Abstract
Several mutants of Escherichia coli affecting aerobic energy generation and energization of the bacterial membrane have been examined for their effect on streptomycin and gentamicin accumulation and susceptibility. A heme-deficient mutant (K207) and two mutants (CJ-8 [colicin K insensitive] and NR-70) associated with defective aerobic active transport were associated with decreased transport of streptomycin and gentamicin and increased resistance to those antibiotics. These mutants also exhibited increased resistance to several other aminoglycoside antibiotics, but not the aminocyclitol spectinomycin. The same observations were made with a ubiquinone-deficient mutant, but a strA derivative of this mutant was shown additionally to be saturable for streptomycin accumulation at a concentration four or more times lower than that required for saturation of the parent. A mutant uncoupled for adenosine 5′-triphosphate synthesis from electron transport and membrane Mg-adenosine 5′-triphosphatase deficient was hypersensitive to those aminoglycosides tested and spectinomycin, and showed enhanced transport of streptomycin and gentamicin. A variety of compounds structurally related to streptomycin were examined at high concentrations for inhibition of streptomycin uptake in a strA mutant of E. coli K-12 SA 1306, but no evidence for competition was detected, suggesting the absence of a common transport carrier. Four different divalent cations were shown to inhibit streptomycin and gentamicin accumulation in E. coli K-12 SA 1306. Divalent cations were shown to inhibit uptake of these two drugs in two bacterial species with distinct cell wall structures, Pseudomonas aeruginosa and Staphylococcus aureus, and to inhibit streptomycin uptake in spheroplasts of streptomycin-susceptible and -resistant E. coli. However, calcium had almost no inhibitory effect on streptomycin uptake by the ubiquinone-deficient mutant E. coli AN66. These and previous findings have been used to formulate a model for aminoglycoside entry into bacteria using a low-affinity membranous complex involved in membrane energization that includes respiratory quinones, which probably act to bind and transport aminoglycosides across the cell membrane. This phase of transport is associated with the lowest accumulation rate (termed energy-dependent phase I) that is rate limiting for susceptibility. It is further proposed that subsequent association of the membrane-bound aminoglycoside with higher-affinity binding sites on membrane-associated ribosomes carrying out a normal ribosomal cycle and protein synthesis results in a more rapid transport rate (termed energy-dependent phase II). The increased rate could result from a state of membrane energization analogous to that causing enhanced aminoglycoside transport rates seen in the uncoupled mutant, AN120. How this model explains the mechanism by which enzymatically modified aminoglycosides render cells resistant to unmodified aminoglycosides is also discussed.
Full text
PDF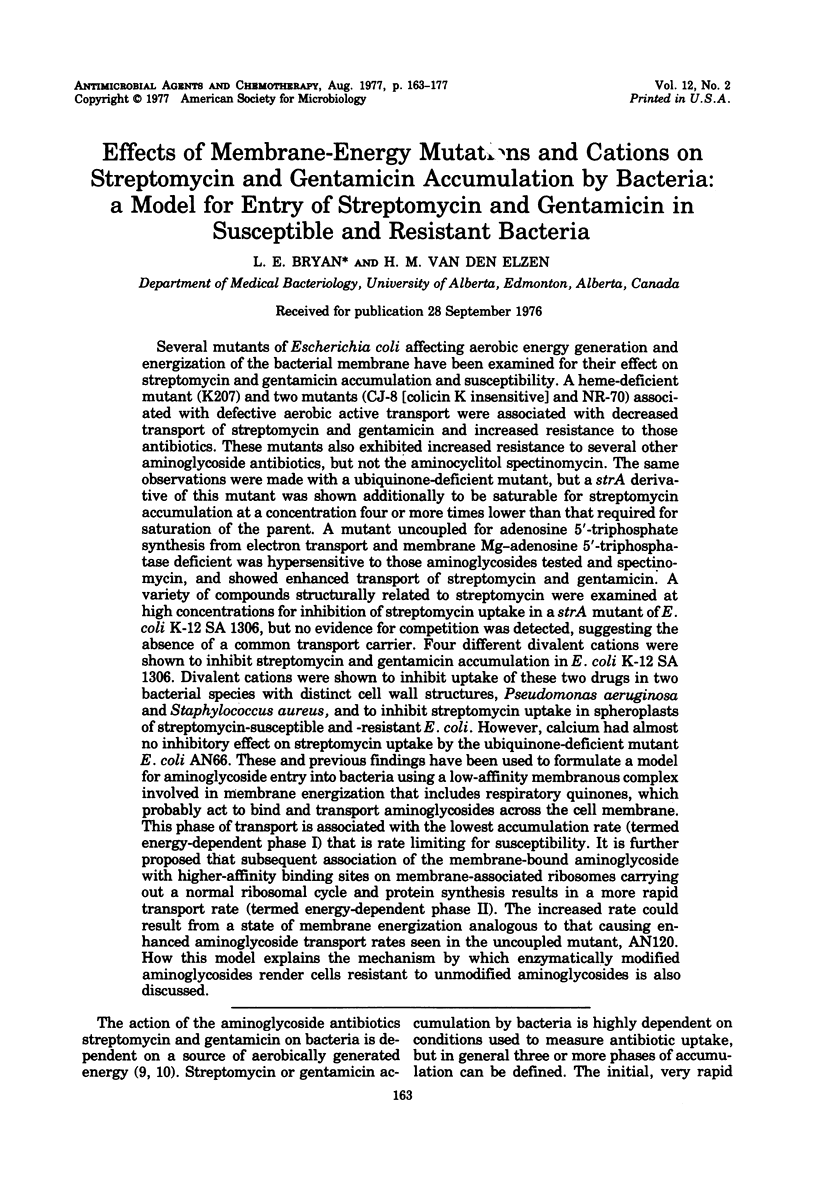
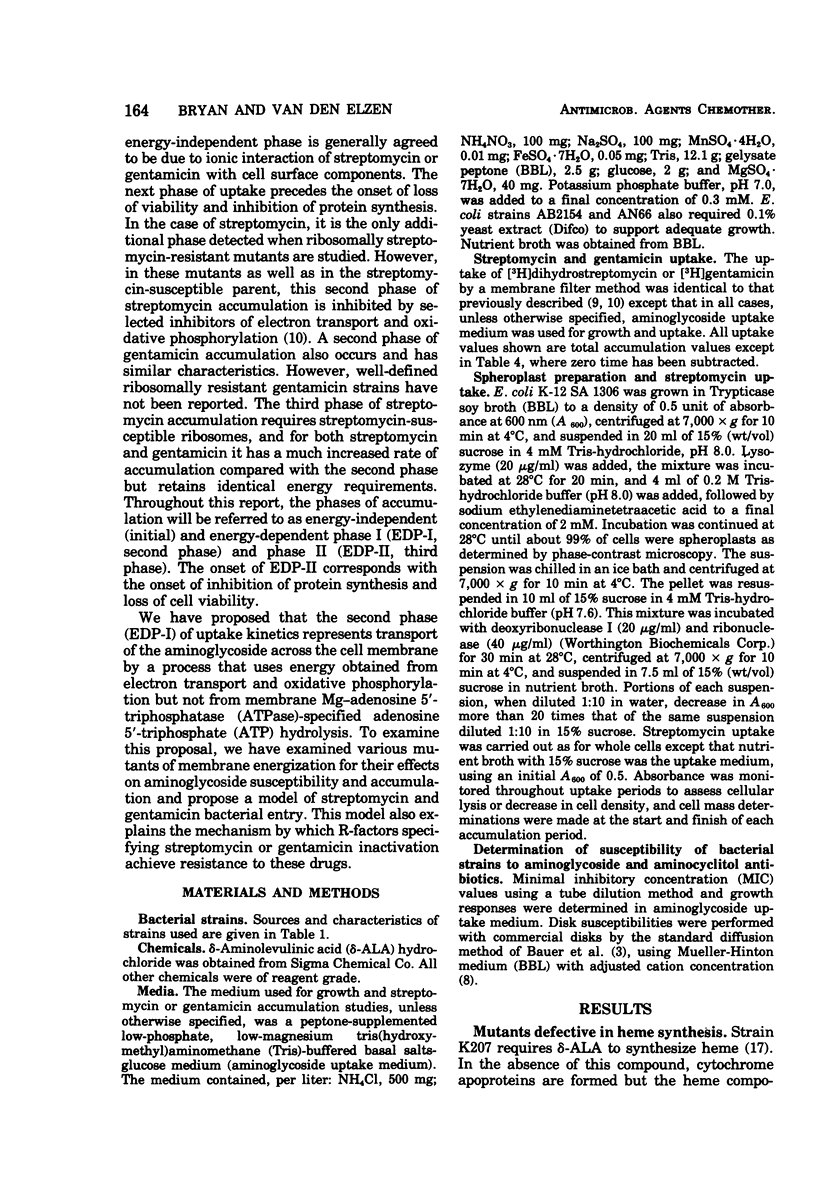
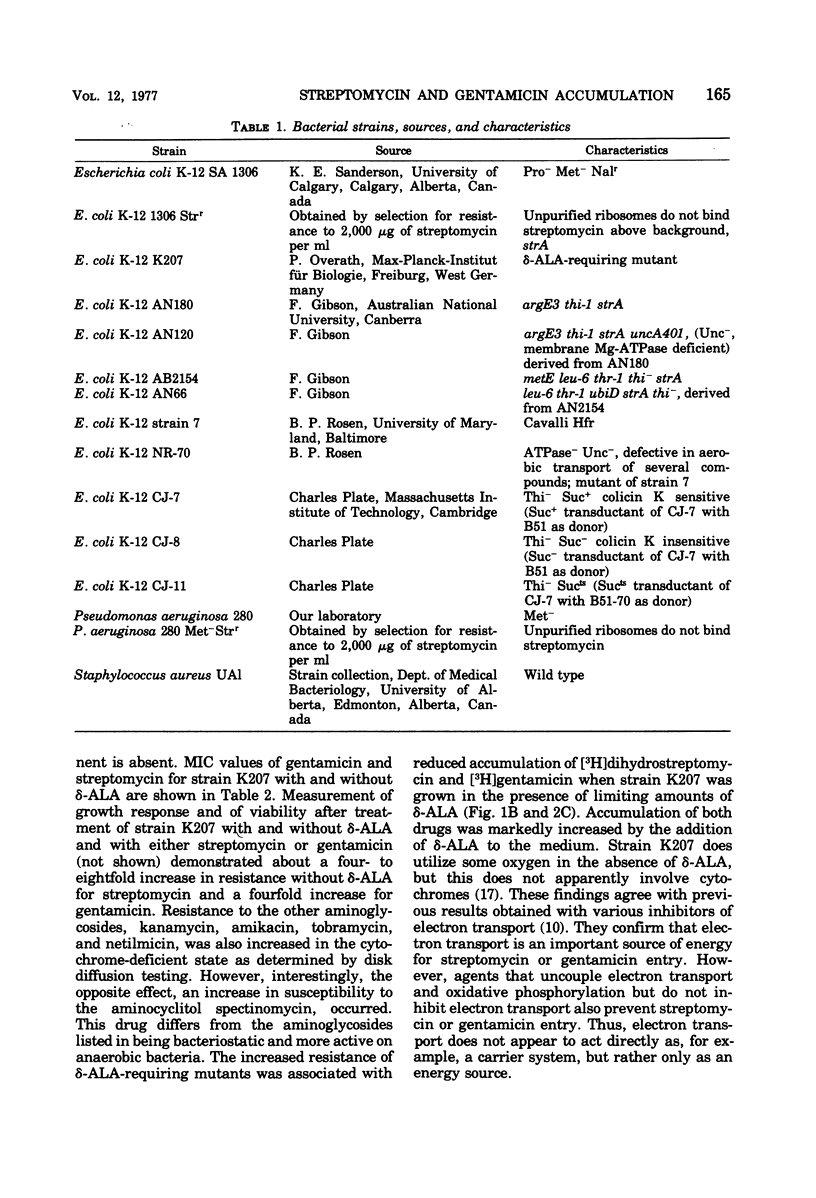
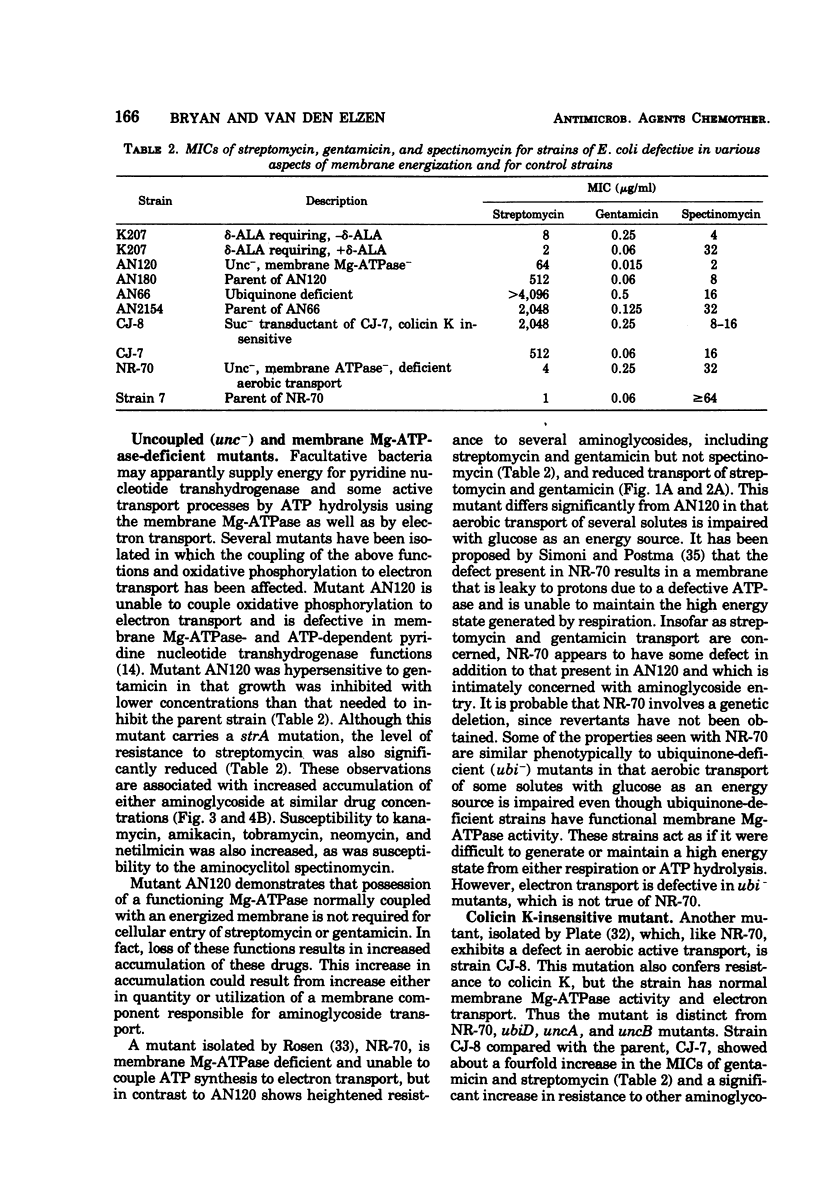
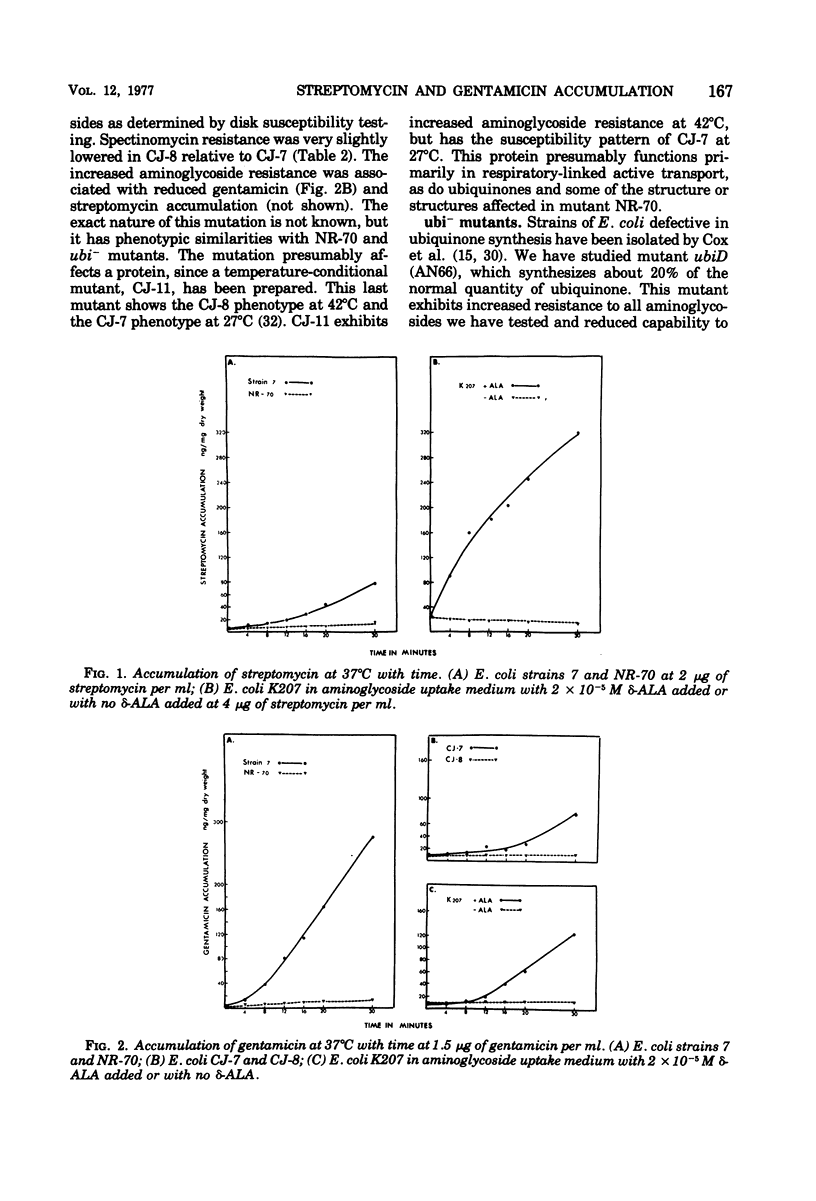
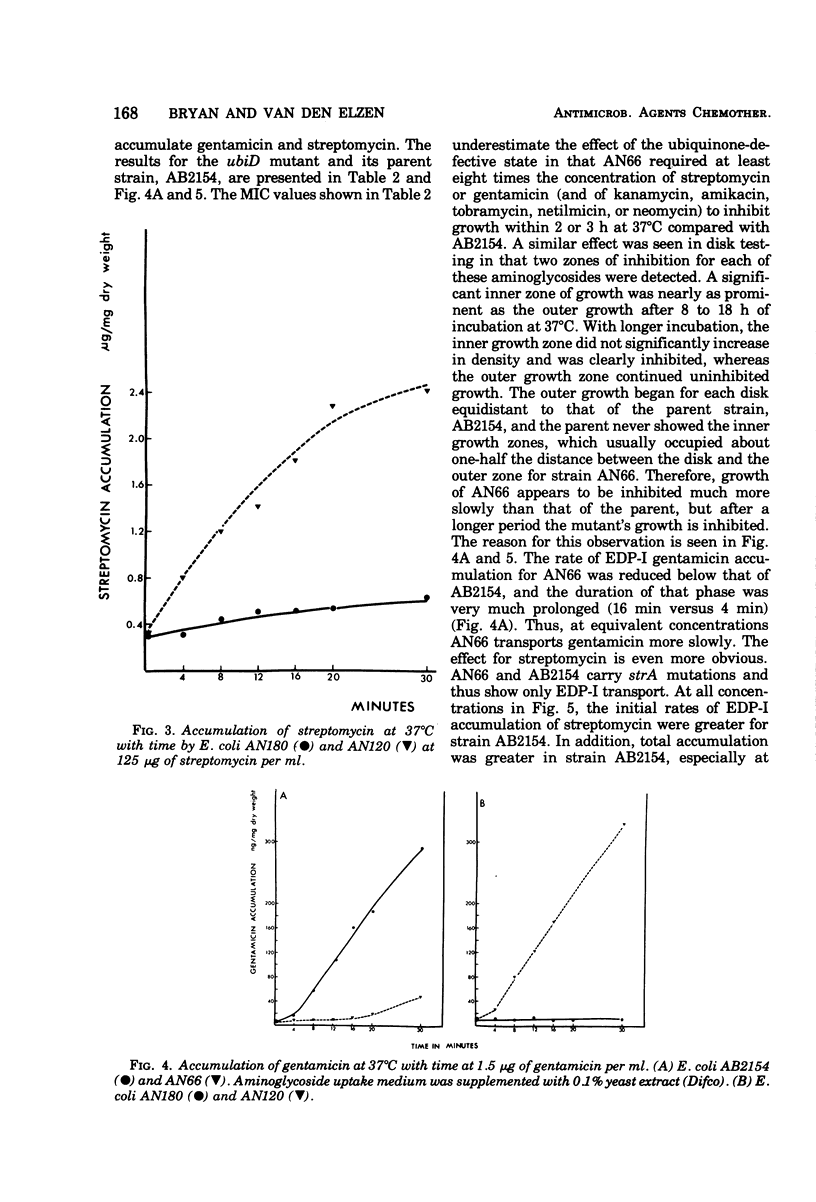
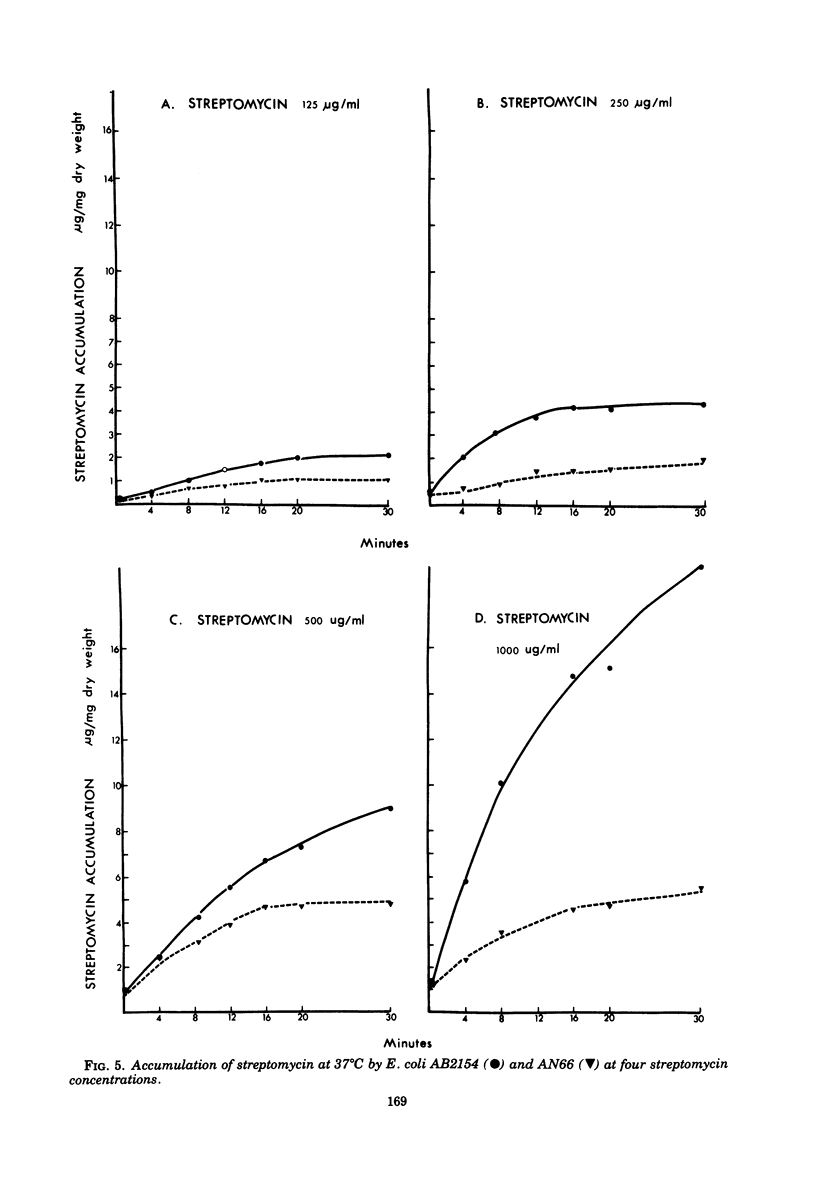
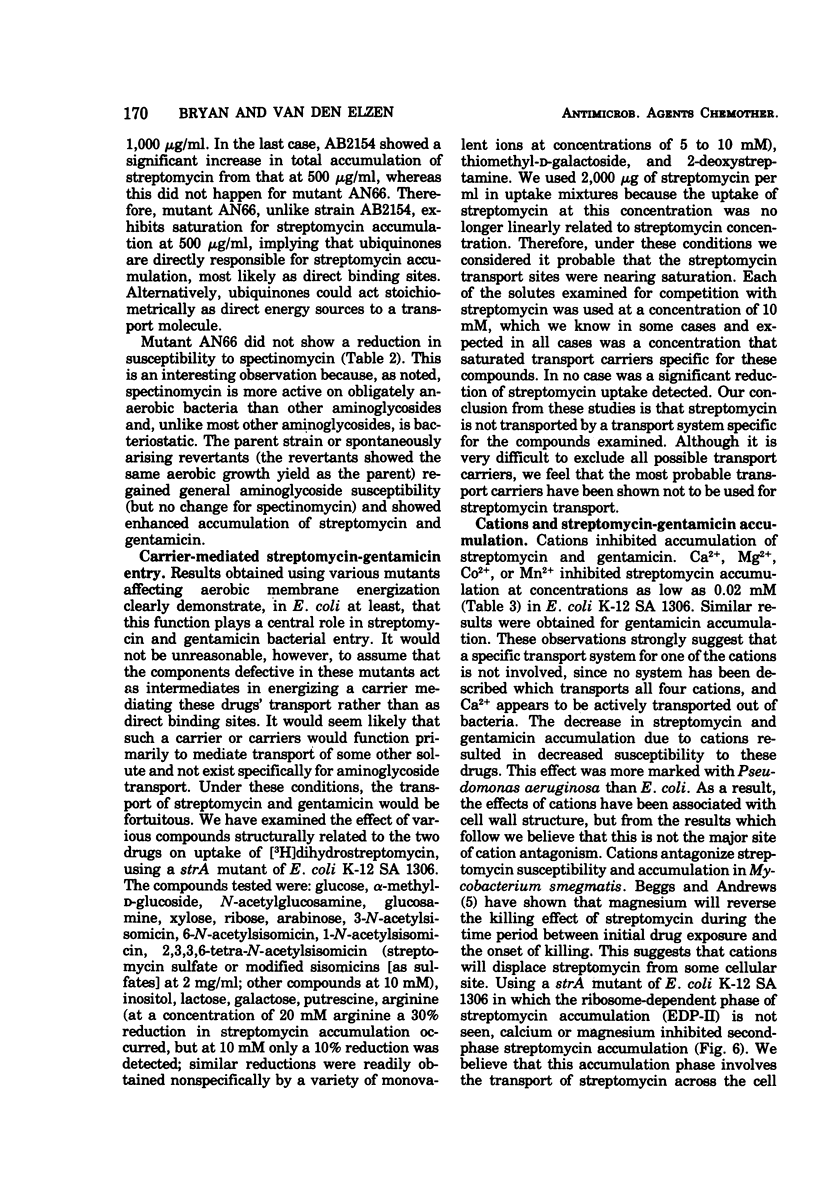
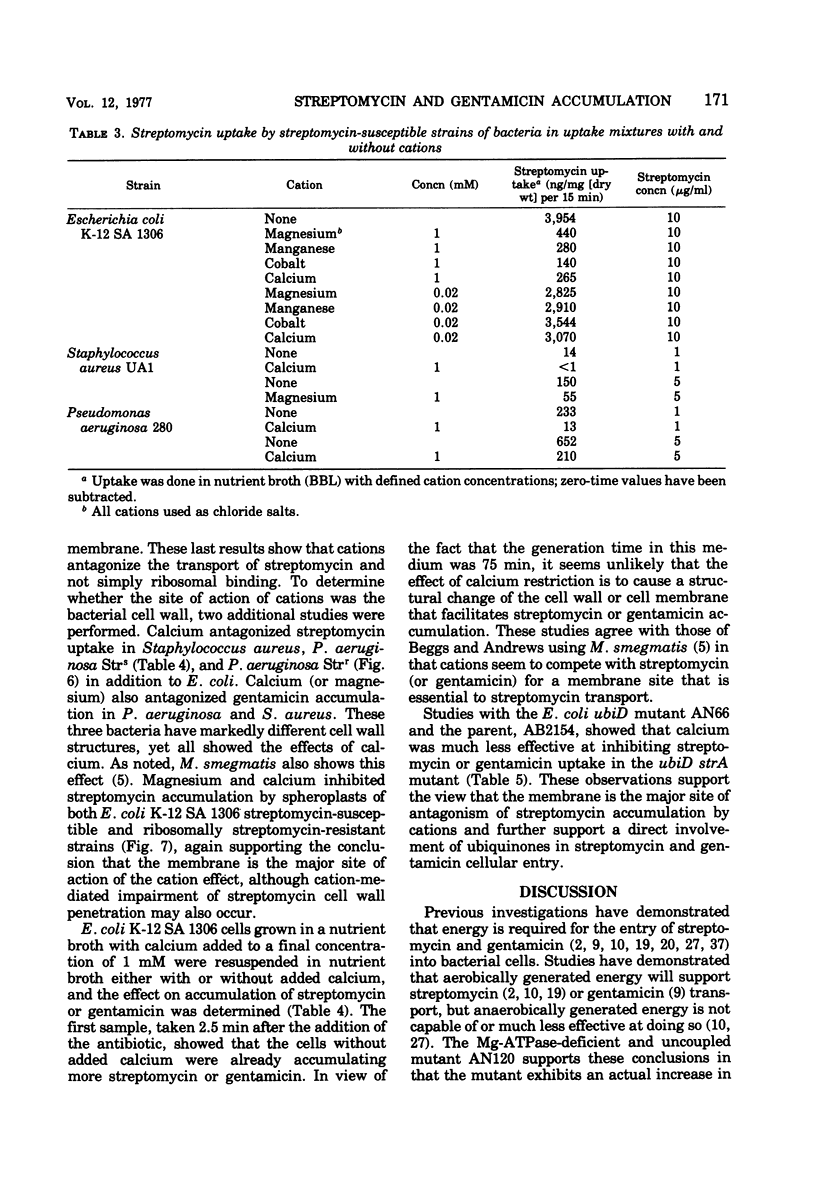
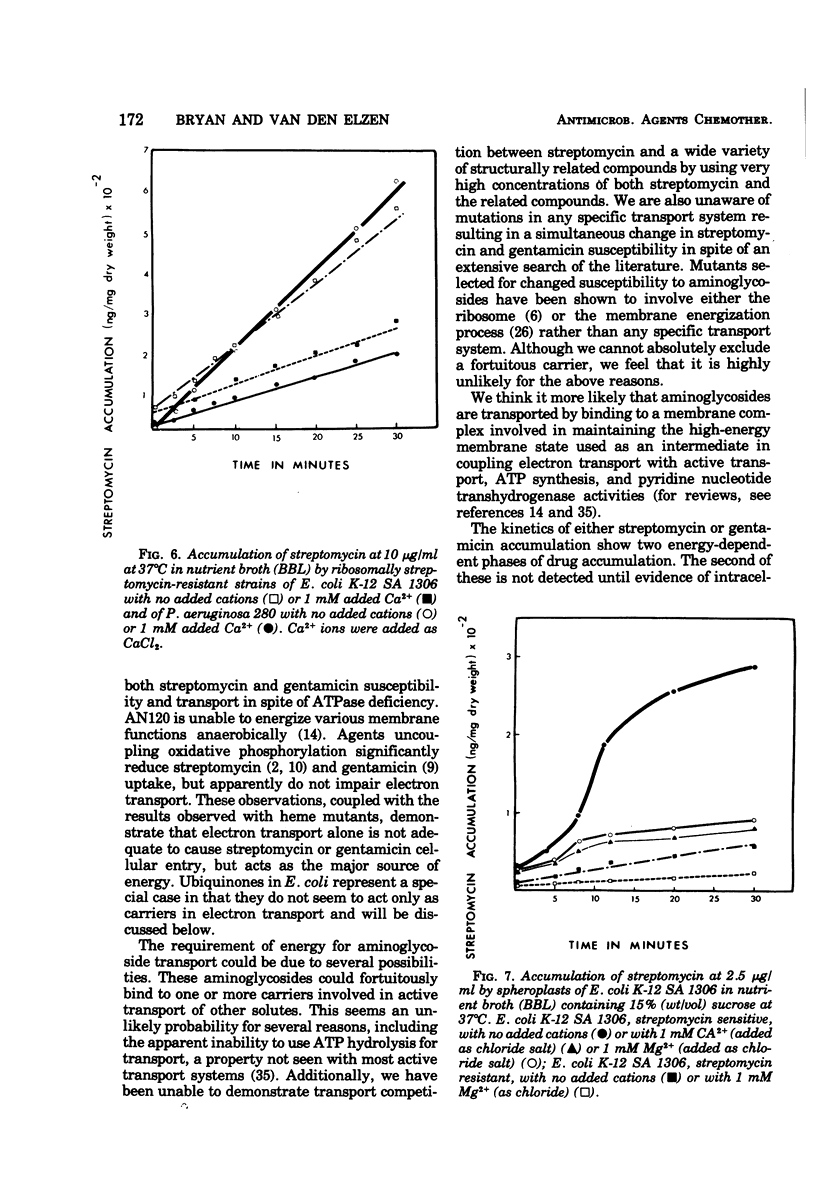
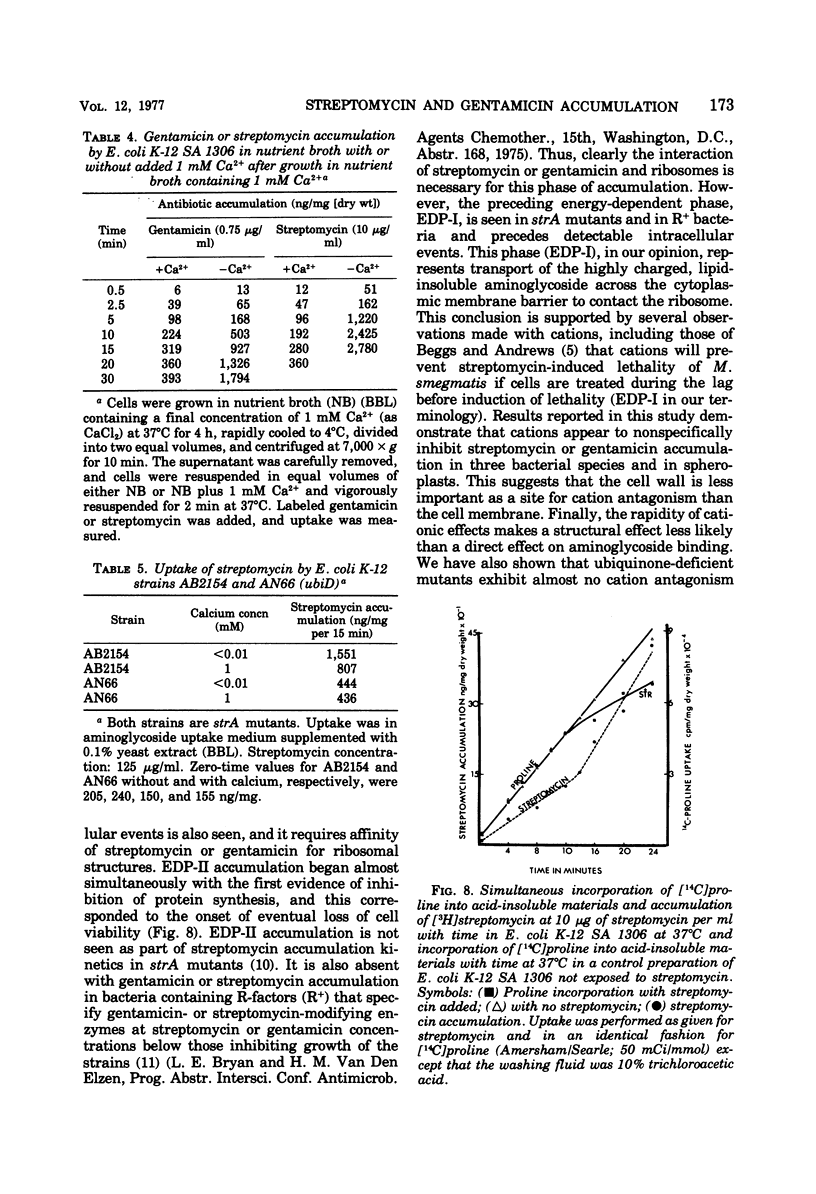
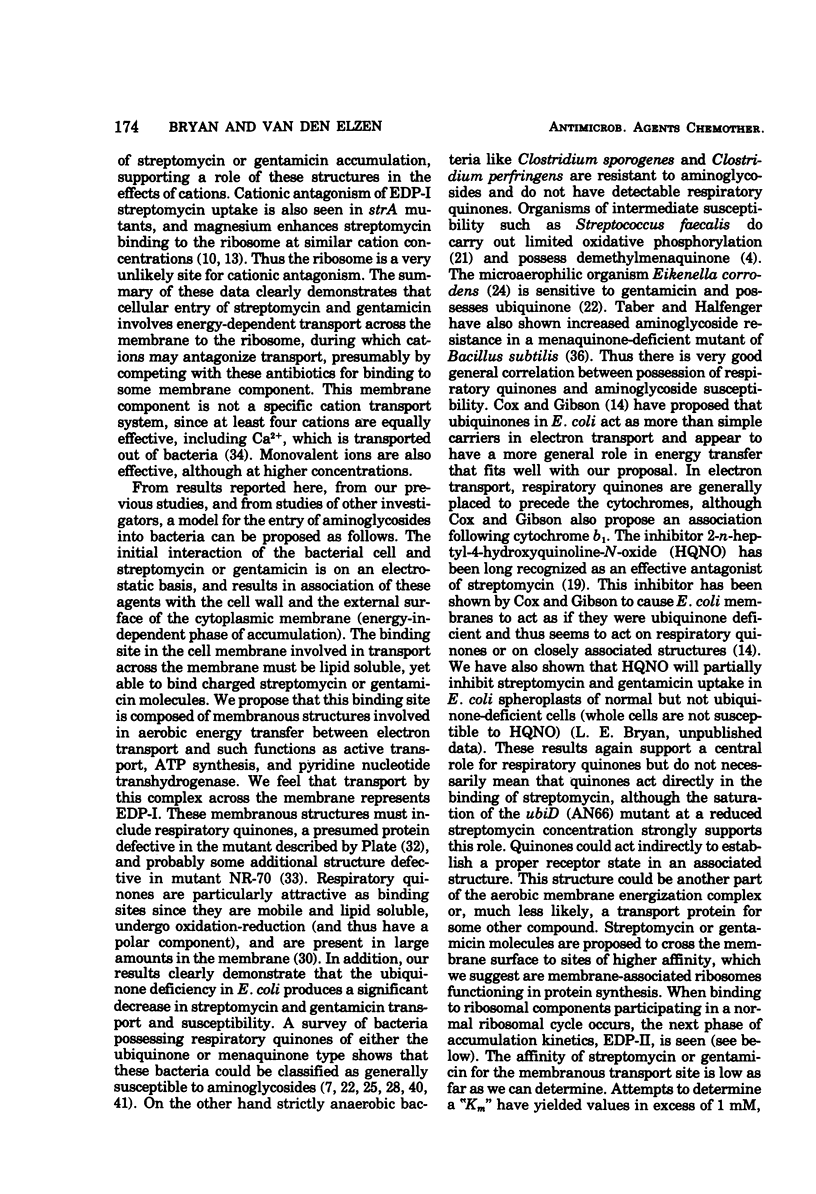
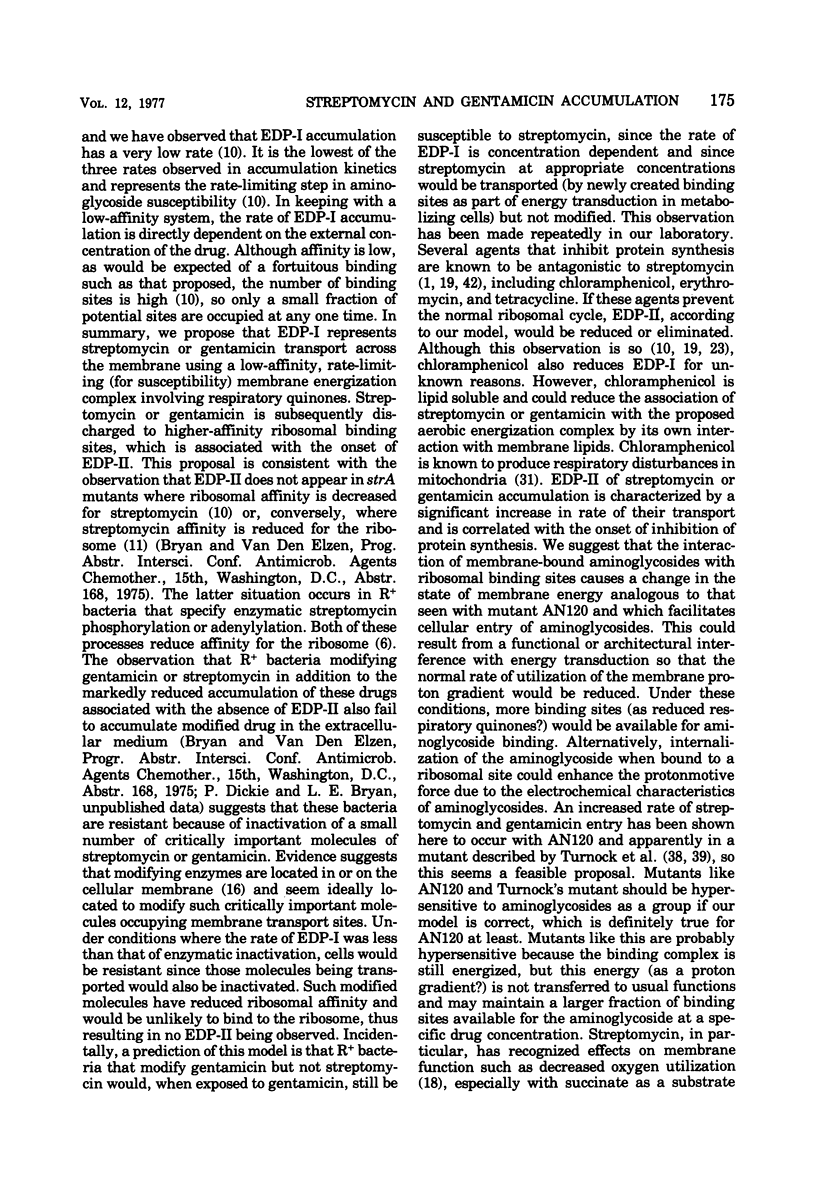
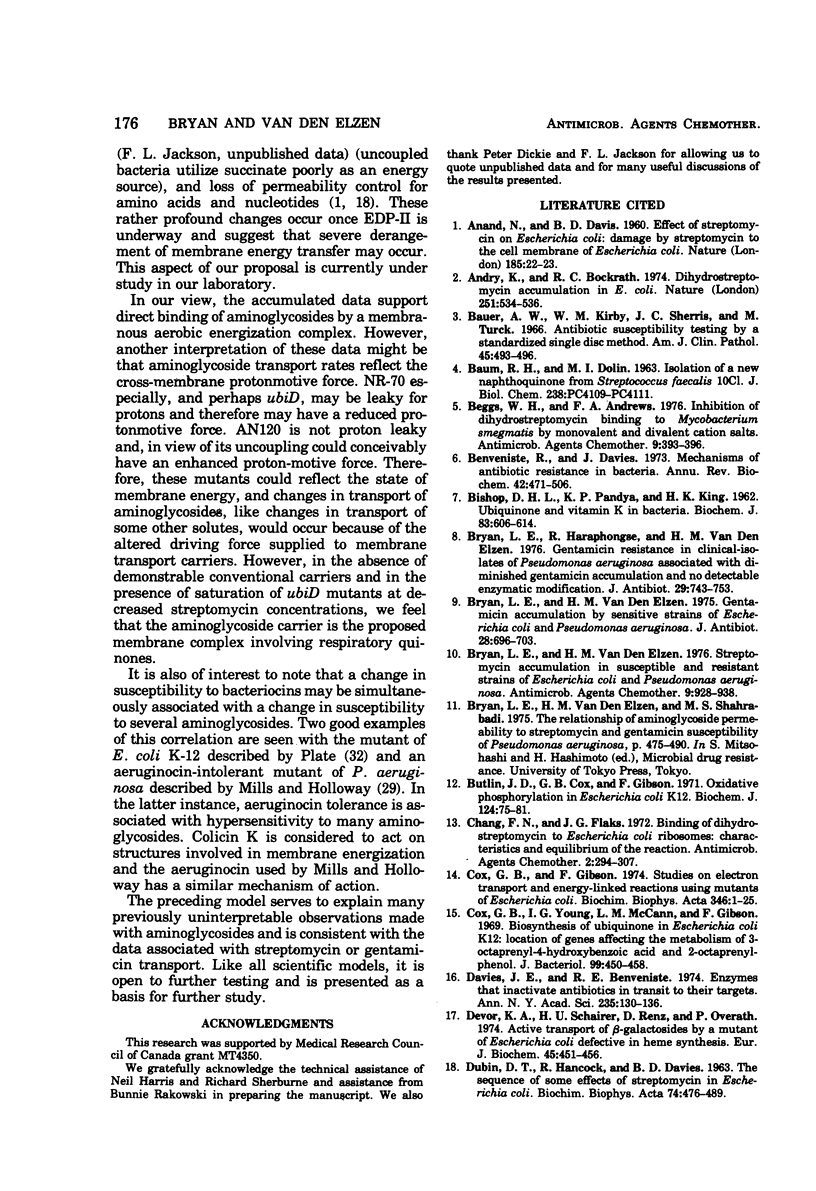
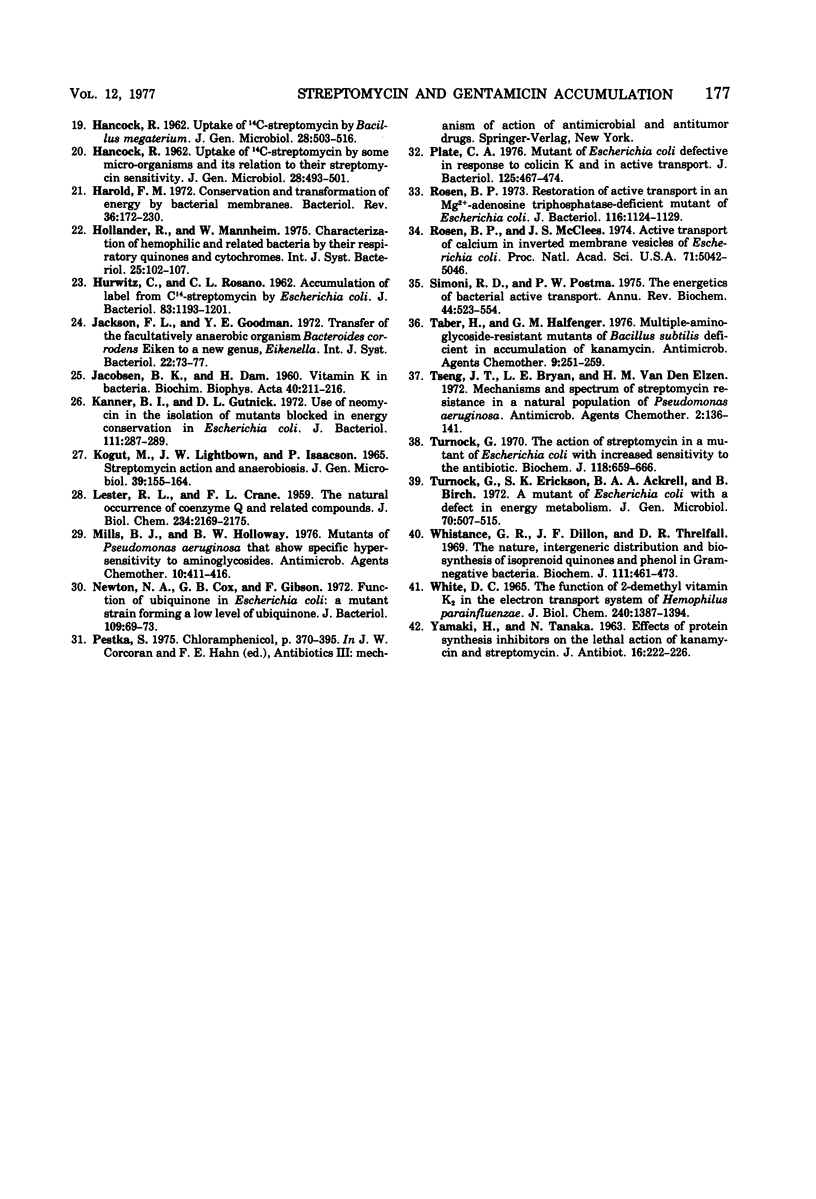
Selected References
These references are in PubMed. This may not be the complete list of references from this article.
- ANAND N., DAVIS B. D. Damage by streptomycin to the cell membrane of Escherichia coli. Nature. 1960 Jan 2;185:22–23. doi: 10.1038/185022a0. [DOI] [PubMed] [Google Scholar]
- Andry K., Bockrath R. C. Dihydrostreptomycin accumulation in E. coli. Nature. 1974 Oct 11;251(5475):534–536. doi: 10.1038/251534a0. [DOI] [PubMed] [Google Scholar]
- BISHOP D. H., PANDYA K. P., KING H. K. Ubiquinone and vitamin K in bacteria. Biochem J. 1962 Jun;83:606–614. doi: 10.1042/bj0830606. [DOI] [PMC free article] [PubMed] [Google Scholar]
- Bauer A. W., Kirby W. M., Sherris J. C., Turck M. Antibiotic susceptibility testing by a standardized single disk method. Am J Clin Pathol. 1966 Apr;45(4):493–496. [PubMed] [Google Scholar]
- Beggs W. H., Andrews F. A. Inhibition of dihydrostreptomycin binding to Mycobacterium smegmatis by monovalent and divalent cation salts. Antimicrob Agents Chemother. 1976 Mar;9(3):393–396. doi: 10.1128/aac.9.3.393. [DOI] [PMC free article] [PubMed] [Google Scholar]
- Benveniste R., Davies J. Mechanisms of antibiotic resistance in bacteria. Annu Rev Biochem. 1973;42:471–506. doi: 10.1146/annurev.bi.42.070173.002351. [DOI] [PubMed] [Google Scholar]
- Bryan L. E., Haraphongse R., Van den Elzen H. M. Gentamicin resistance in clinical-isolates of Pseudomonas aeruginosa associated with diminished gentamicin accumulation and no detectable enzymatic modification. J Antibiot (Tokyo) 1976 Jul;29(7):743–753. doi: 10.7164/antibiotics.29.743. [DOI] [PubMed] [Google Scholar]
- Bryan L. E., Van Den Elzen H. M. Gentamicin accumulation by sensitive strains of Escherichia coli and Pseudomonas aeruginosa. J Antibiot (Tokyo) 1975 Sep;28(9):696–703. doi: 10.7164/antibiotics.28.696. [DOI] [PubMed] [Google Scholar]
- Bryan L. E., Van den Elzen H. M. Streptomycin accumulation in susceptible and resistant strains of Escherichia coli and Pseudomonas aeruginosa. Antimicrob Agents Chemother. 1976 Jun;9(6):928–938. doi: 10.1128/aac.9.6.928. [DOI] [PMC free article] [PubMed] [Google Scholar]
- Butlin J. D., Cox G. B., Gibson F. Oxidative phosphorylation in Escherichia coli K12. Mutations affecting magnesium ion- or calcium ion-stimulated adenosine triphosphatase. Biochem J. 1971 Aug;124(1):75–81. doi: 10.1042/bj1240075. [DOI] [PMC free article] [PubMed] [Google Scholar]
- Chang F. N., Flaks J. G. Binding of dihydrostreptomycin to Escherichia coli ribosomes: characteristics and equilibrium of the reaction. Antimicrob Agents Chemother. 1972 Oct;2(4):294–307. doi: 10.1128/aac.2.4.294. [DOI] [PMC free article] [PubMed] [Google Scholar]
- Cox G. B., Gibson F. Studies on electron transport and energy-linked reactions using mutants of Escherichia coli. Biochim Biophys Acta. 1974 Apr 30;346(1):1–25. doi: 10.1016/0304-4173(74)90010-x. [DOI] [PubMed] [Google Scholar]
- Cox G. B., Young I. G., McCann L. M., Gibson F. Biosynthesis of ubiquinone in Escherichia coli K-12: location of genes affecting the metabolism of 3-octaprenyl-4-hydroxybenzoic acid and 2-octaprenylphenol. J Bacteriol. 1969 Aug;99(2):450–458. doi: 10.1128/jb.99.2.450-458.1969. [DOI] [PMC free article] [PubMed] [Google Scholar]
- DUBIN D. T., HANCOCK R., DAVIS B. D. THE SEQUENCE OF SOME EFFECTS OF STREPTOMYCIN IN ESCHERICHIA COLI. Biochim Biophys Acta. 1963 Aug 13;74:476–489. doi: 10.1016/0006-3002(63)91390-8. [DOI] [PubMed] [Google Scholar]
- Davies J. E., Benveniste R. E. Enzymes that inactivate antibiotics in transit to their targets. Ann N Y Acad Sci. 1974 May 10;235(0):130–136. doi: 10.1111/j.1749-6632.1974.tb43262.x. [DOI] [PubMed] [Google Scholar]
- Devor K. A., Schairer H. U., Renz D., Overath P. Active transport of beta-galactosides by a mutant of Escherichia coli defective in heme synthesis. Eur J Biochem. 1974 Jun 15;45(2):451–456. doi: 10.1111/j.1432-1033.1974.tb03569.x. [DOI] [PubMed] [Google Scholar]
- HANCOCK R. Uptake of 14C-streptomycin by Bacillus megaterium. J Gen Microbiol. 1962 Jul;28:503–516. doi: 10.1099/00221287-28-3-503. [DOI] [PubMed] [Google Scholar]
- HANCOCK R. Uptake of 14C-streptomycin by some microorganisms and its relation to their streptomycin sensitivity. J Gen Microbiol. 1962 Jul;28:493–501. doi: 10.1099/00221287-28-3-493. [DOI] [PubMed] [Google Scholar]
- HURWITZ C., ROSANO C. L. Accumulation of label from C14-streptomycin by Escherichia coli. J Bacteriol. 1962 Jun;83:1193–1201. doi: 10.1128/jb.83.6.1193-1201.1962. [DOI] [PMC free article] [PubMed] [Google Scholar]
- Harold F. M. Conservation and transformation of energy by bacterial membranes. Bacteriol Rev. 1972 Jun;36(2):172–230. doi: 10.1128/br.36.2.172-230.1972. [DOI] [PMC free article] [PubMed] [Google Scholar]
- JACOBSEN B. K., DAM H. Vitamin K in bacteria. Biochim Biophys Acta. 1960 May 20;40:211–216. doi: 10.1016/0006-3002(60)91344-5. [DOI] [PubMed] [Google Scholar]
- KOGUT M., LIGHTBROWN J. W., ISAACSON P. STREPTOMYCIN ACTION AND ANAEROBIOSIS. J Gen Microbiol. 1965 May;39:155–164. doi: 10.1099/00221287-39-2-155. [DOI] [PubMed] [Google Scholar]
- Kanner B. I., Gutnick D. L. Use of neomycin in the isolation of mutants blocked in energy conservation in Escherichia coli. J Bacteriol. 1972 Jul;111(1):287–289. doi: 10.1128/jb.111.1.287-289.1972. [DOI] [PMC free article] [PubMed] [Google Scholar]
- LESTER R. L., CRANE F. L. The natural occurrence of coenzyme Q and related compounds. J Biol Chem. 1959 Aug;234(8):2169–2175. [PubMed] [Google Scholar]
- Mills B. J., Holloway B. W. Mutants of Pseudomonas aeruginosa that show specific hypersensitivity to aminoglycosides. Antimicrob Agents Chemother. 1976 Sep;10(3):411–416. doi: 10.1128/aac.10.3.411. [DOI] [PMC free article] [PubMed] [Google Scholar]
- Newton N. A., Cox G. B., Gibson F. Function of ubiquinone in Escherichia coli: a mutant strain forming a low level of ubiquinone. J Bacteriol. 1972 Jan;109(1):69–73. doi: 10.1128/jb.109.1.69-73.1972. [DOI] [PMC free article] [PubMed] [Google Scholar]
- Plate C. A. Mutant of Escherichia coli defective in response to colicin K and in active transport. J Bacteriol. 1976 Feb;125(2):467–474. doi: 10.1128/jb.125.2.467-474.1976. [DOI] [PMC free article] [PubMed] [Google Scholar]
- Rosen B. P., McClees J. S. Active transport of calcium in inverted membrane vesicles of Escherichia coli. Proc Natl Acad Sci U S A. 1974 Dec;71(12):5042–5046. doi: 10.1073/pnas.71.12.5042. [DOI] [PMC free article] [PubMed] [Google Scholar]
- Rosen B. P. Restoration of active transport in an Mg2+-adenosine triphosphatase-deficient mutant of Escherichia coli. J Bacteriol. 1973 Dec;116(3):1124–1129. doi: 10.1128/jb.116.3.1124-1129.1973. [DOI] [PMC free article] [PubMed] [Google Scholar]
- Simoni R. D., Postma P. W. The energetics of bacterial active transport. Annu Rev Biochem. 1975;44:523–554. doi: 10.1146/annurev.bi.44.070175.002515. [DOI] [PubMed] [Google Scholar]
- Taber H., Halfenger G. M. Multiple-aminoglycoside-resistant mutants of Bacillus subtilis deficient in accumulation of kanamycin. Antimicrob Agents Chemother. 1976 Feb;9(2):251–259. doi: 10.1128/aac.9.2.251. [DOI] [PMC free article] [PubMed] [Google Scholar]
- Tseng J. T., Bryan L. E., Van den Elzen H. M. Mechanisms and spectrum of streptomycin resistance in a natural population of Pseudomonas aeruginosa. Antimicrob Agents Chemother. 1972 Sep;2(3):136–141. doi: 10.1128/aac.2.3.136. [DOI] [PMC free article] [PubMed] [Google Scholar]
- Turnock G., Erickson S. K., Ackrell B. A., Birch B. A mutant of Escherichia coli with a defect in energy metabolism. J Gen Microbiol. 1972 May;70(3):507–515. doi: 10.1099/00221287-70-3-507. [DOI] [PubMed] [Google Scholar]
- Turnock G. The action of streptomycin in a mutant of Escherichia coli with increased sensitivity to the antibiotic. Biochem J. 1970 Jul;118(4):659–666. doi: 10.1042/bj1180659. [DOI] [PMC free article] [PubMed] [Google Scholar]
- WHITE D. C. THE FUNCTION OF 2-DEMETHYL VITAMIN K2 IN THE ELECTRON TRANSPORT SYSTEM OF HEMOPHILUS PARAINFLUENZAE. J Biol Chem. 1965 Mar;240:1387–1394. [PubMed] [Google Scholar]
- Whistance G. R., Dillon J. F., Threlfall D. R. The nature, intergeneric distribution and biosynthesis of isoprenoid quinones and phenols in gram-negative bacteria. Biochem J. 1969 Feb;111(4):461–472. doi: 10.1042/bj1110461. [DOI] [PMC free article] [PubMed] [Google Scholar]
- YAMAKI H., TANAKA N. EFFECTS OF PROTEIN SYNTHESIS INHIBITORS ON THE LETHAL ACTION OF KANAMYCIN AND STREPTOMYCIN. J Antibiot (Tokyo) 1963 Nov;16:222–226. [PubMed] [Google Scholar]