Abstract
A “temporal gradient apparatus” has been developed that allows the motility of bacteria to be studied after they have been subjected to a sudden change from one uniform concentration of attractant to another. A sudden decrease elicits the tumbling response observed with spatial gradients; it was found, however, that a sudden increase also elicits a response, namely supercoordinated swimming. This demonstrates that chemotaxis is achieved by modulation of the incidence of tumbling both above and below its steady-state value. The initial responses gradually revert to the steady-state motility pattern characteristic of a uniform distribution of attractant. The apparent detection of a spatial gradient by the bacteria therefore involves an actual detection of a temporal gradient experienced as a result of movement through space. Potential models for the chemotactic response based on some “memory” mechanism are discussed.
Keywords: temporal gradient apparatus, stopped-flow, S. typhimurium, motility tracks, memory
Full text
PDF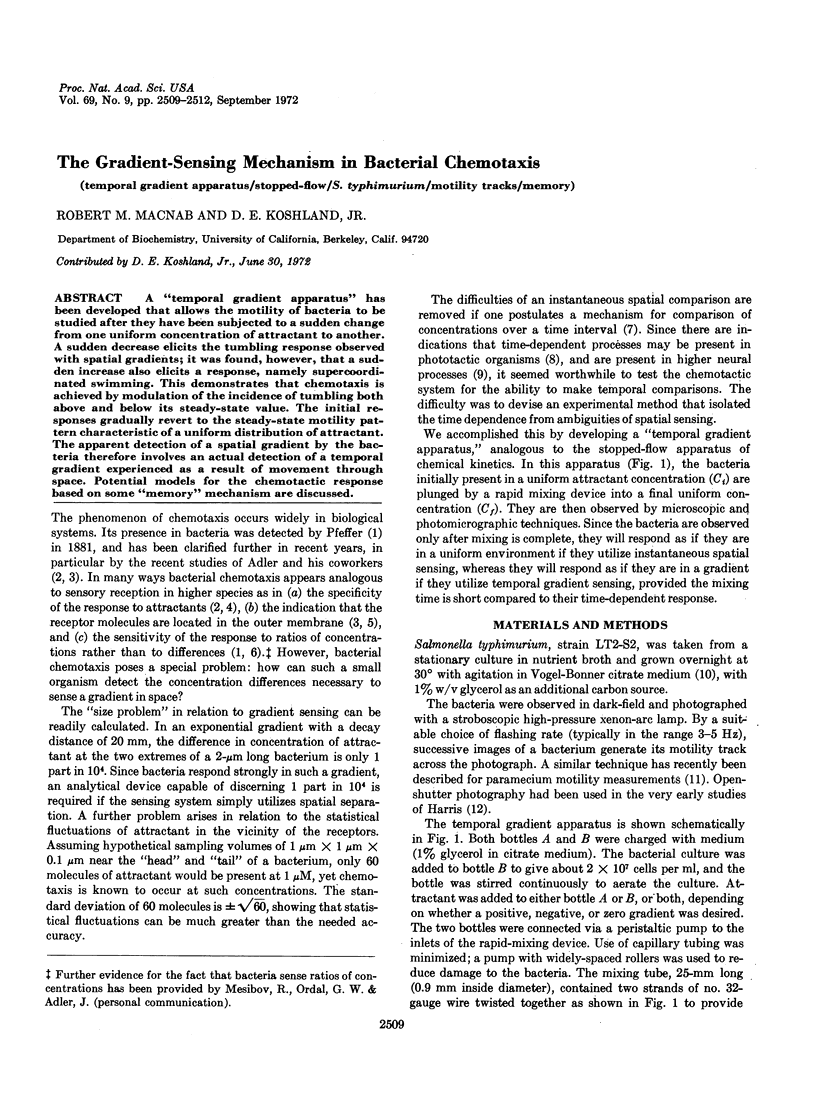
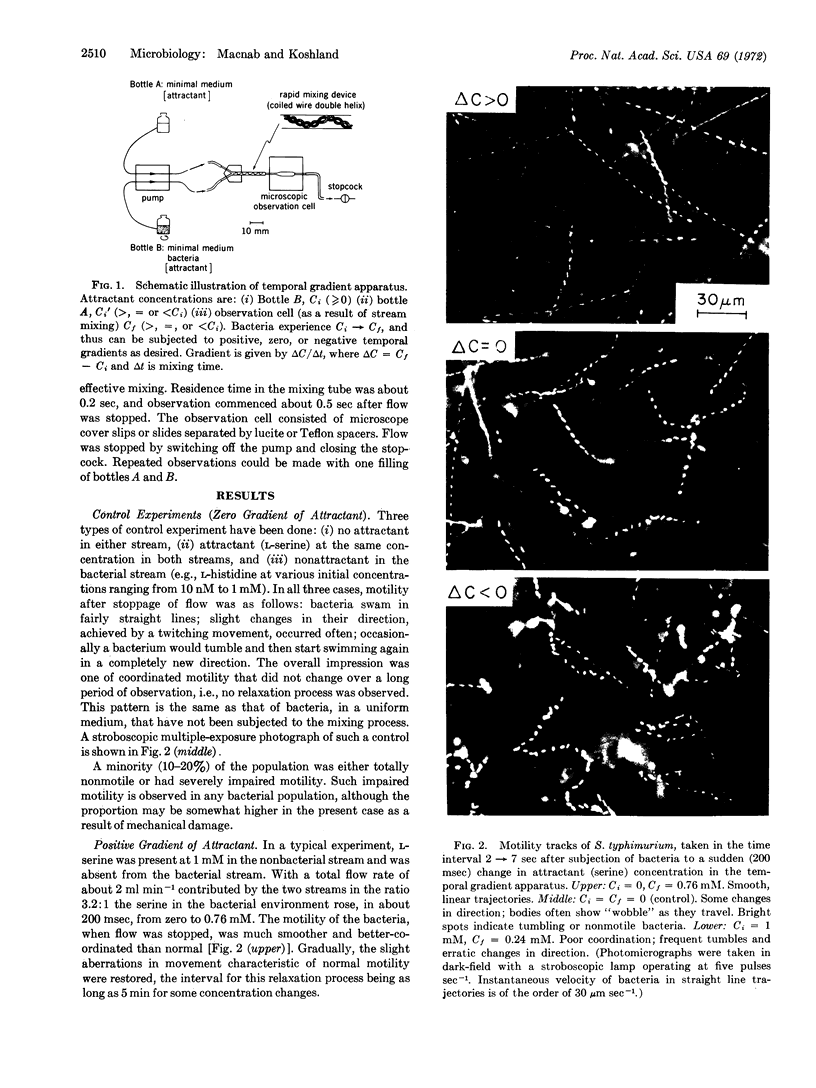
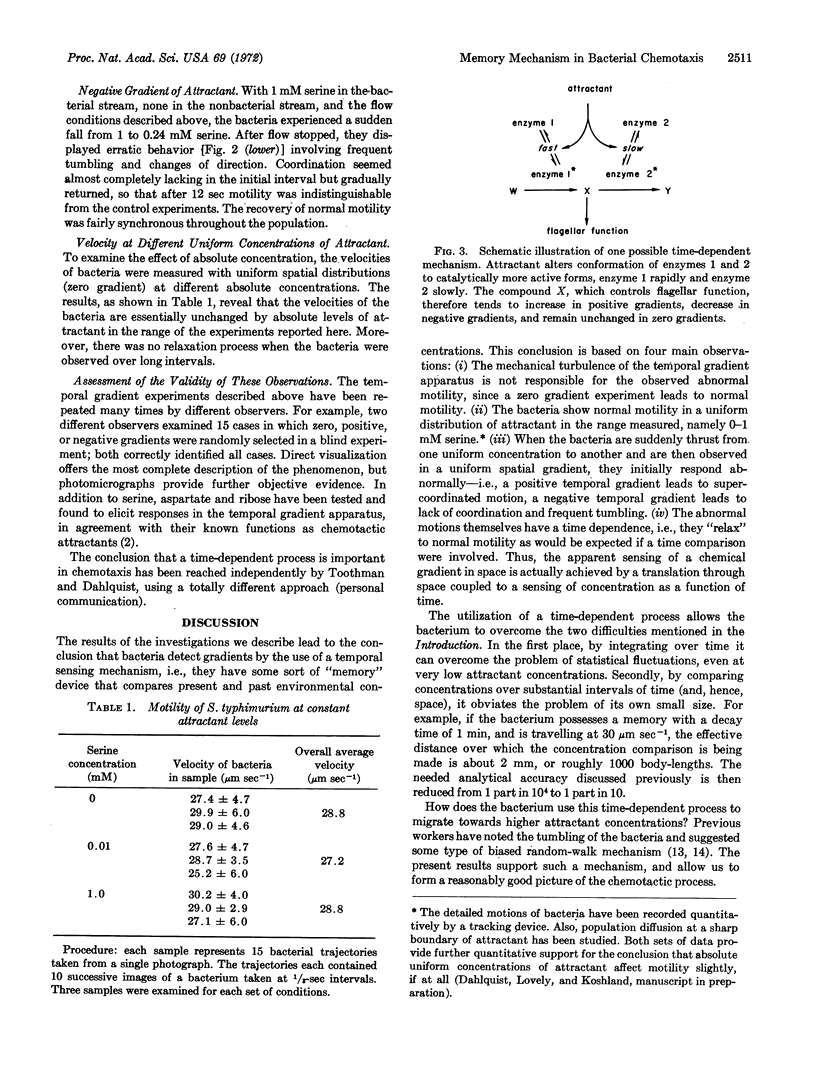
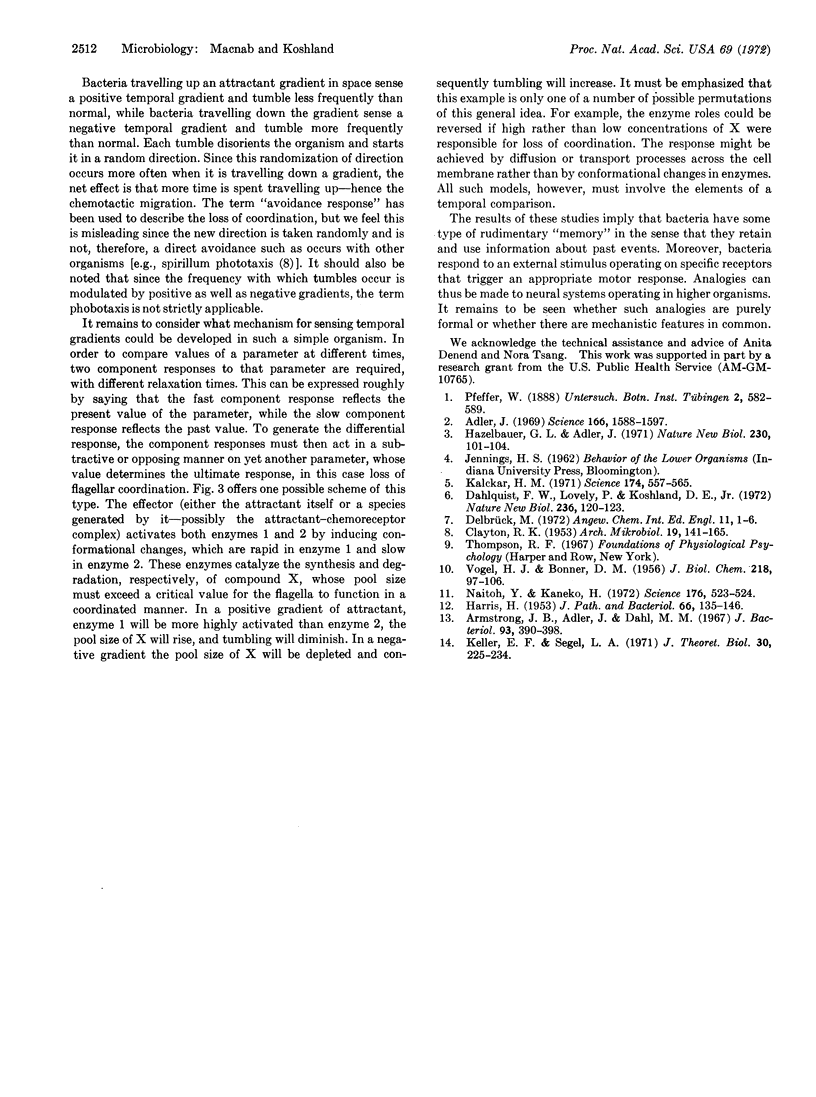
Images in this article
Selected References
These references are in PubMed. This may not be the complete list of references from this article.
- Adler J. Chemoreceptors in bacteria. Science. 1969 Dec 26;166(3913):1588–1597. doi: 10.1126/science.166.3913.1588. [DOI] [PubMed] [Google Scholar]
- Armstrong J. B., Adler J., Dahl M. M. Nonchemotactic mutants of Escherichia coli. J Bacteriol. 1967 Jan;93(1):390–398. doi: 10.1128/jb.93.1.390-398.1967. [DOI] [PMC free article] [PubMed] [Google Scholar]
- CLAYTON R. K. Studies in the phototaxis of Rhodospirillum rubrum. III. Quantitative relations between stimulus and response. Arch Mikrobiol. 1953;19(2):141–165. doi: 10.1007/BF00446397. [DOI] [PubMed] [Google Scholar]
- Dahlquist F. W., Lovely P., Koshland D. E., Jr Quantitative analysis of bacterial migration in chemotaxis. Nat New Biol. 1972 Mar 29;236(65):120–123. doi: 10.1038/newbio236120a0. [DOI] [PubMed] [Google Scholar]
- Delbrück M. Signal transducers: terra incognita of molecular biology. Angew Chem Int Ed Engl. 1972 Jan;11(1):1–6. doi: 10.1002/anie.197200011. [DOI] [PubMed] [Google Scholar]
- HARRIS H. Chemotaxis of granulocytes. J Pathol Bacteriol. 1953 Jul;66(1):135–146. doi: 10.1002/path.1700660117. [DOI] [PubMed] [Google Scholar]
- Hazelbauer G. L., Adler J. Role of the galactose binding protein in chemotaxis of Escherichia coli toward galactose. Nat New Biol. 1971 Mar 24;230(12):101–104. doi: 10.1038/newbio230101a0. [DOI] [PubMed] [Google Scholar]
- Kalckar H. M. The periplasmic galactose binding protein of Escherichia coli. Science. 1971 Nov 5;174(4009):557–565. doi: 10.1126/science.174.4009.557. [DOI] [PubMed] [Google Scholar]
- Keller E. F., Segel L. A. Model for chemotaxis. J Theor Biol. 1971 Feb;30(2):225–234. doi: 10.1016/0022-5193(71)90050-6. [DOI] [PubMed] [Google Scholar]
- Naito Y., Kaneko H. Reactivated triton-extracted models o paramecium: modification of ciliary movement by calcium ions. Science. 1972 May 5;176(4034):523–524. doi: 10.1126/science.176.4034.523. [DOI] [PubMed] [Google Scholar]
- VOGEL H. J., BONNER D. M. Acetylornithinase of Escherichia coli: partial purification and some properties. J Biol Chem. 1956 Jan;218(1):97–106. [PubMed] [Google Scholar]