Abstract
The dielectric properties of proteins are central to their stability and activity. We use the Fröhlich-Kirkwood theory of dielectrics to analyze two 1-ns molecular dynamics simulations of ferro- and ferricytochrome c in spherical droplets of 1400 water molecules. Protein and solvent are idealized as a series of concentric, spherical, dielectric media. Analysis results depend strongly on the treatment of the charged protein side chains at the protein/solvent interface. If charged side chains are viewed as part of the protein medium, then the protein dipole fluctuations are dominated by large, mutually uncorrelated, anisotropic, motions of the charged side chains. It is then incorrect to view the protein region as a single, homogeneous dielectric material. If one does take this view, estimates of the protein "dielectric constant" vary from 16 to 37, depending on the exact choice of model parameters. In contrast, if the charged portions of the charged side chains are viewed as part of the solvent medium, then theory and simulation are consistent: the protein dipole fluctuations excluding charged side chains are roughly those of a homogeneous, isotropic dielectric medium, with a dielectric constant of 4.7 +/- 1.0 (ferro) or 3.4 +/- 1.0 (ferri), in agreement with powder experiments. Statistical uncertainty and sensitivity to model parameters are small. Analysis of the radial dependence of the dipole fluctuations suggests that the inner half of the protein has a somewhat lower dielectric constant of 1.5-2, consistent with its biological function in electron transfer. These results suggest that Poisson-Boltzmann models could treat the protein bulk as a low-dielectric medium and the charged surface groups as part of the solvent region.
Full text
PDF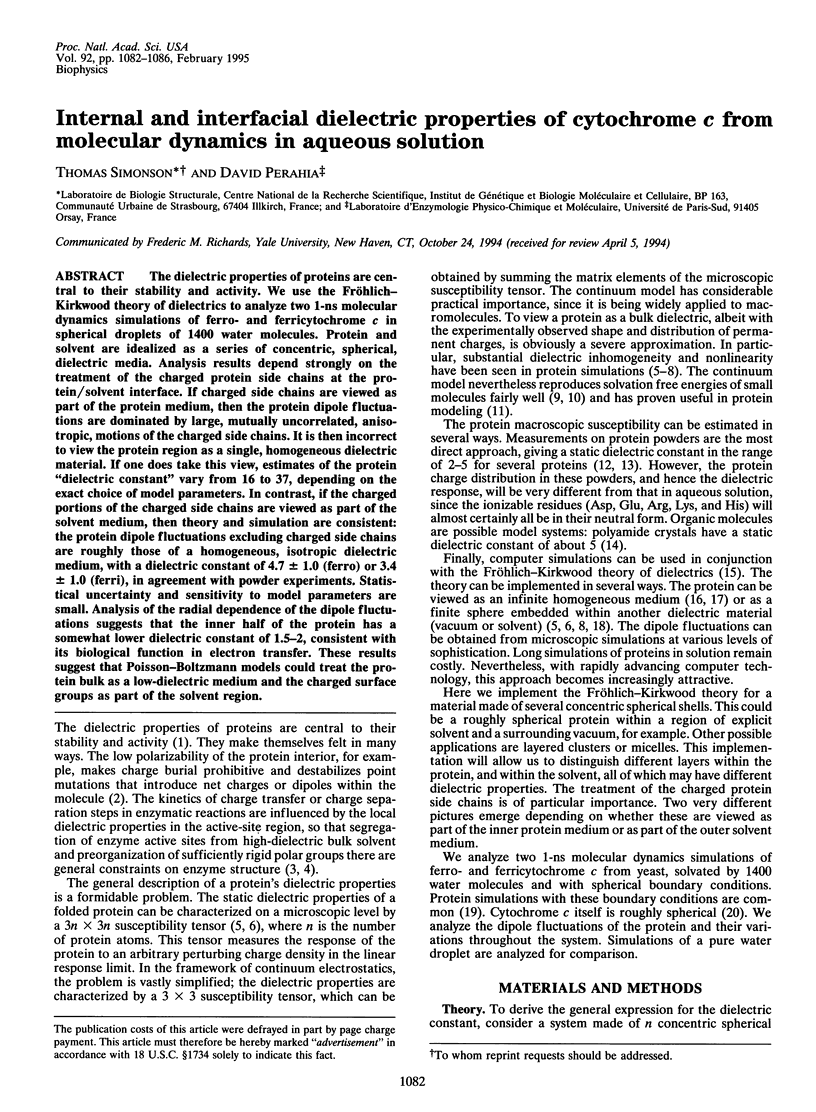
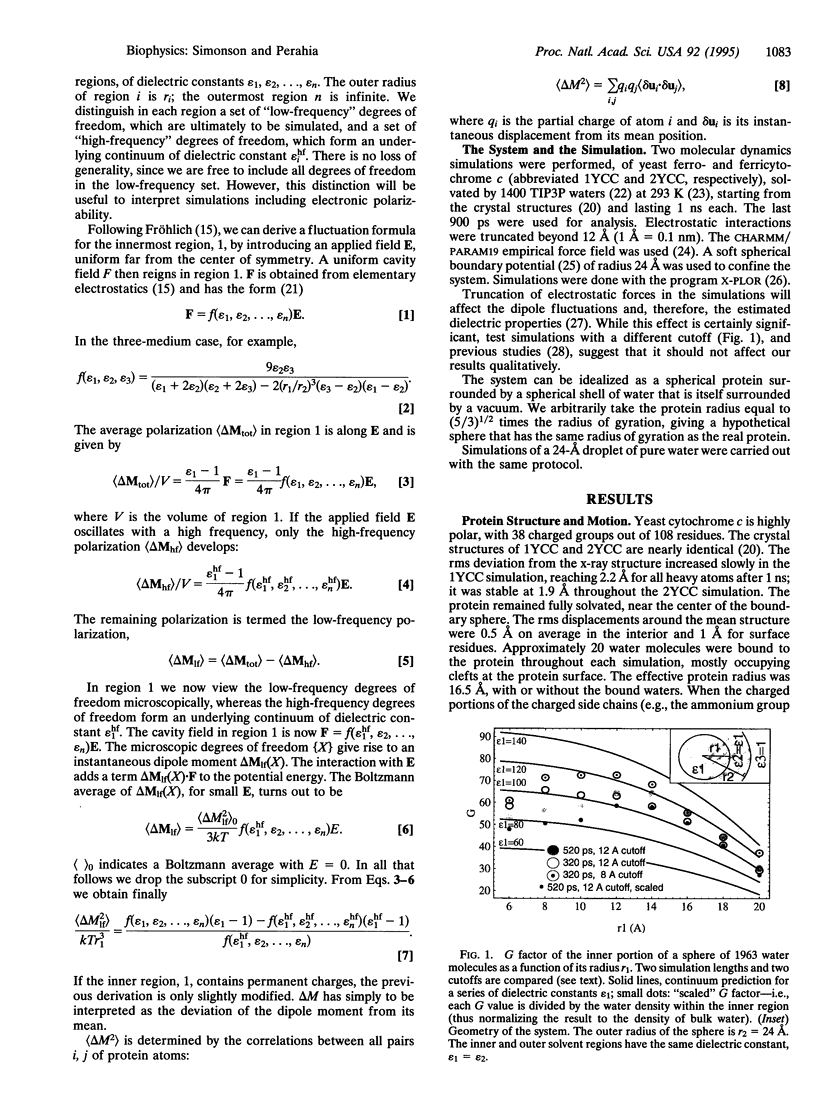
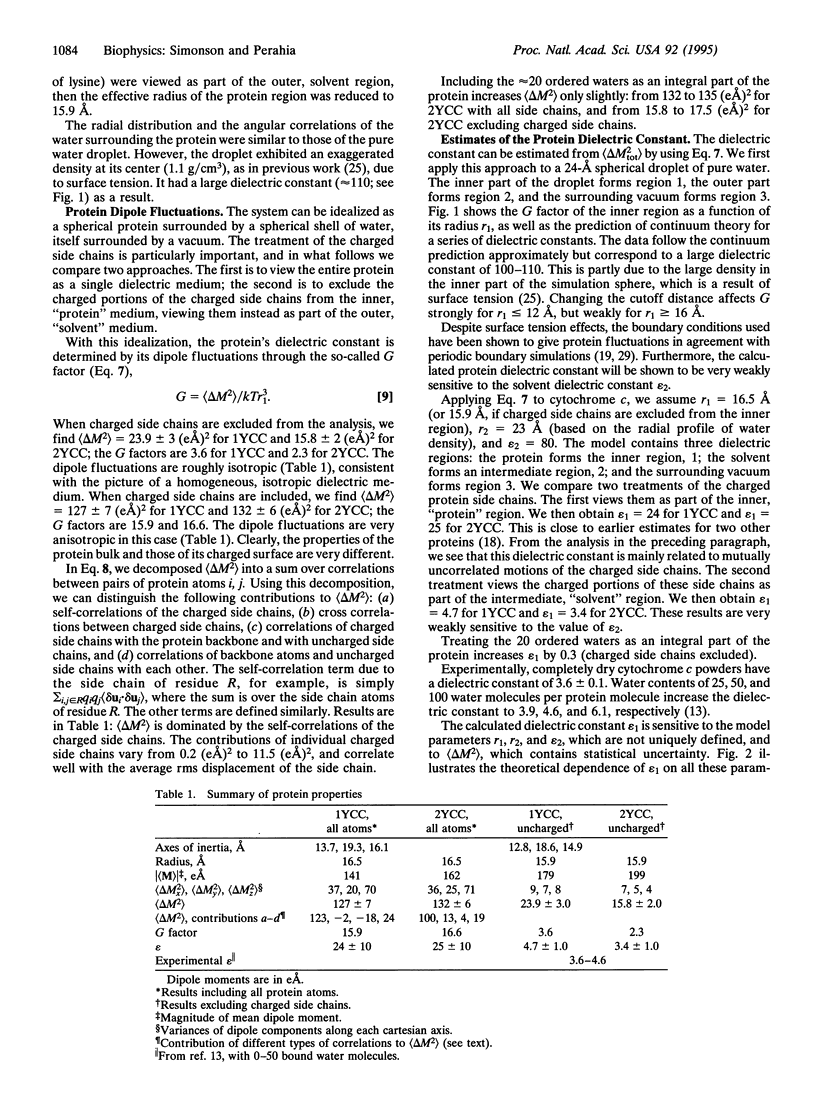
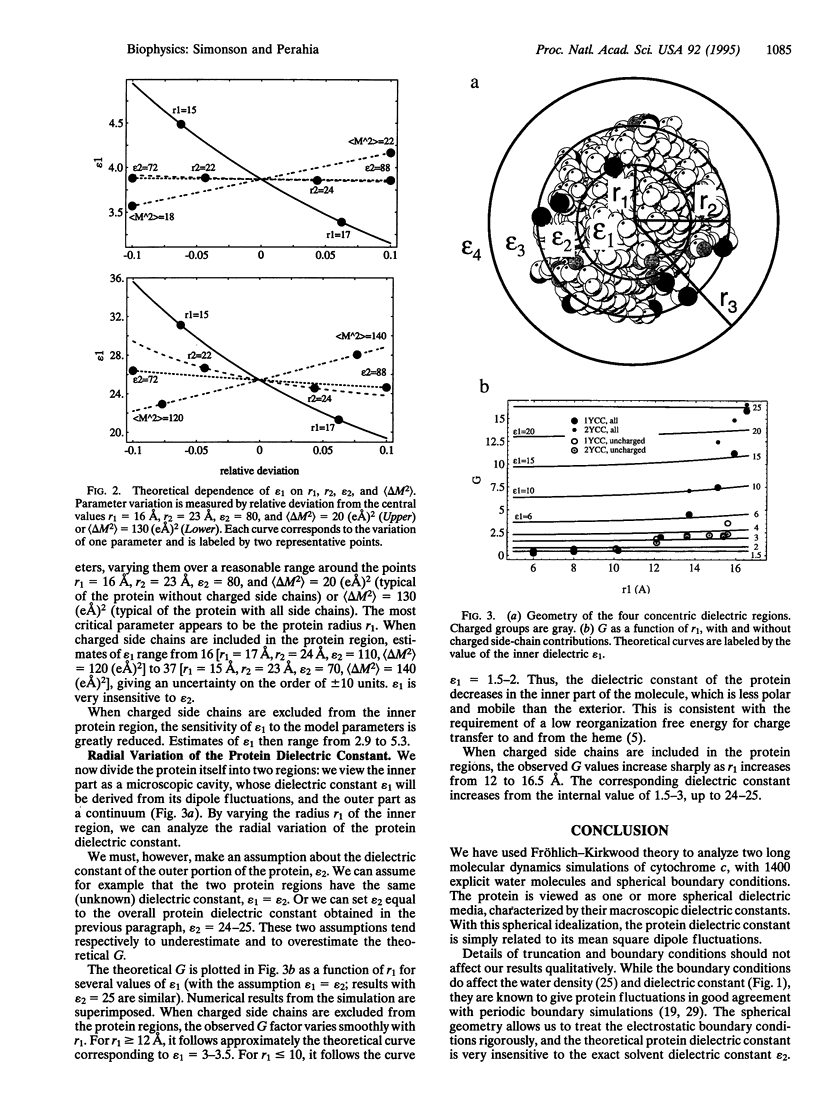
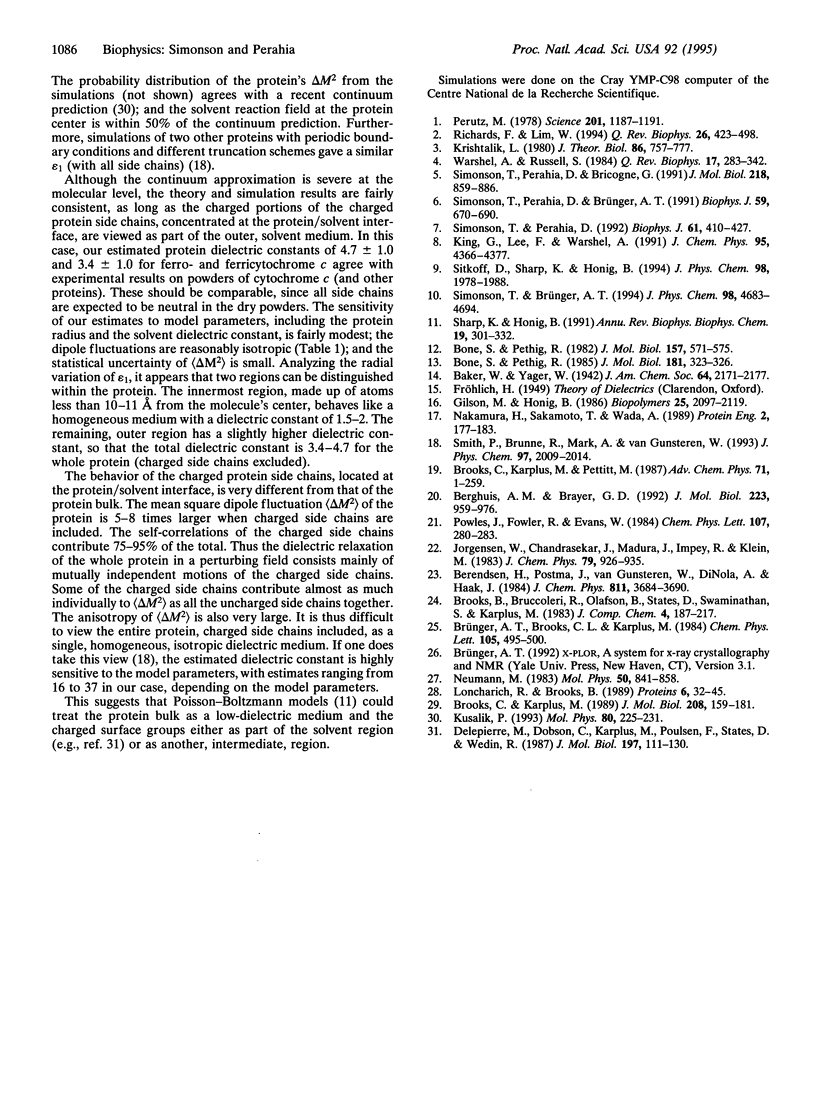
Images in this article
Selected References
These references are in PubMed. This may not be the complete list of references from this article.
- Berghuis A. M., Brayer G. D. Oxidation state-dependent conformational changes in cytochrome c. J Mol Biol. 1992 Feb 20;223(4):959–976. doi: 10.1016/0022-2836(92)90255-i. [DOI] [PubMed] [Google Scholar]
- Bone S., Pethig R. Dielectric studies of protein hydration and hydration-induced flexibility. J Mol Biol. 1985 Jan 20;181(2):323–326. doi: 10.1016/0022-2836(85)90096-8. [DOI] [PubMed] [Google Scholar]
- Bone S., Pethig R. Dielectric studies of the binding of water to lysozyme. J Mol Biol. 1982 May 25;157(3):571–575. doi: 10.1016/0022-2836(82)90477-6. [DOI] [PubMed] [Google Scholar]
- Brooks C. L., 3rd, Karplus M. Solvent effects on protein motion and protein effects on solvent motion. Dynamics of the active site region of lysozyme. J Mol Biol. 1989 Jul 5;208(1):159–181. doi: 10.1016/0022-2836(89)90093-4. [DOI] [PubMed] [Google Scholar]
- Delepierre M., Dobson C. M., Karplus M., Poulsen F. M., States D. J., Wedin R. E. Electrostatic effects and hydrogen exchange behaviour in proteins. The pH dependence of exchange rates in lysozyme. J Mol Biol. 1987 Sep 5;197(1):111–130. doi: 10.1016/0022-2836(87)90613-9. [DOI] [PubMed] [Google Scholar]
- Gilson M. K., Honig B. H. The dielectric constant of a folded protein. Biopolymers. 1986 Nov;25(11):2097–2119. doi: 10.1002/bip.360251106. [DOI] [PubMed] [Google Scholar]
- Krishtalik L. I. Catalytic acceleration of reactions by enzymes. Effect of screening of a polar medium by a protein globule. J Theor Biol. 1980 Oct 21;86(4):757–771. doi: 10.1016/0022-5193(80)90309-4. [DOI] [PubMed] [Google Scholar]
- Loncharich R. J., Brooks B. R. The effects of truncating long-range forces on protein dynamics. Proteins. 1989;6(1):32–45. doi: 10.1002/prot.340060104. [DOI] [PubMed] [Google Scholar]
- Nakamura H., Sakamoto T., Wada A. A theoretical study of the dielectric constant of protein. Protein Eng. 1988 Sep;2(3):177–183. doi: 10.1093/protein/2.3.177. [DOI] [PubMed] [Google Scholar]
- Perutz M. F. Electrostatic effects in proteins. Science. 1978 Sep 29;201(4362):1187–1191. doi: 10.1126/science.694508. [DOI] [PubMed] [Google Scholar]
- Richards F. M., Lim W. A. An analysis of packing in the protein folding problem. Q Rev Biophys. 1993 Nov;26(4):423–498. doi: 10.1017/s0033583500002845. [DOI] [PubMed] [Google Scholar]
- Sharp K. A., Honig B. Electrostatic interactions in macromolecules: theory and applications. Annu Rev Biophys Biophys Chem. 1990;19:301–332. doi: 10.1146/annurev.bb.19.060190.001505. [DOI] [PubMed] [Google Scholar]
- Simonson T., Perahia D., Bricogne G. Intramolecular dielectric screening in proteins. J Mol Biol. 1991 Apr 20;218(4):859–886. doi: 10.1016/0022-2836(91)90273-9. [DOI] [PubMed] [Google Scholar]
- Simonson T., Perahia D., Brünger A. T. Microscopic theory of the dielectric properties of proteins. Biophys J. 1991 Mar;59(3):670–690. doi: 10.1016/S0006-3495(91)82282-2. [DOI] [PMC free article] [PubMed] [Google Scholar]
- Simonson T., Perahia D. Normal modes of symmetric protein assemblies. Application to the tobacco mosaic virus protein disk. Biophys J. 1992 Feb;61(2):410–427. doi: 10.1016/S0006-3495(92)81847-7. [DOI] [PMC free article] [PubMed] [Google Scholar]
- Warshel A., Russell S. T. Calculations of electrostatic interactions in biological systems and in solutions. Q Rev Biophys. 1984 Aug;17(3):283–422. doi: 10.1017/s0033583500005333. [DOI] [PubMed] [Google Scholar]