Abstract
Solubilities of deoxygenated sickle cell hemoglobin (deoxy-Hb S), at varying pH and temperature over a range of concentrations encompassing those found in erythrocytes, were measured. The technique involved ultracentrifugation, which gave values of the supernatant concentration and the mass of the sedimented material. The data establish that the solubility of doexy-Hb S is the saturation concentration and is independent of initial concentration. The mass of the pellet phase increases linearly with initial concentration. Moreover, the saturation concentration represents the critical concentration above which monomers are in equilibrium with polymers. These polymers are the putative cause of erythrocytes deformation associated with sickle cell anemia. The solubility-pH profiles of deoxy-Hb S at various temperatures, unlike those of other proteins, show no minima at the isoelectric pH but instead show a marked decrease in solubility below pH 7.0, indicating the predominance of polymerization over the expected increase in solubility. Deoxy-Hb S, within specified ranges of temperature and pH, possesses a negative temperature coefficient of solubility, a property characteristic of hydrophobic interactions. The saturation concentration is, however, temperature independent at conditions close to physiological. The enthalpy of polymerization (3.5 kcal/mol) is temperature independent from 6 degrees to 22 degrees for all pH values between 6.45 and 7.40. In the range of 22 degrees to 38 degrees, this parameter becomes less endothermic, having a value of 2.5 kcal/mol at pH 6.45 and a value of zero at pH 7.20. Such behavior of the system suggests a phase transition near 22 degreas. Within the range of conditions examined the polymerization is entropically driven.
Full text
PDF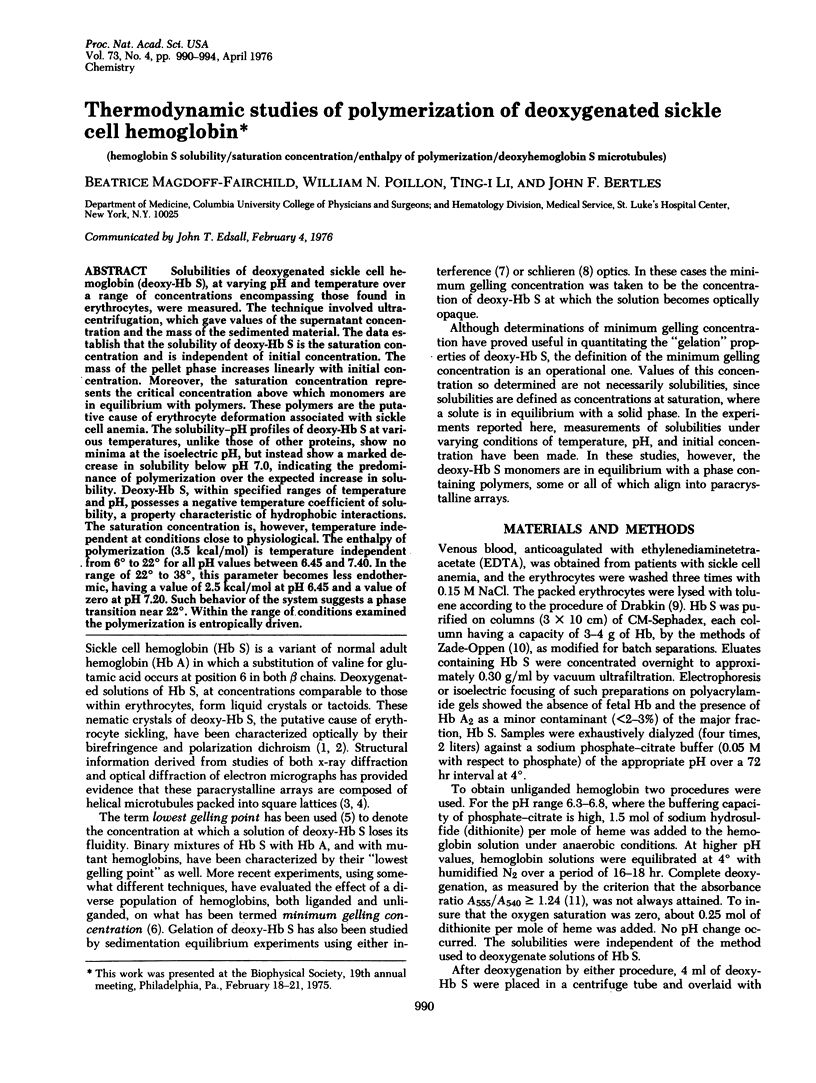
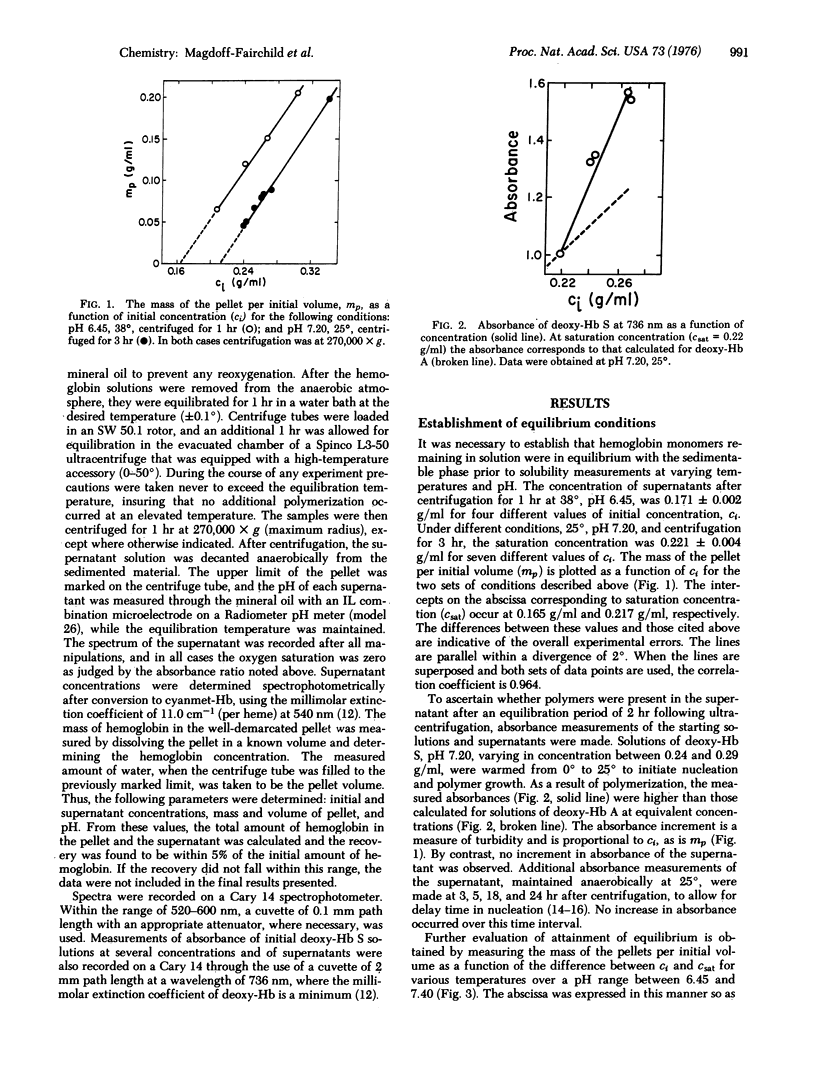
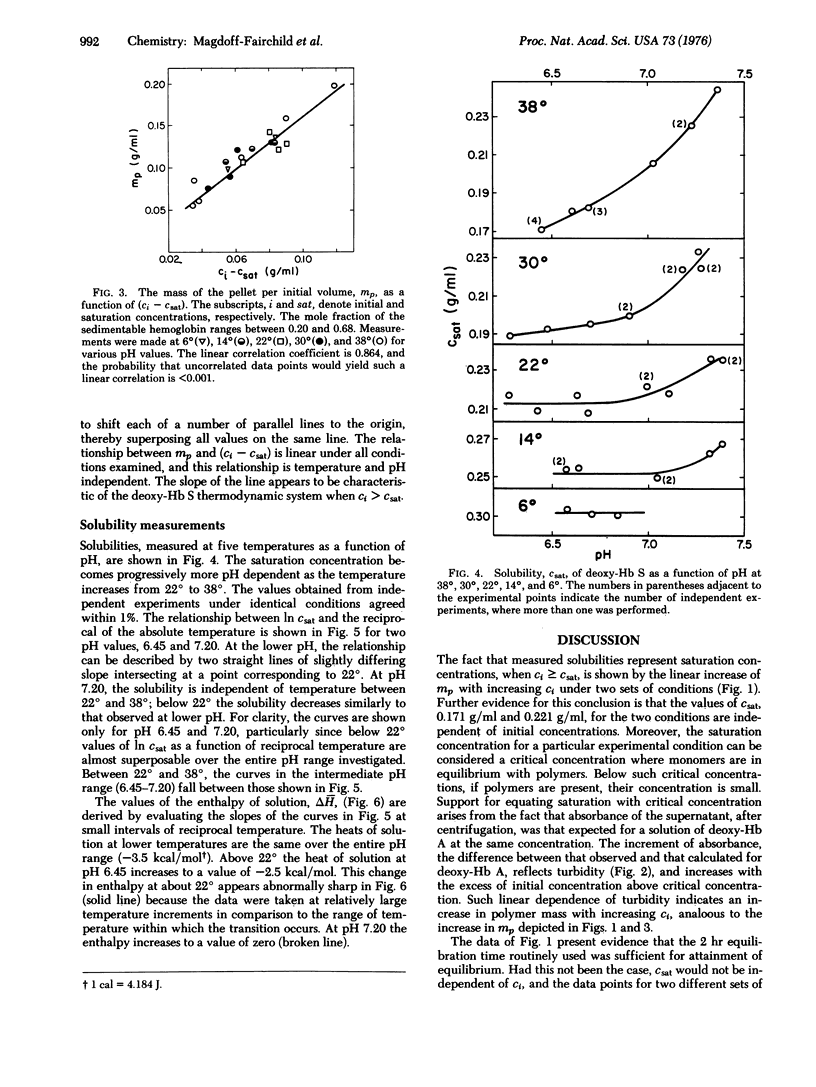
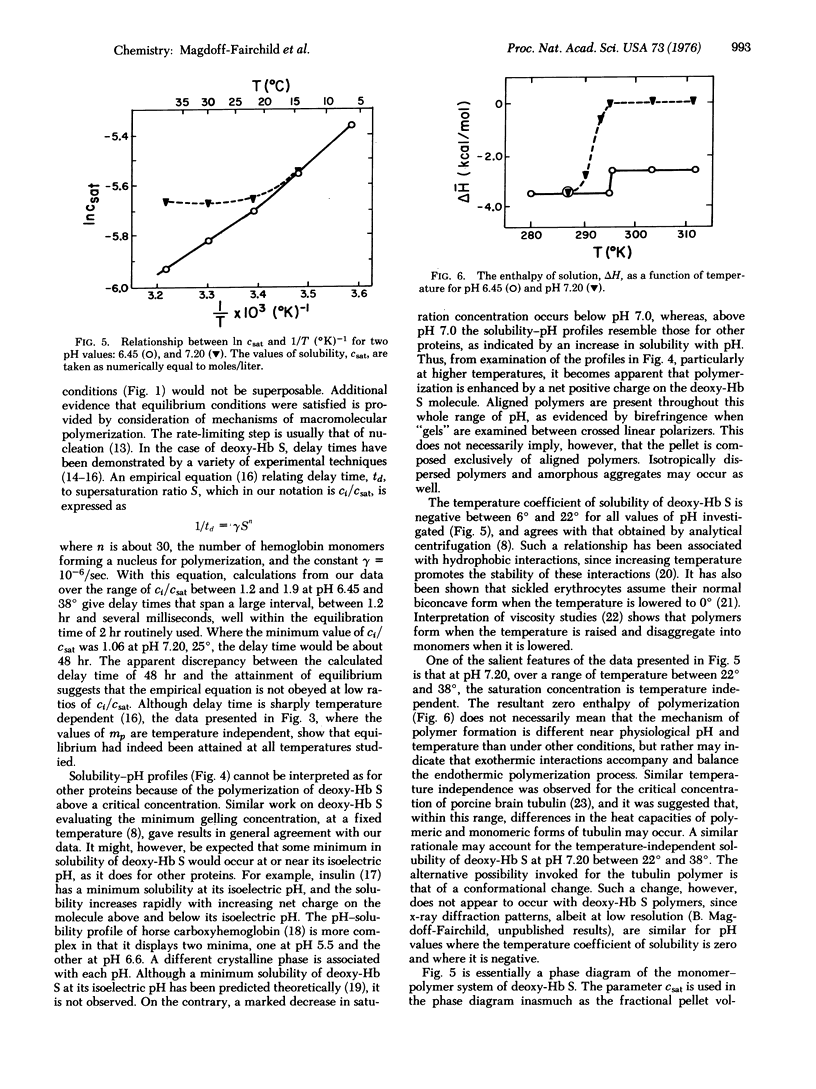
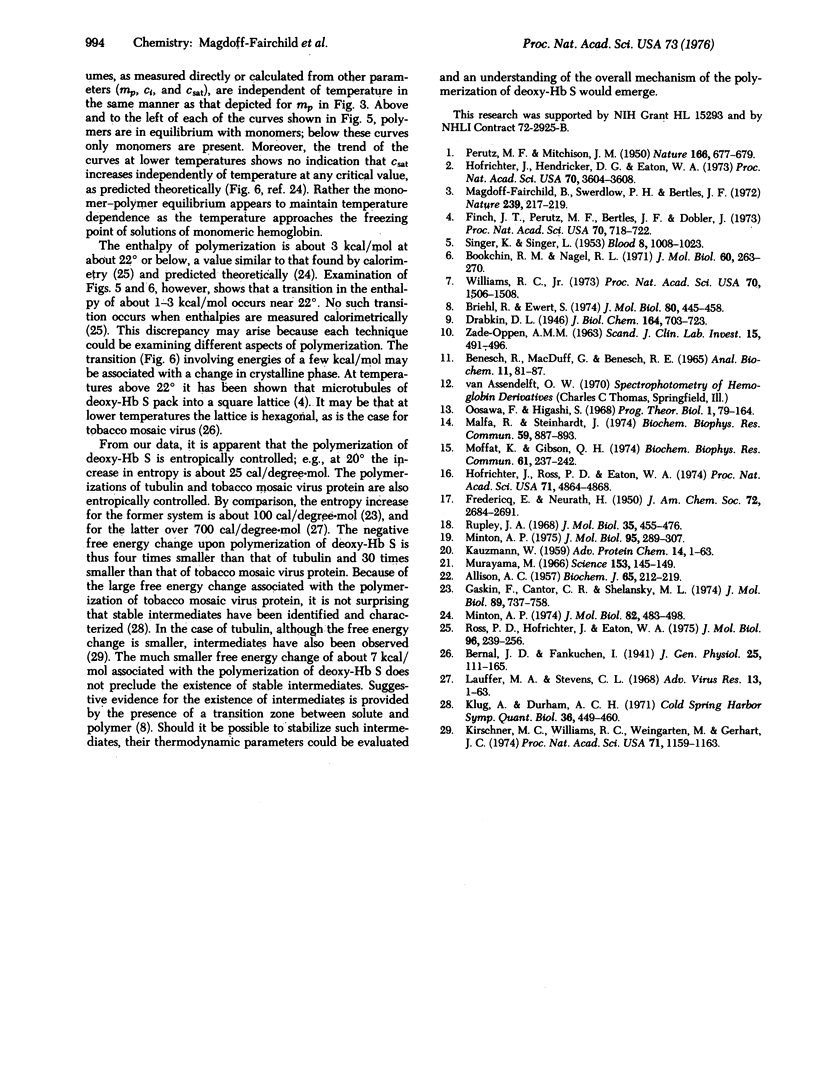
Selected References
These references are in PubMed. This may not be the complete list of references from this article.
- ALLISON A. C. Properties of sickle-cell haemoglobin. Biochem J. 1957 Feb;65(2):212–219. doi: 10.1042/bj0650212. [DOI] [PMC free article] [PubMed] [Google Scholar]
- BENESCH R., MACDUFF G., BENESCH R. E. DETERMINATION OF OXYGEN EQUILIBRIA WITH A VERSATILE NEW TONOMETER. Anal Biochem. 1965 Apr;11:81–87. doi: 10.1016/0003-2697(65)90045-x. [DOI] [PubMed] [Google Scholar]
- Bookchin R. M., Nagel R. L. Ligand-induced conformational dependence of hemoglobin in sickling interactios. J Mol Biol. 1971 Sep 14;60(2):263–270. doi: 10.1016/0022-2836(71)90292-0. [DOI] [PubMed] [Google Scholar]
- Briehl R. W., Ewert S. Effects of pH, 2,3-diphosphoglycerate and salts on gelation of sickle cell deoxyhemoglobin. J Mol Biol. 1973 Nov 5;80(3):445–458. doi: 10.1016/0022-2836(73)90415-4. [DOI] [PubMed] [Google Scholar]
- Finch J. T., Perutz M. F., Bertles J. F., Döbler J. Structure of sickled erythrocytes and of sickle-cell hemoglobin fibers. Proc Natl Acad Sci U S A. 1973 Mar;70(3):718–722. doi: 10.1073/pnas.70.3.718. [DOI] [PMC free article] [PubMed] [Google Scholar]
- Gaskin F., Cantor C. R., Shelanski M. L. Turbidimetric studies of the in vitro assembly and disassembly of porcine neurotubules. J Mol Biol. 1974 Nov 15;89(4):737–755. doi: 10.1016/0022-2836(74)90048-5. [DOI] [PubMed] [Google Scholar]
- Hofrichter J., Hendricker D. G., Eaton W. A. Structure of hemoglobin S fibers: optical determination of the molecular orientation in sickled erythrocytes. Proc Natl Acad Sci U S A. 1973 Dec;70(12):3604–3608. doi: 10.1073/pnas.70.12.3604. [DOI] [PMC free article] [PubMed] [Google Scholar]
- Hofrichter J., Ross P. D., Eaton W. A. Kinetics and mechanism of deoxyhemoglobin S gelation: a new approach to understanding sickle cell disease. Proc Natl Acad Sci U S A. 1974 Dec;71(12):4864–4868. doi: 10.1073/pnas.71.12.4864. [DOI] [PMC free article] [PubMed] [Google Scholar]
- KAUZMANN W. Some factors in the interpretation of protein denaturation. Adv Protein Chem. 1959;14:1–63. doi: 10.1016/s0065-3233(08)60608-7. [DOI] [PubMed] [Google Scholar]
- Kirschner M. W., Williams R. C., Weingarten M., Gerhart J. C. Microtubules from mammalian brain: some properties of their depolymerization products and a proposed mechanism of assembly and disassembly. Proc Natl Acad Sci U S A. 1974 Apr;71(4):1159–1163. doi: 10.1073/pnas.71.4.1159. [DOI] [PMC free article] [PubMed] [Google Scholar]
- Klug A., Durham A. C. The disk of TMV protein and its relation to the helical and other modes of aggregation. Cold Spring Harb Symp Quant Biol. 1972;36:449–460. doi: 10.1101/sqb.1972.036.01.057. [DOI] [PubMed] [Google Scholar]
- Lauffer M. A., Stevens C. L. Structure of the tobacco mosaic virus particle; polymerization of tobacco mosaic virus protein. Adv Virus Res. 1968;13:1–63. doi: 10.1016/s0065-3527(08)60250-x. [DOI] [PubMed] [Google Scholar]
- Magdoff-Fairchild B., Swerdlow P. H., Bertles J. F. Intermolecular organization of deoxygenated sickle haemoglobin determined by x-ray diffraction. Nature. 1972 Sep 22;239(5369):217–219. doi: 10.1038/239217a0. [DOI] [PubMed] [Google Scholar]
- Malfa R., Steinhardt J. A temperature-dependent latent-period in the aggregation of sickle-cell deoxyhemoglobin. Biochem Biophys Res Commun. 1974 Aug 5;59(3):887–893. doi: 10.1016/s0006-291x(74)80062-8. [DOI] [PubMed] [Google Scholar]
- Minton A. P. A thermodynamic model for gelation of sickle-cell hemoglobin. J Mol Biol. 1974 Feb 5;82(4):483–498. doi: 10.1016/0022-2836(74)90243-5. [DOI] [PubMed] [Google Scholar]
- Minton A. P. Thermodynamic analysis of the chemical inhibition of sickle-cell hemoglobin gelation. J Mol Biol. 1975 Jun 25;95(2):289–307. doi: 10.1016/0022-2836(75)90396-4. [DOI] [PubMed] [Google Scholar]
- Moffat K., Gibson Q. H. The rates of polymerization and depolymerization of sickle cell hemoglobin. Biochem Biophys Res Commun. 1974 Nov 6;61(1):237–242. doi: 10.1016/0006-291x(74)90558-0. [DOI] [PubMed] [Google Scholar]
- Murayama M. Molecular mechanism of red cell "sickling". Science. 1966 Jul 8;153(3732):145–149. doi: 10.1126/science.153.3732.145. [DOI] [PubMed] [Google Scholar]
- PERUTZ M. F., MITCHISON J. M. State of haemoglobin in sickle-cell anaemia. Nature. 1950 Oct 21;166(4225):677–679. doi: 10.1038/166677a0. [DOI] [PubMed] [Google Scholar]
- Ross P. D., Hofrichter J., Eaton W. A. Calorimetric and optical characterization of sickle cell hemoglobin gelation. J Mol Biol. 1975 Aug 5;96(2):239–253. doi: 10.1016/0022-2836(75)90345-9. [DOI] [PubMed] [Google Scholar]
- Rupley J. A. Comparison of protein structure in the crystal and in solution. IV. Protein solubility. J Mol Biol. 1968 Aug 14;35(3):455–476. doi: 10.1016/s0022-2836(68)80006-3. [DOI] [PubMed] [Google Scholar]
- SINGER K., SINGER L. Studies on abnormal hemoglobins. VIII. The gelling phenomenon of sickle cell hemoglobin: its biologic and diagnostic significance. Blood. 1953 Nov;8(11):1008–1023. [PubMed] [Google Scholar]
- Williams R. C., Jr Concerted formation of the gel of hemoglobin S. Proc Natl Acad Sci U S A. 1973 May;70(5):1506–1508. doi: 10.1073/pnas.70.5.1506. [DOI] [PMC free article] [PubMed] [Google Scholar]