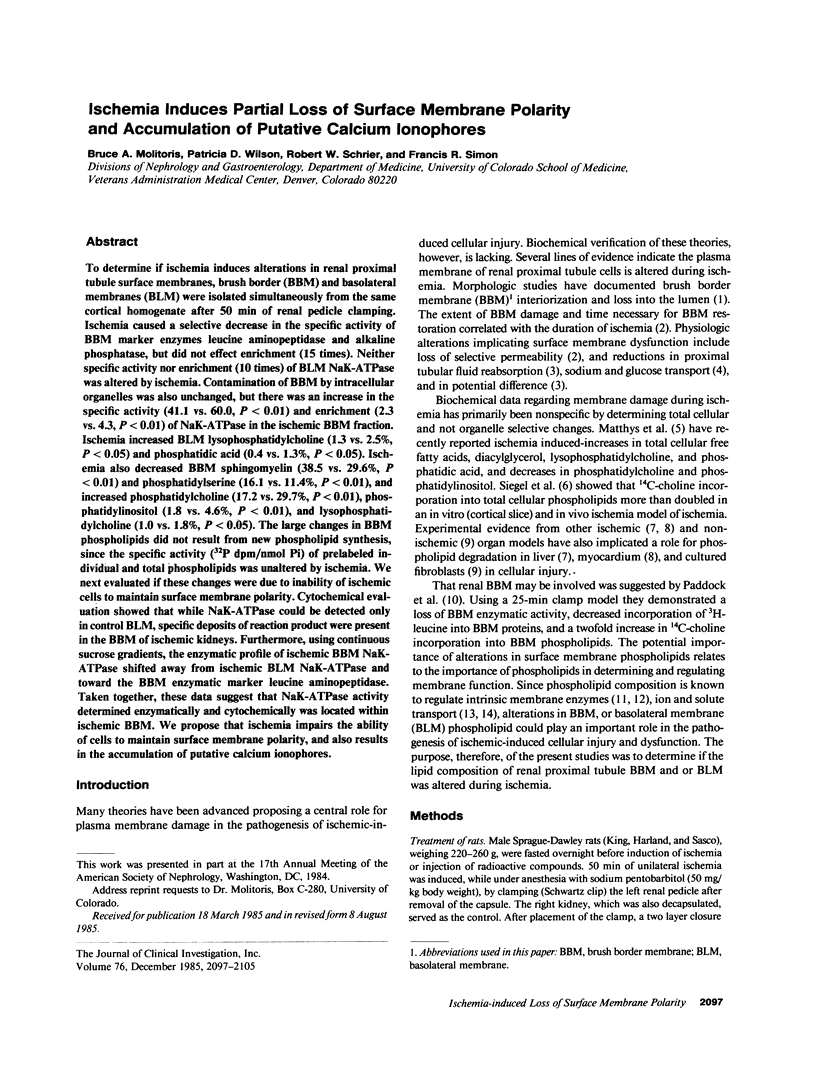
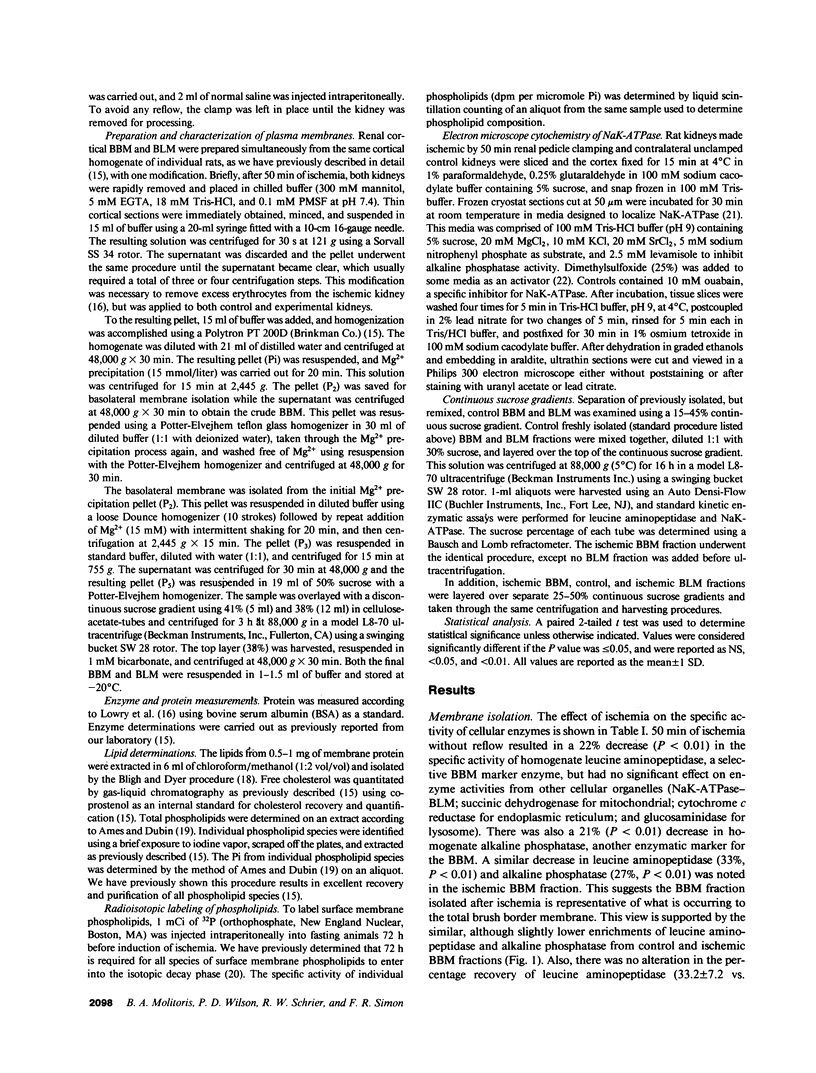
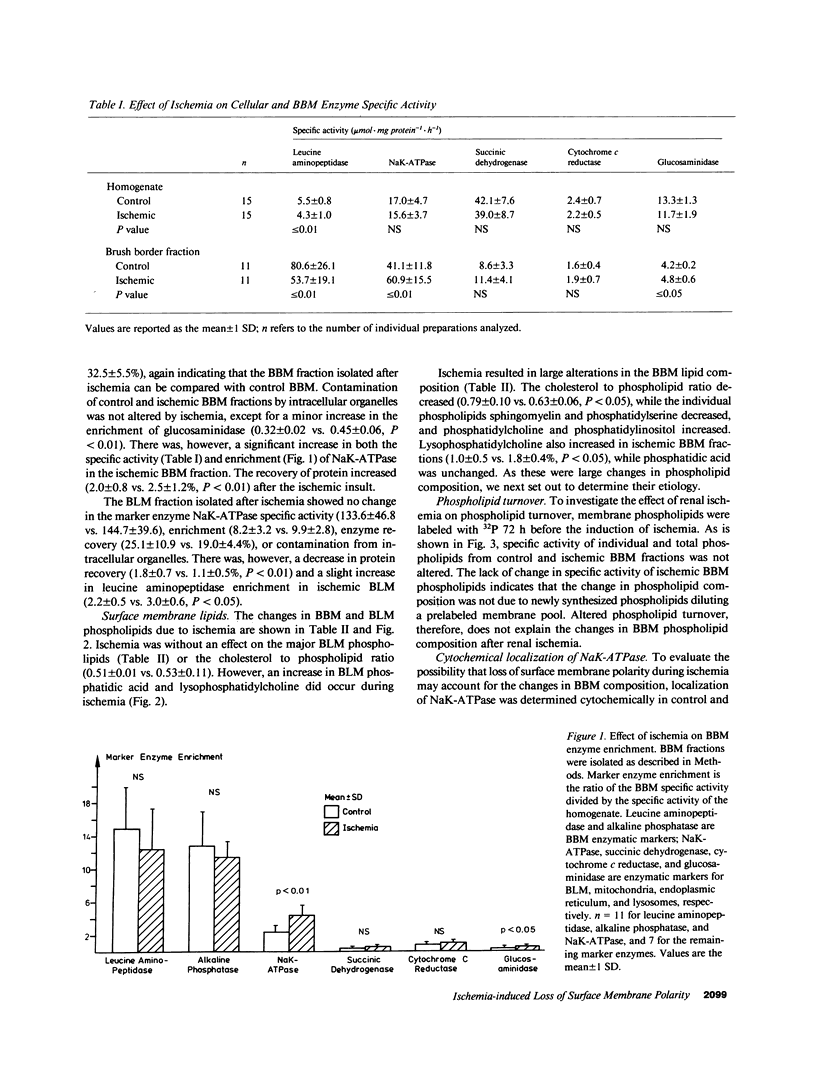
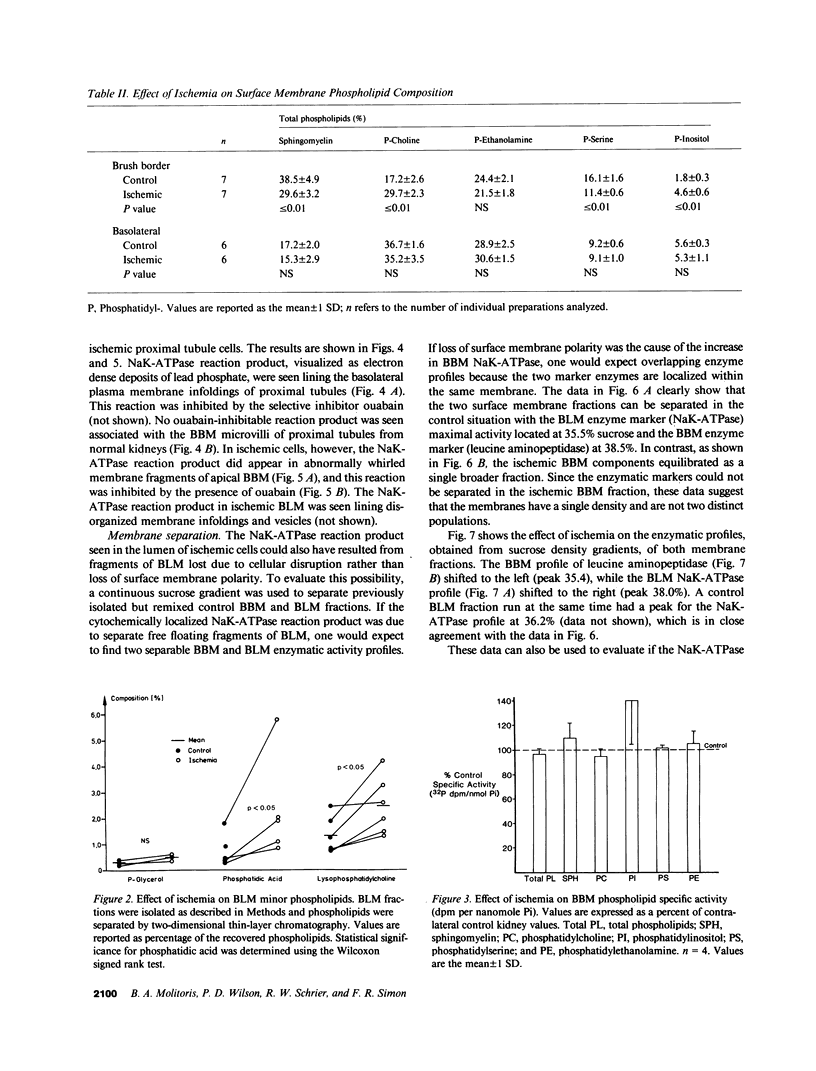
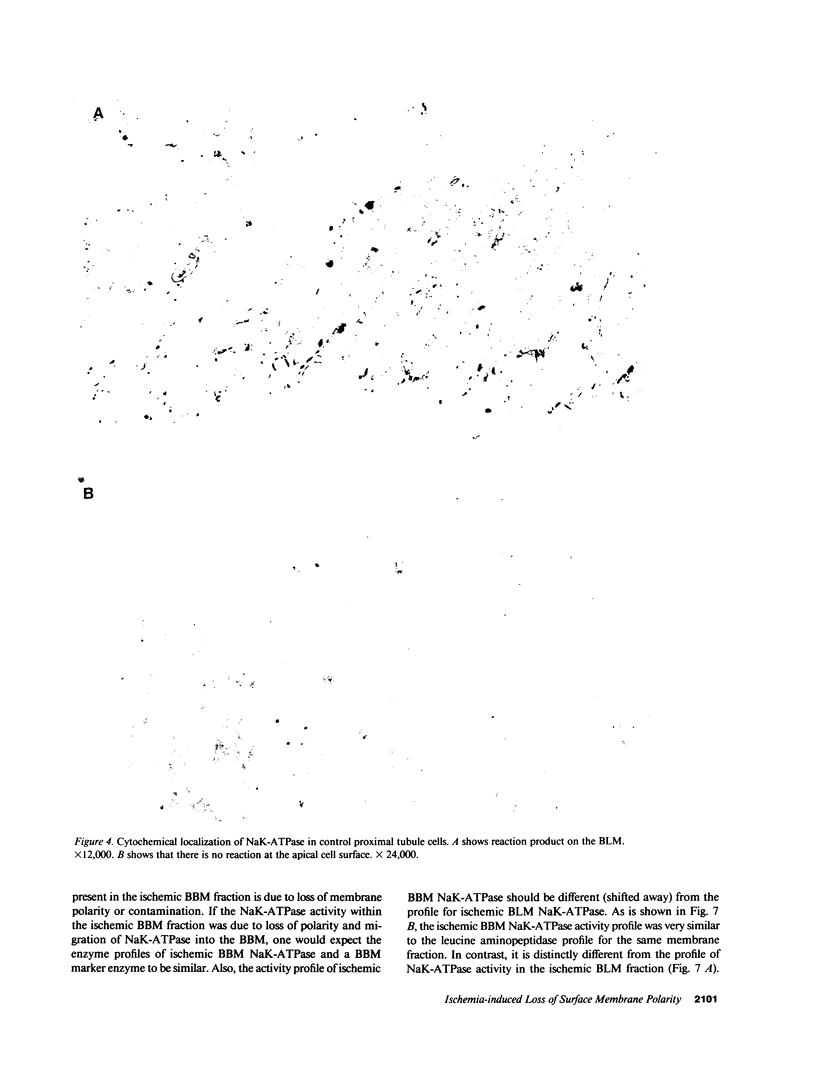
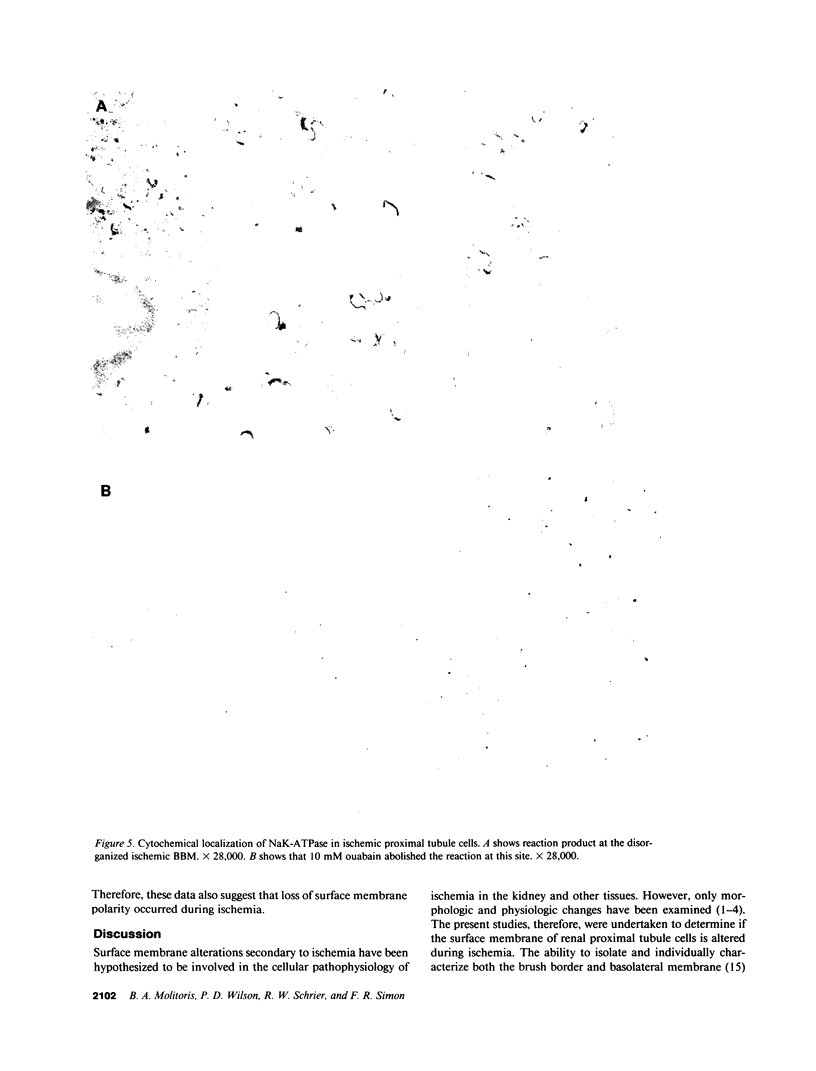
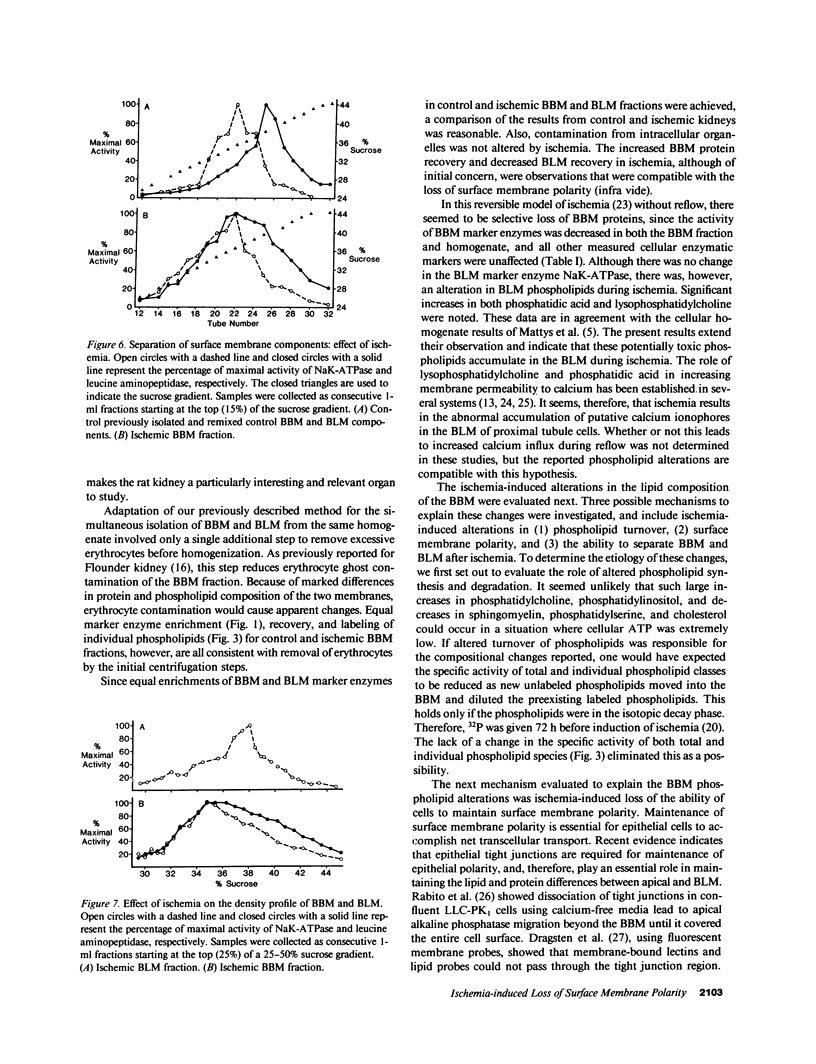
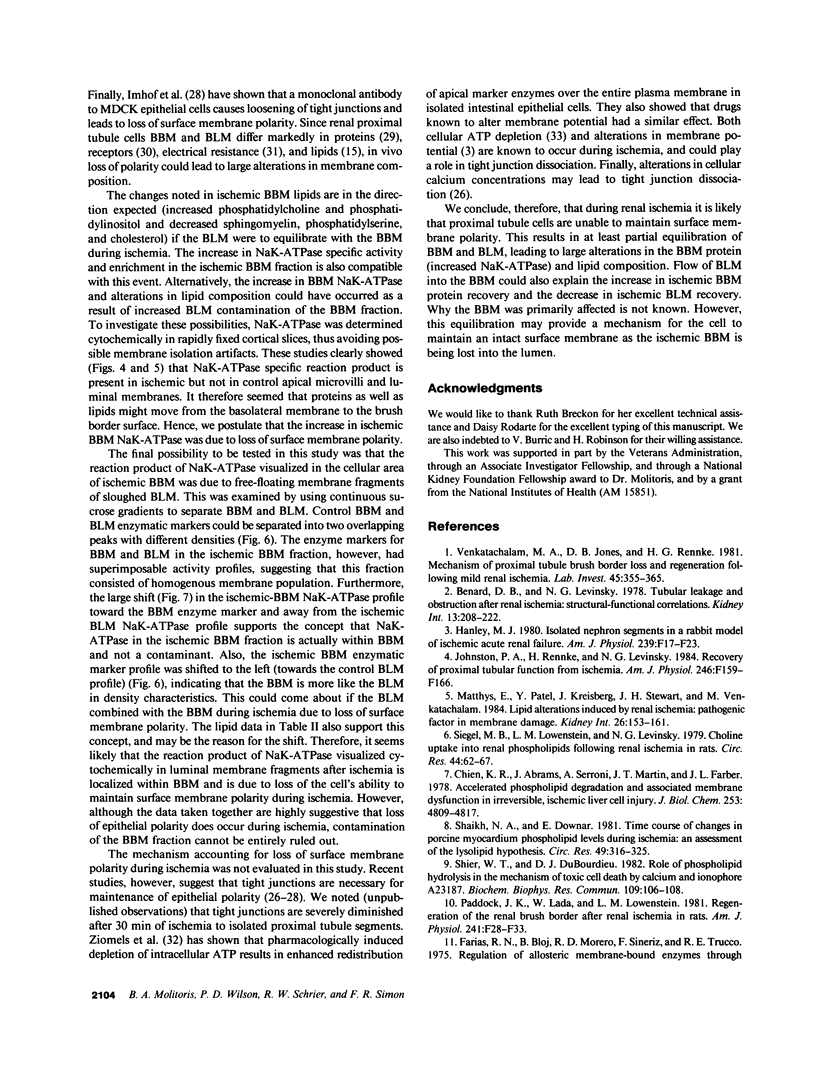
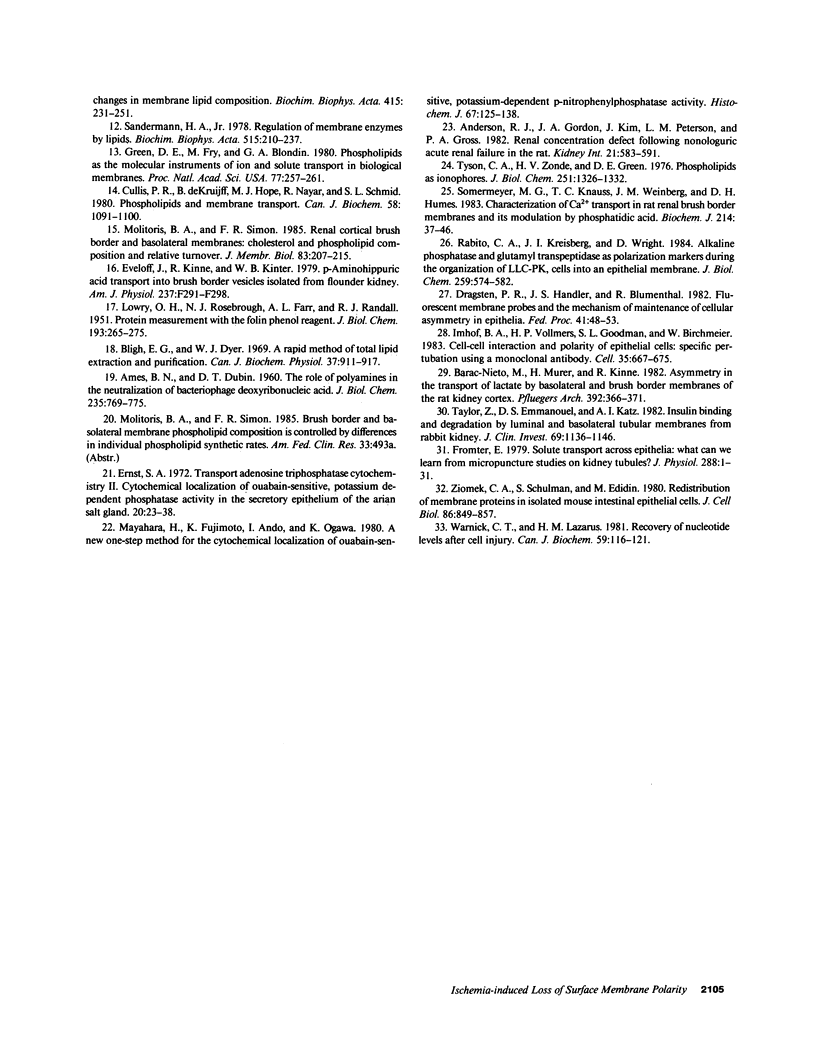
Images in this article
Selected References
These references are in PubMed. This may not be the complete list of references from this article.
- AMES B. N., DUBIN D. T. The role of polyamines in the neutralization of bacteriophage deoxyribonucleic acid. J Biol Chem. 1960 Mar;235:769–775. [PubMed] [Google Scholar]
- Anderson R. J., Gordon J. A., Kim J., Peterson L. M., Gross P. A. Renal concentration defect following nonoliguric acute renal failure in the rat. Kidney Int. 1982 Apr;21(4):583–591. doi: 10.1038/ki.1982.65. [DOI] [PubMed] [Google Scholar]
- BLIGH E. G., DYER W. J. A rapid method of total lipid extraction and purification. Can J Biochem Physiol. 1959 Aug;37(8):911–917. doi: 10.1139/o59-099. [DOI] [PubMed] [Google Scholar]
- Barac-Nieto M., Murer H., Kinne R. Asymmetry in the transport of lactate by basolateral and brush border membranes of rat kidney cortex. Pflugers Arch. 1982 Feb;392(4):366–371. doi: 10.1007/BF00581633. [DOI] [PubMed] [Google Scholar]
- Chien K. R., Abrams J., Serroni A., Martin J. T., Farber J. L. Accelerated phospholipid degradation and associated membrane dysfunction in irreversible, ischemic liver cell injury. J Biol Chem. 1978 Jul 10;253(13):4809–4817. [PubMed] [Google Scholar]
- Cullis P. R., de Kruijff B., Hope M. J., Nayar R., Schmid S. L. Phospholipids and membrane transport. Can J Biochem. 1980 Oct;58(10):1091–1100. doi: 10.1139/o80-147. [DOI] [PubMed] [Google Scholar]
- Donohoe J. F., Venkatachalam M. A., Bernard D. B., Levinsky N. G. Tubular leakage and obstruction after renal ischemia: structural-functional correlations. Kidney Int. 1978 Mar;13(3):208–222. doi: 10.1038/ki.1978.31. [DOI] [PubMed] [Google Scholar]
- Dragsten P. R., Handler J. S., Blumenthal R. Fluorescent membrane probes and the mechanism of maintenance of cellular asymmetry in epithelia. Fed Proc. 1982 Jan;41(1):48–53. [PubMed] [Google Scholar]
- Ernst S. A. Transport adenosine triphosphatase cytochemistry. II. Cytochemical localization of ouabin-sensitive, potassium-dependent phosphatase activity in the secretory epithelium of the avian salt gland. J Histochem Cytochem. 1972 Jan;20(1):23–38. doi: 10.1177/20.1.23. [DOI] [PubMed] [Google Scholar]
- Eveloff J., Kinne R., Kinter W. B. p-Aminohippuric acid transport into brush border vesicles isolated from flounder kidney. Am J Physiol. 1979 Oct;237(4):F291–F298. doi: 10.1152/ajprenal.1979.237.4.F291. [DOI] [PubMed] [Google Scholar]
- Frömter E. The Feldberg Lecture 1976. Solute transport across epithelia: what can we learn from micropuncture studies in kidney tubules? J Physiol. 1979 Mar;288:1–31. [PMC free article] [PubMed] [Google Scholar]
- Green D. E., Fry M., Blondin G. A. Phospholipids as the molecular instruments of ion and solute transport in biological membranes. Proc Natl Acad Sci U S A. 1980 Jan;77(1):257–261. doi: 10.1073/pnas.77.1.257. [DOI] [PMC free article] [PubMed] [Google Scholar]
- Hanley M. J. Isolated nephron segments in a rabbit model of ischemic acute renal failure. Am J Physiol. 1980 Jul;239(1):F17–F23. doi: 10.1152/ajprenal.1980.239.1.F17. [DOI] [PubMed] [Google Scholar]
- Imhof B. A., Vollmers H. P., Goodman S. L., Birchmeier W. Cell-cell interaction and polarity of epithelial cells: specific perturbation using a monoclonal antibody. Cell. 1983 Dec;35(3 Pt 2):667–675. doi: 10.1016/0092-8674(83)90099-5. [DOI] [PubMed] [Google Scholar]
- Johnston P. A., Rennke H., Levinsky N. G. Recovery of proximal tubular function from ischemic injury. Am J Physiol. 1984 Feb;246(2 Pt 2):F159–F166. doi: 10.1152/ajprenal.1984.246.2.F159. [DOI] [PubMed] [Google Scholar]
- LOWRY O. H., ROSEBROUGH N. J., FARR A. L., RANDALL R. J. Protein measurement with the Folin phenol reagent. J Biol Chem. 1951 Nov;193(1):265–275. [PubMed] [Google Scholar]
- Matthys E., Patel Y., Kreisberg J., Stewart J. H., Venkatachalam M. Lipid alterations induced by renal ischemia: pathogenic factor in membrane damage. Kidney Int. 1984 Aug;26(2):153–161. doi: 10.1038/ki.1984.149. [DOI] [PubMed] [Google Scholar]
- Mayahara H., Fujimoto K., Ando T., Ogawa K. A new one-step method for the cytochemical localization of ouabain-sensitive, potassium-dependent p-nitrophenylphosphatase activity. Histochemistry. 1980;67(2):125–138. doi: 10.1007/BF00493231. [DOI] [PubMed] [Google Scholar]
- Molitoris B. A., Simon F. R. Renal cortical brush-border and basolateral membranes: cholesterol and phospholipid composition and relative turnover. J Membr Biol. 1985;83(3):207–215. doi: 10.1007/BF01868695. [DOI] [PubMed] [Google Scholar]
- Paddock J. K., Lada W., Lowenstein L. M. Regeneration of the renal brush border after renal ischemia in rats. Am J Physiol. 1981 Jul;241(1):F28–F33. doi: 10.1152/ajprenal.1981.241.1.F28. [DOI] [PubMed] [Google Scholar]
- Rabito C. A., Kreisberg J. I., Wight D. Alkaline phosphatase and gamma-glutamyl transpeptidase as polarization markers during the organization of LLC-PK1 cells into an epithelial membrane. J Biol Chem. 1984 Jan 10;259(1):574–582. [PubMed] [Google Scholar]
- Sandermann H., Jr Regulation of membrane enzymes by lipids. Biochim Biophys Acta. 1978 Sep 29;515(3):209–237. doi: 10.1016/0304-4157(78)90015-1. [DOI] [PubMed] [Google Scholar]
- Shaikh N. A., Downar E. Time course of changes in porcine myocardial phospholipid levels during ischemia. A reassessment of the lysolipid hypothesis. Circ Res. 1981 Aug;49(2):316–325. doi: 10.1161/01.res.49.2.316. [DOI] [PubMed] [Google Scholar]
- Shier W. T., DuBourdieu D. J. Role of phospholipid hydrolysis in the mechanism of toxic cell death by calcium and ionophore A23187. Biochem Biophys Res Commun. 1982 Nov 16;109(1):106–112. doi: 10.1016/0006-291x(82)91572-8. [DOI] [PubMed] [Google Scholar]
- Siegel M. B., Lowenstein L. M., Levinsky N. G. Choline uptake into renal phospholipids following renal ischemia in rats. Circ Res. 1979 Jan;44(1):62–67. doi: 10.1161/01.res.44.1.62. [DOI] [PubMed] [Google Scholar]
- Somermeyer M. G., Knauss T. C., Weinberg J. M., Humes H. D. Characterization of Ca2+ transport in rat renal brush-border membranes and its modulation by phosphatidic acid. Biochem J. 1983 Jul 15;214(1):37–46. doi: 10.1042/bj2140037. [DOI] [PMC free article] [PubMed] [Google Scholar]
- Talor Z., Emmanouel D. S., Katz A. I. Insulin binding and degradation by luminal and basolateral tubular membranes from rabbit kidney. J Clin Invest. 1982 May;69(5):1136–1146. doi: 10.1172/JCI110549. [DOI] [PMC free article] [PubMed] [Google Scholar]
- Tyson C. A., Vande Zande H., Green D. E. Phospholipids as ionophores. J Biol Chem. 1976 Mar 10;251(5):1326–1332. [PubMed] [Google Scholar]
- Venkatachalam M. A., Jones D. B., Rennke H. G., Sandstrom D., Patel Y. Mechanism of proximal tubule brush border loss and regeneration following mild renal ischemia. Lab Invest. 1981 Oct;45(4):355–365. [PubMed] [Google Scholar]
- Warnick C. T., Lazarus H. M. Recovery of nucleotide levels after cell injury. Can J Biochem. 1981 Feb;59(2):116–121. doi: 10.1139/o81-017. [DOI] [PubMed] [Google Scholar]
- Ziomek C. A., Schulman S., Edidin M. Redistribution of membrane proteins in isolated mouse intestinal epithelial cells. J Cell Biol. 1980 Sep;86(3):849–857. doi: 10.1083/jcb.86.3.849. [DOI] [PMC free article] [PubMed] [Google Scholar]