Abstract
It is demonstrated that proteins and nucleic acids can be transported through hydrophobic organic solvents (liquid membranes) via nonspecific complex formation with detergents, whereas no macromolecule transport is observed without the latter. A protein (or a nucleic acid) first interacts with an oppositely charged detergent due to hydrophobic ion pairing in the aqueous feed phase. The resultant hydrophobic complex readily partitions into an organic solvent and then into the aqueous receiver phase, where it dissociates. Experiments with (i) different detergent/protein molar ratios, (ii) a range of unrelated organic solvents as liquid membranes, and (iii) homologous detergents with hydrophobic tails of varying lengths indicate that the protein flux through the membrane directly correlates with the partitioning of the protein-detergent complexes from the aqueous feed into the organic phase. Very little protein transport was detected at detergent concentrations above the critical micelle concentration, suggesting that individual detergent molecules, rather than micelles, play the key role. The rate of the detergent-enabled protein transport is not a function of the protein molecular weight, provided that enough detergent molecules bind to make the complex sufficiently hydrophobic; e.g., bovine serum albumin can be transported faster than insulin, which is less than 1/10th of its size.
Full text
PDF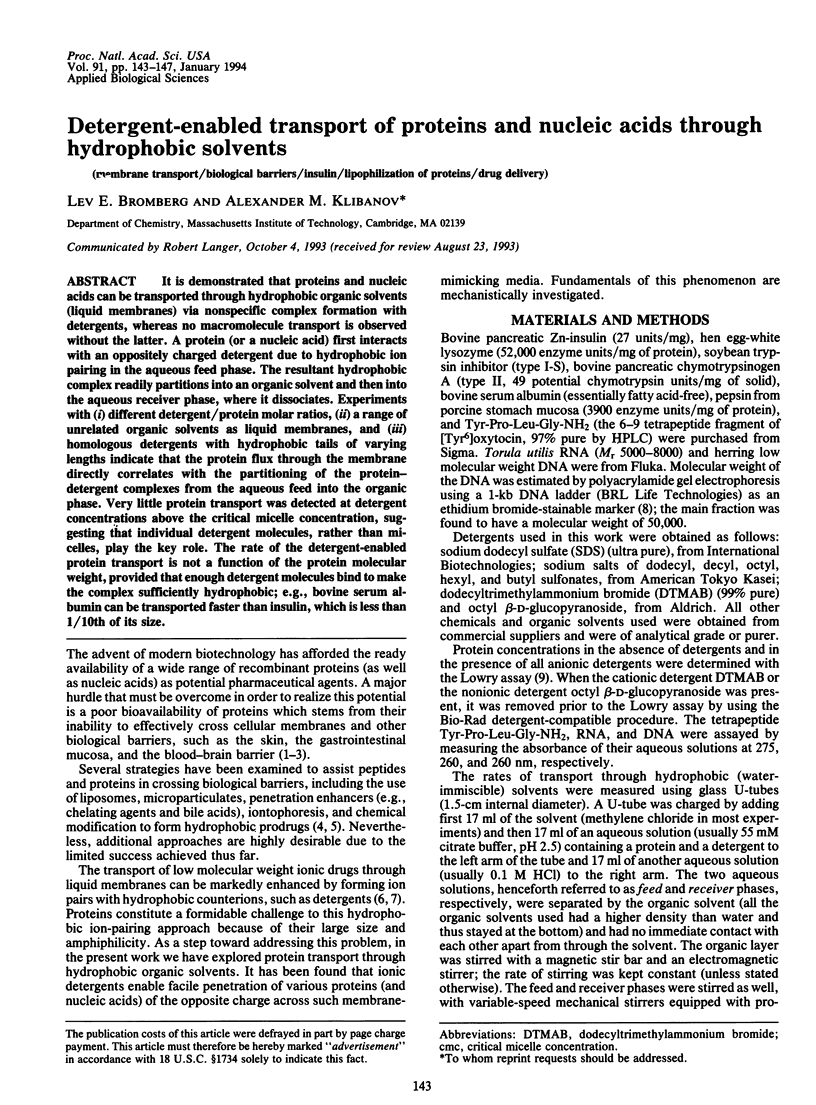
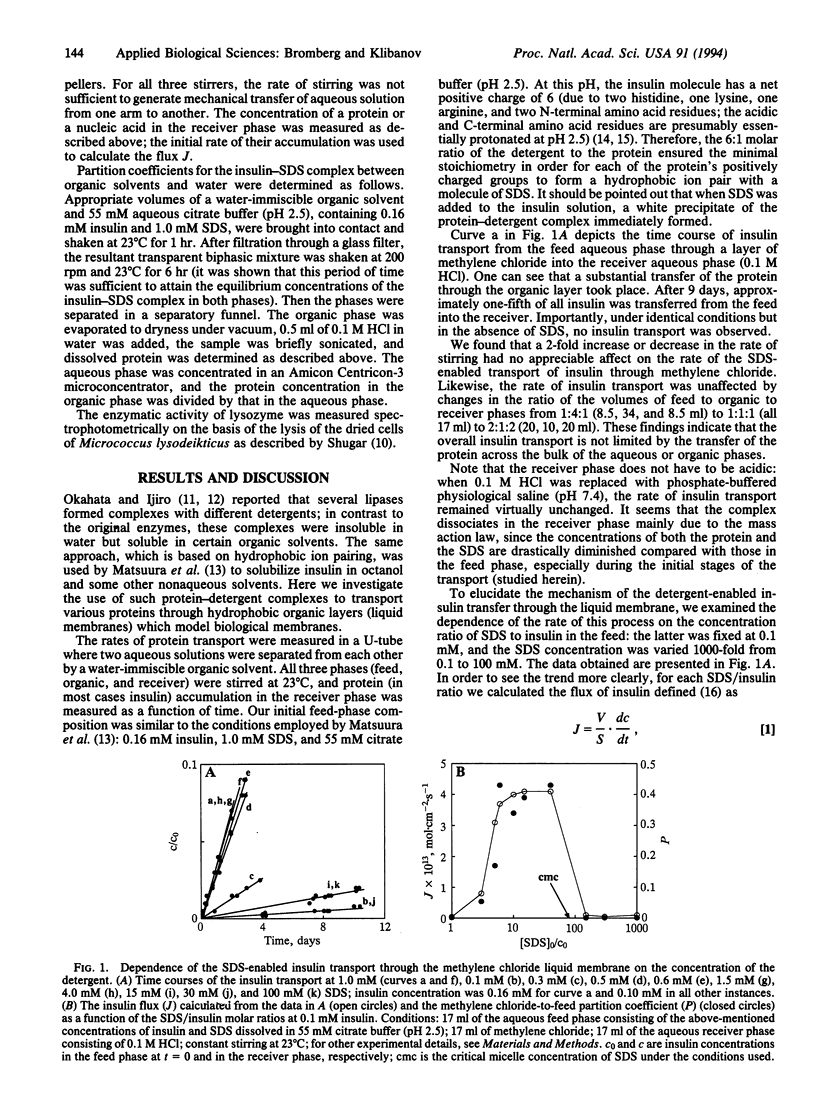
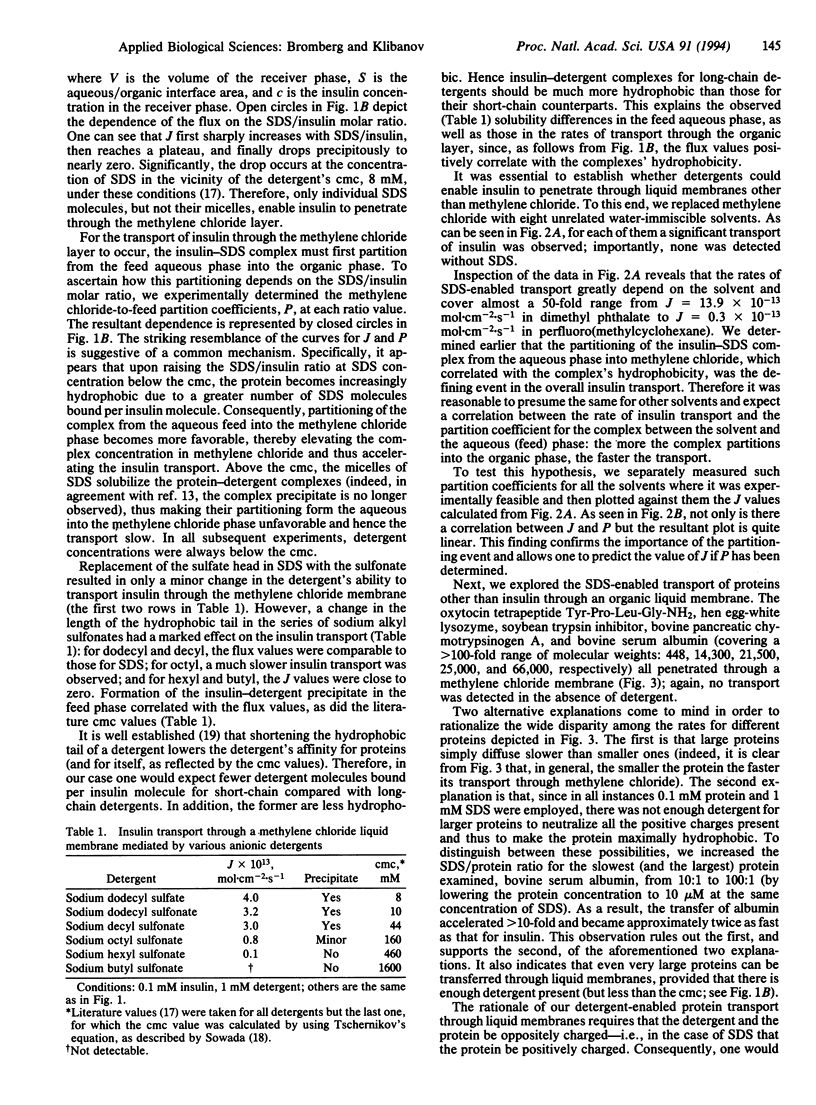
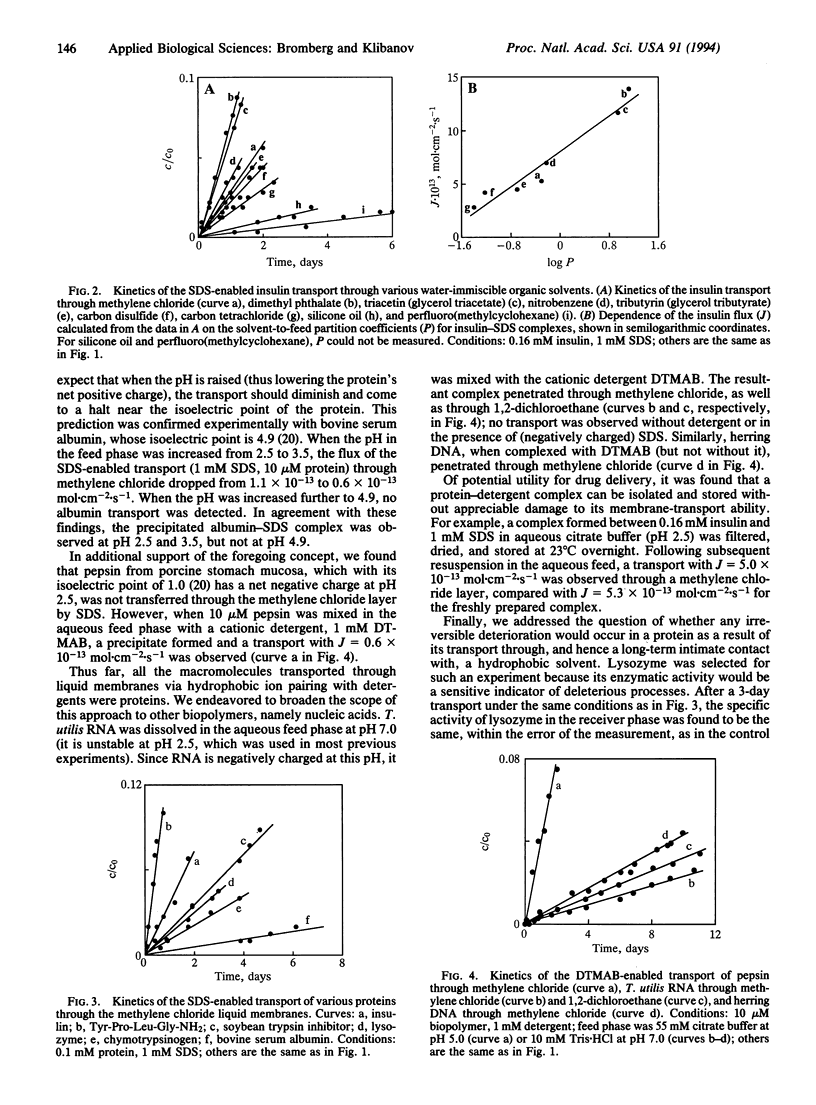
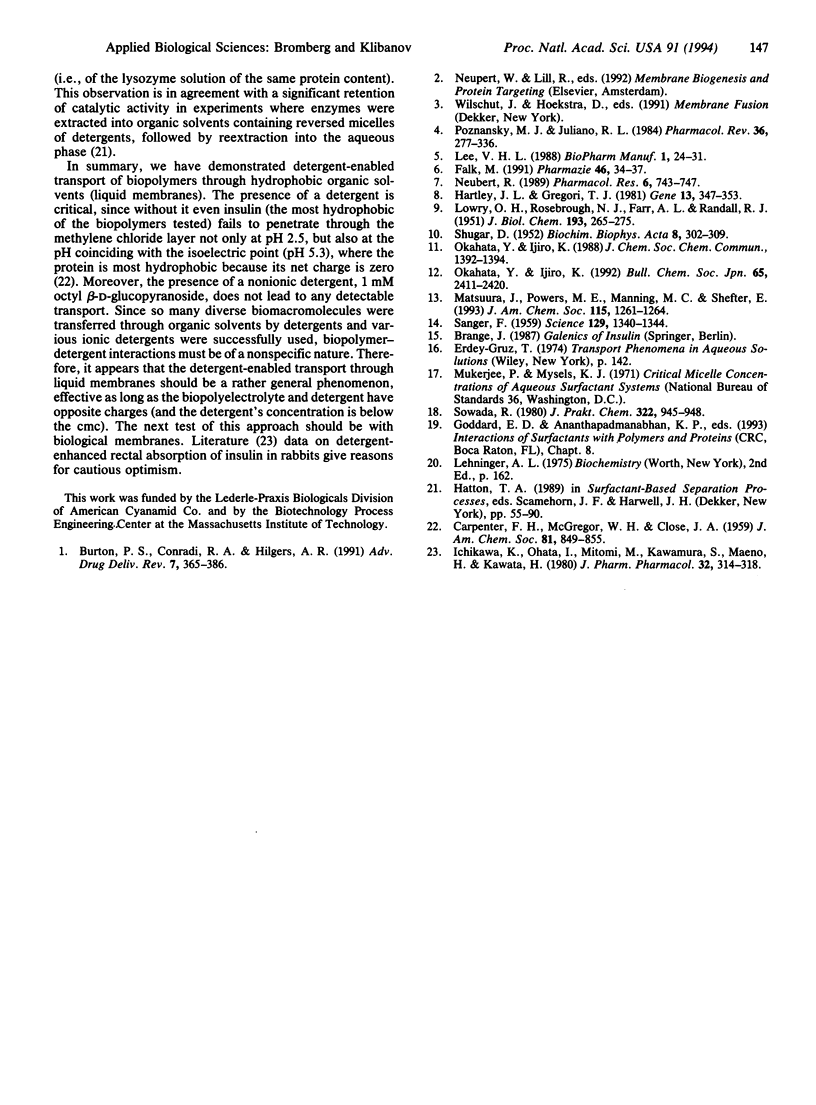
Selected References
These references are in PubMed. This may not be the complete list of references from this article.
- Hartley J. L., Gregori T. J. Cloning multiple copies of a DNA segment. Gene. 1981 May;13(4):347–353. doi: 10.1016/0378-1119(81)90014-7. [DOI] [PubMed] [Google Scholar]
- Ichikawa K., Ohata I., Mitomi M., Kawamura S., Maeno H., Kawata H. Rectal absorption of insulin suppositories in rabbits. J Pharm Pharmacol. 1980 May;32(5):314–318. doi: 10.1111/j.2042-7158.1980.tb12927.x. [DOI] [PubMed] [Google Scholar]
- LOWRY O. H., ROSEBROUGH N. J., FARR A. L., RANDALL R. J. Protein measurement with the Folin phenol reagent. J Biol Chem. 1951 Nov;193(1):265–275. [PubMed] [Google Scholar]
- Lawler J. M., Powers S. K., Hammeren J., Martin A. D. Oxygen cost of treadmill running in 24-month-old Fischer-344 rats. Med Sci Sports Exerc. 1993 Nov;25(11):1259–1264. [PubMed] [Google Scholar]
- Neubert R. Ion pair transport across membranes. Pharm Res. 1989 Sep;6(9):743–747. doi: 10.1023/a:1015963128124. [DOI] [PubMed] [Google Scholar]
- Poznansky M. J., Juliano R. L. Biological approaches to the controlled delivery of drugs: a critical review. Pharmacol Rev. 1984 Dec;36(4):277–336. [PubMed] [Google Scholar]
- SANGER F. Chemistry of insulin; determination of the structure of insulin opens the way to greater understanding of life processes. Science. 1959 May 15;129(3359):1340–1344. doi: 10.1126/science.129.3359.1340. [DOI] [PubMed] [Google Scholar]
- SHUGAR D. The measurement of lysozyme activity and the ultra-violet inactivation of lysozyme. Biochim Biophys Acta. 1952 Mar;8(3):302–309. doi: 10.1016/0006-3002(52)90045-0. [DOI] [PubMed] [Google Scholar]