Abstract
Three allelic mutants of Arabidopsis thaliana which lack mitochondrial serine transhydroxymethylase activity due to a recessive nuclear mutation have been characterized. The mutants were shown to be deficient both in glycine decarboxylation and in the conversion of glycine to serine. Glycine accumulated as an end product of photosynthesis in the mutants, largely at the expense of serine, starch, and sucrose formation. The mutants photorespired CO2 at low rates in the light, but this evolution of photorespiratory CO2 was abolished by provision of exogenous NH3. Exogenous NH3 was required by the mutants for continued synthesis of glycine under photorespiratory conditions. These and related results with wild-type Arabidopsis suggested that glycine decarboxylation is the sole site of photorespiratory CO2 release in wild-type plants but that depletion of the amino donors required for glyoxylate amination may lead to CO2 release from direct decarboxylation of glyoxylate. Photosynthetic CO2 fixation was inhibited in the mutants under atmospheric conditions which promote photorespiration but could be partially restored by exogenous NH3. The magnitude of the NH3 stimulation of photosynthesis indicated that the increase was due to the suppression of glyoxylate decarboxylation. The normal growth of the mutants under nonphotorespiratory atmospheric conditions indicates that mitochondrial serine transhydroxymethylase is not required in C3 plants for any function unrelated to photorespiration.
Full text
PDF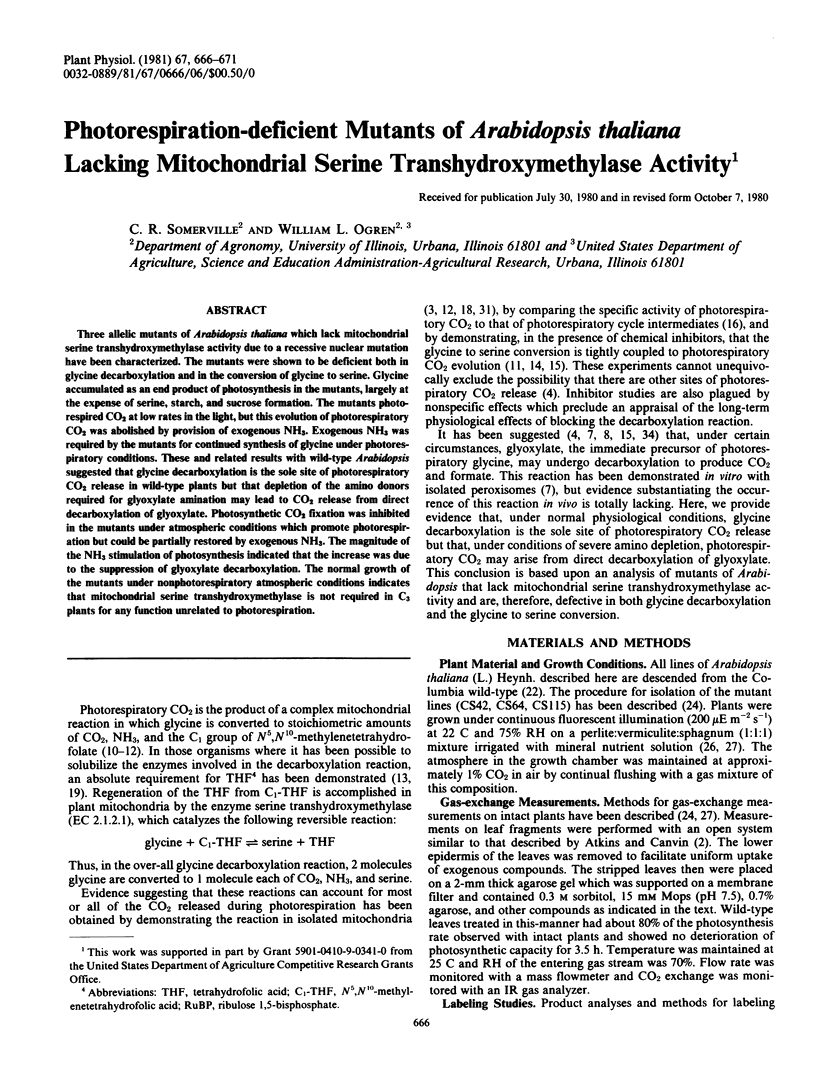
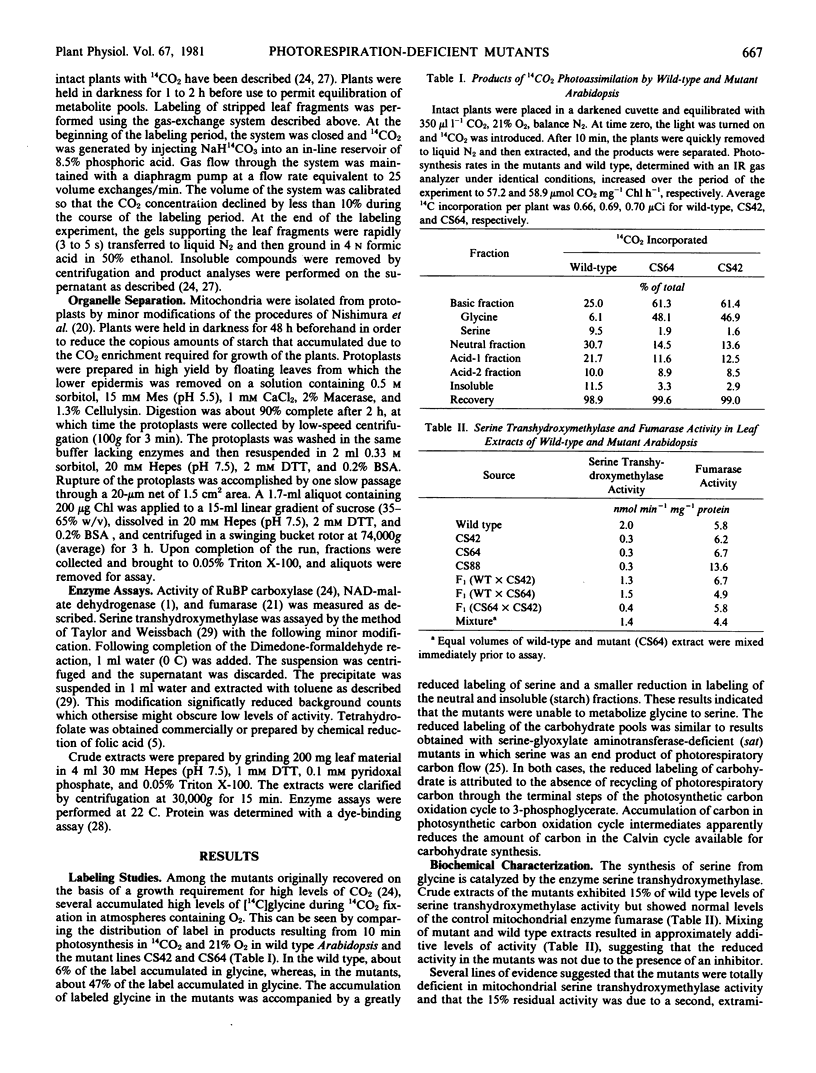
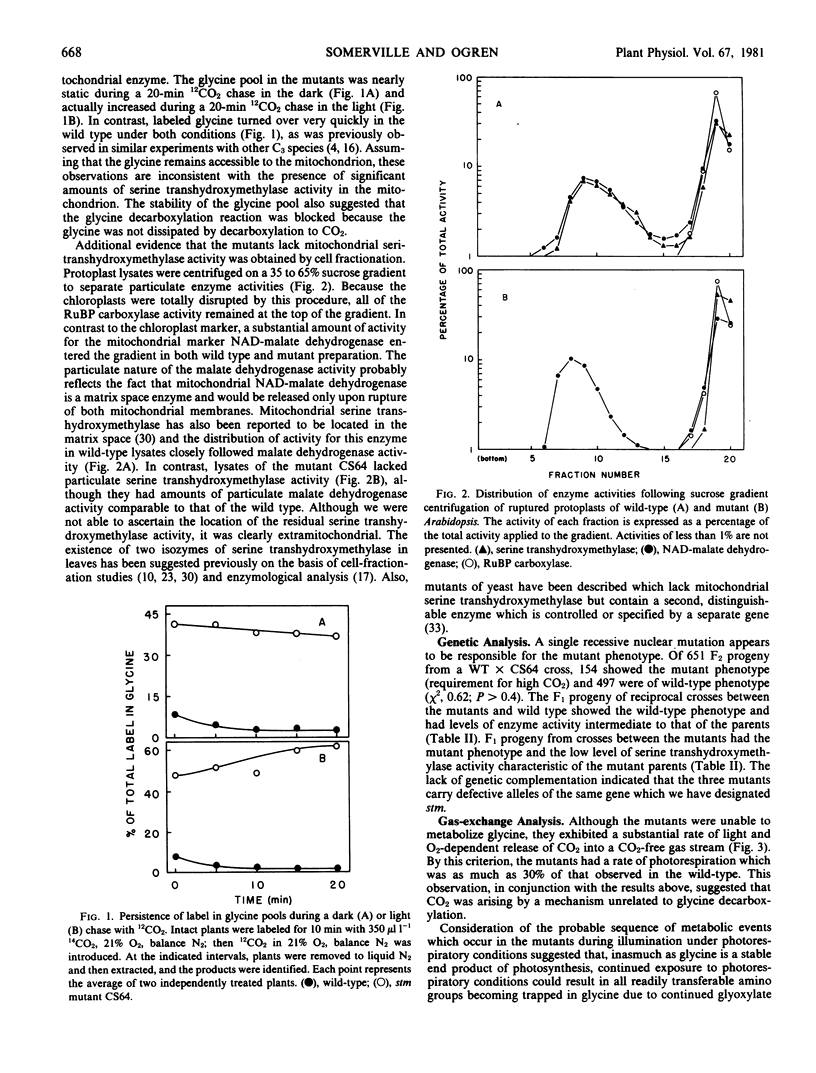
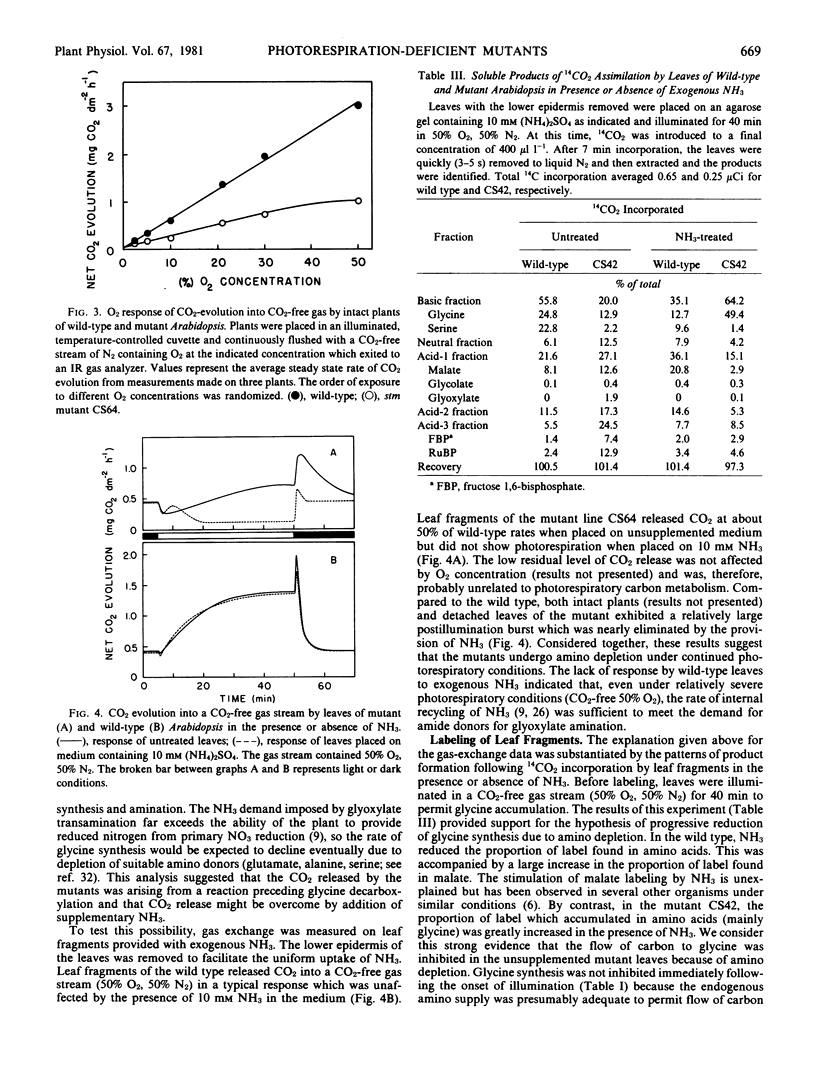
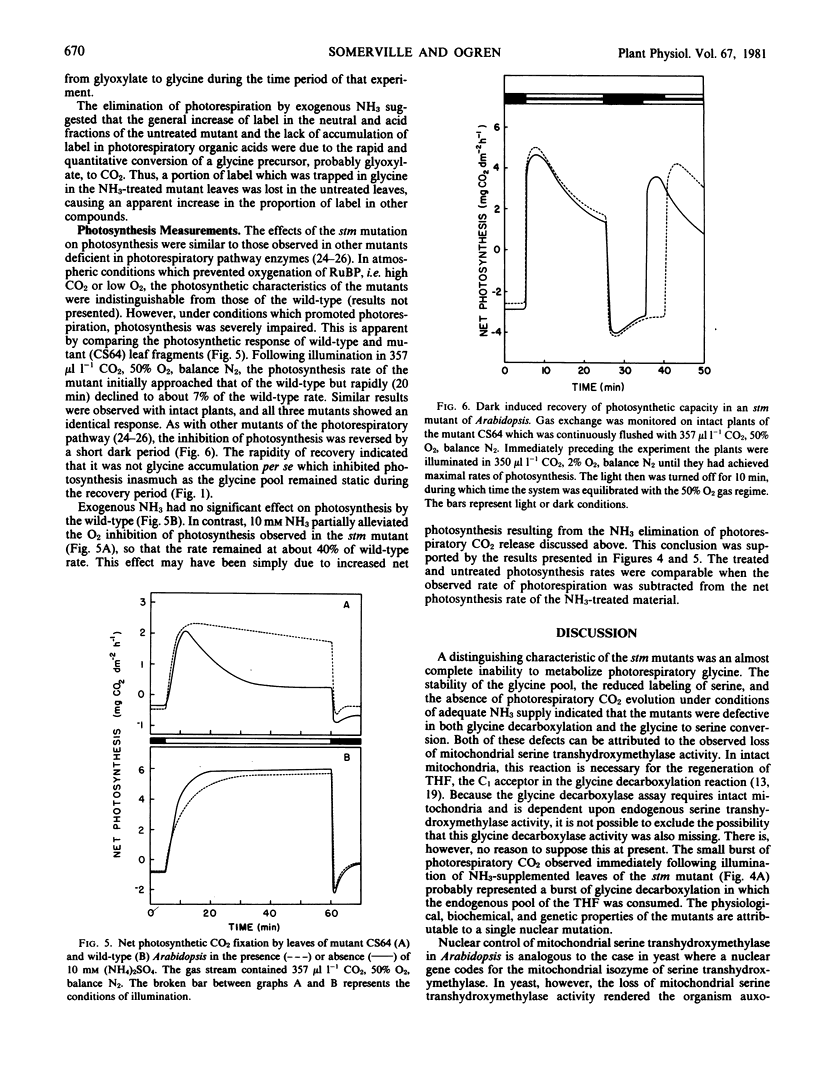
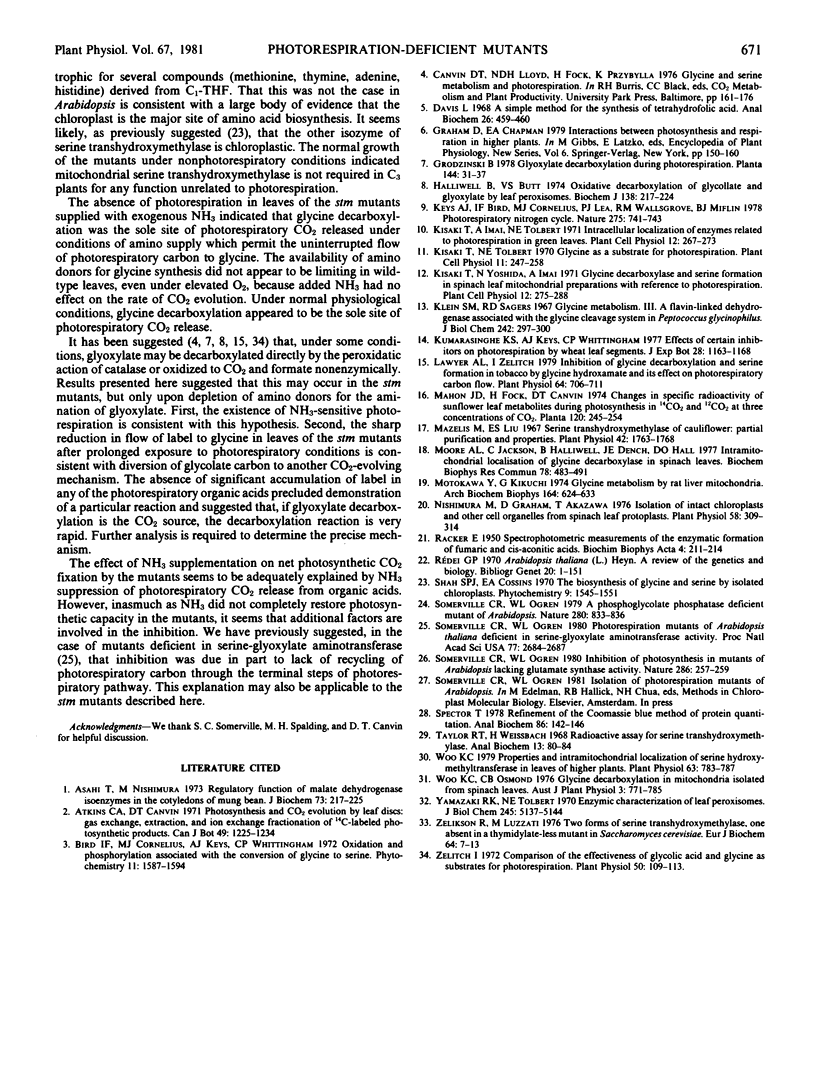
Selected References
These references are in PubMed. This may not be the complete list of references from this article.
- Asahi T., Nishimura M. Regulatory function of malate dehydrogenase isoenzymes in the cotyledons of mung bean. J Biochem. 1973 Feb;73(2):217–225. [PubMed] [Google Scholar]
- Davis L. A simple method for the synthesis of tetrahydrofolic acid. Anal Biochem. 1968 Dec;26(3):459–460. doi: 10.1016/0003-2697(68)90211-x. [DOI] [PubMed] [Google Scholar]
- Halliwell B., Butt V. S. Oxidative decarboxylation of glycollate and glyoxylate by leaf peroxisomes. Biochem J. 1974 Feb;138(2):217–224. doi: 10.1042/bj1380217. [DOI] [PMC free article] [PubMed] [Google Scholar]
- Klein S. M., Sagers R. D. Glycine metabolism. 3. A flavin-linked dehydrogenase associated with the glycine cleavage system in Peptococcus glycinophilus. J Biol Chem. 1967 Jan 25;242(2):297–300. [PubMed] [Google Scholar]
- Lawyer A. L., Zelitch I. Inhibition of glycine decarboxylation and serine formation in tobacco by glycine hydroxamate and its effect on photorespiratory carbon flow. Plant Physiol. 1979 Nov;64(5):706–711. doi: 10.1104/pp.64.5.706. [DOI] [PMC free article] [PubMed] [Google Scholar]
- Mazelis M., Liu E. S. Serine Transhydroxymethylase of Cauliflower (Brassica oleracea var. botrytis L.): Partial Purification and Properties. Plant Physiol. 1967 Dec;42(12):1763–1768. doi: 10.1104/pp.42.12.1763. [DOI] [PMC free article] [PubMed] [Google Scholar]
- Moore A. L., Jackson C., Halliwell B., Dench J. E., Hall D. O. Intramitochondrial localisation of glycine decarboxylase in spinach leaves. Biochem Biophys Res Commun. 1977 Sep 23;78(2):483–491. doi: 10.1016/0006-291x(77)90204-2. [DOI] [PubMed] [Google Scholar]
- Motokawa Y., Kikuchi G. Glycine metabolism by rat liver mitochondria. Reconstruction of the reversible glycine cleavage system with partially purified protein components. Arch Biochem Biophys. 1974 Oct;164(2):624–633. doi: 10.1016/0003-9861(74)90074-5. [DOI] [PubMed] [Google Scholar]
- Nishimura M., Graham D., Akazawa T. Isolation of intact chloroplasts and other cell organelles from spinach leaf protoplasts. Plant Physiol. 1976 Sep;58(3):309–314. doi: 10.1104/pp.58.3.309. [DOI] [PMC free article] [PubMed] [Google Scholar]
- RACKER E. Spectrophotometric measurements of the enzymatic formation of fumaric and cis-aconitic acids. Biochim Biophys Acta. 1950 Jan;4(1-3):211–214. doi: 10.1016/0006-3002(50)90026-6. [DOI] [PubMed] [Google Scholar]
- Somerville C. R., Ogren W. L. Photorespiration mutants of Arabidopsis thaliana deficient in serine-glyoxylate aminotransferase activity. Proc Natl Acad Sci U S A. 1980 May;77(5):2684–2687. doi: 10.1073/pnas.77.5.2684. [DOI] [PMC free article] [PubMed] [Google Scholar]
- Spector T. Refinement of the coomassie blue method of protein quantitation. A simple and linear spectrophotometric assay for less than or equal to 0.5 to 50 microgram of protein. Anal Biochem. 1978 May;86(1):142–146. doi: 10.1016/0003-2697(78)90327-5. [DOI] [PubMed] [Google Scholar]
- Woo K. C. Properties and intramitochondrial localization of serine hydroxymethyltransferase in leaves of higher plants. Plant Physiol. 1979 Apr;63(4):783–787. doi: 10.1104/pp.63.4.783. [DOI] [PMC free article] [PubMed] [Google Scholar]
- Yamazaki R. K., Tolbert N. E. Enzymic characterization of leaf peroxisomes. J Biol Chem. 1970 Oct 10;245(19):5137–5144. [PubMed] [Google Scholar]
- Zelikson R., Luzzati M. Two forms of serine transhydroxymethylase, one absent in a thymidylate-less mutant in Saccharomyces cerevisiae. Eur J Biochem. 1976 Apr 15;64(1):7–13. doi: 10.1111/j.1432-1033.1976.tb10269.x. [DOI] [PubMed] [Google Scholar]
- Zelitch I. Comparison of the effectiveness of glycolic Acid and glycine as substrates for photorespiration. Plant Physiol. 1972 Jul;50(1):109–113. doi: 10.1104/pp.50.1.109. [DOI] [PMC free article] [PubMed] [Google Scholar]