Abstract
The utilization of the isoaccepting species of leucyl-tRNA in protein synthesis has been examined in E. coli. Two minor leucyl-tRNA species, isoacceptors tRNA3Leu and tRNA4Leu, are the predominant species found bound to ribosomes during exponential growth in minimal medium. In rich medium, an increased proportion of tRNA1Leu is found bound to ribosomes. One species, tRNA5Leu, is always absent from ribosomes. In a mutant strain in which normal tRNA3Leu and tRNA5Leu are reduced or absent, tRNA1Leu is a major ribosome-bound species in minimal medium. Protein synthesis in vitro with RNA from E. coli further suggests that tRNA5Leu is rarely used for total protein synthesis; however, this species is active with RNA from MS2 phage. We propose that tRNA1Leu can substitute for tRNA3Leu under rapid growth conditions, and that tRNA5Leu is used minimally in total protein synthesis.
Full text
PDF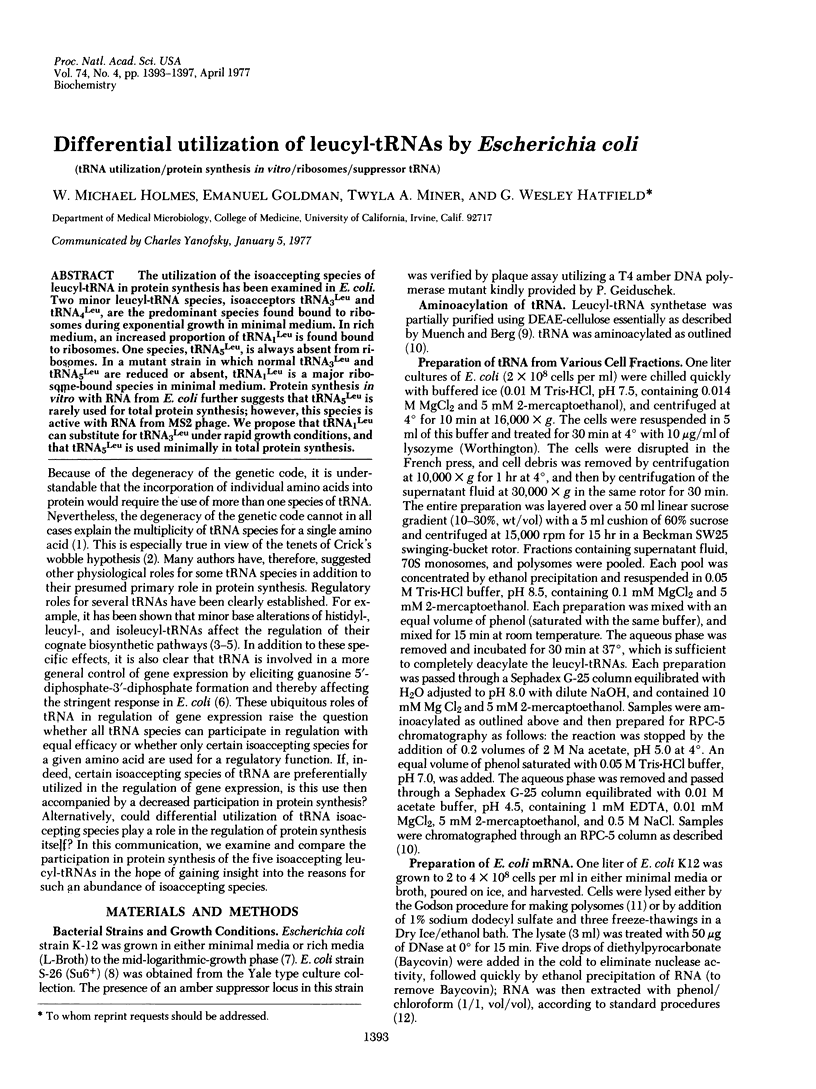
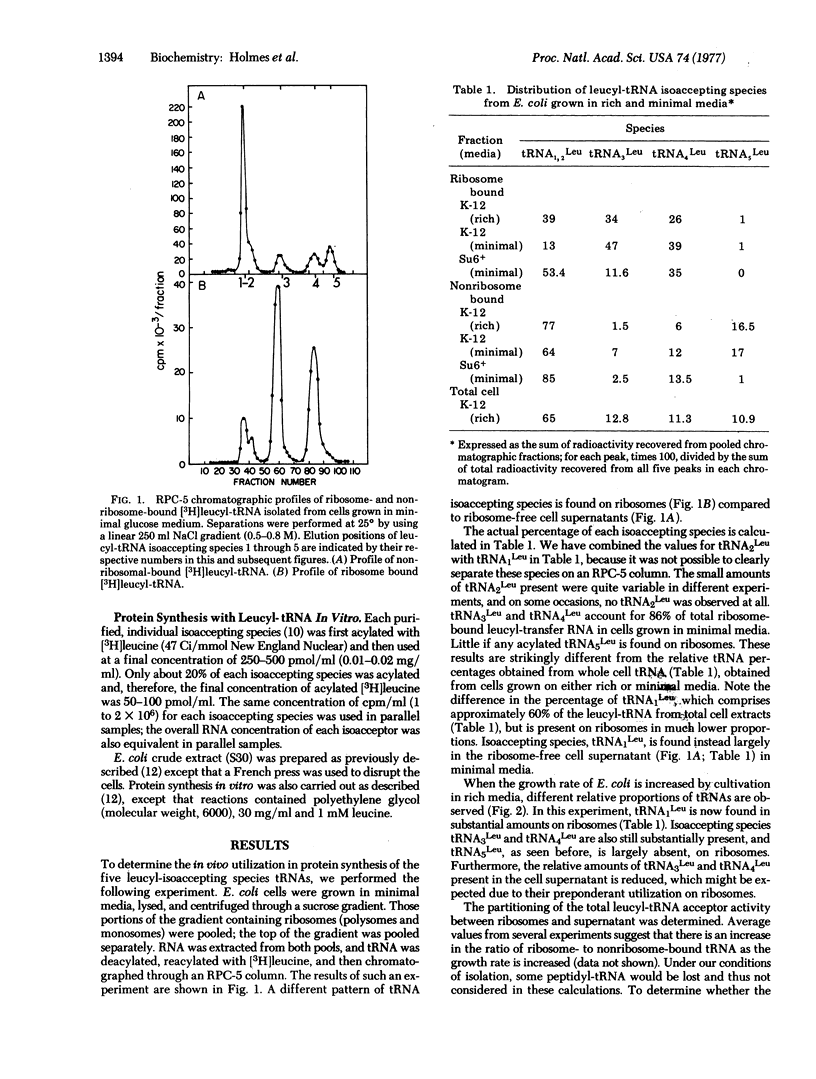
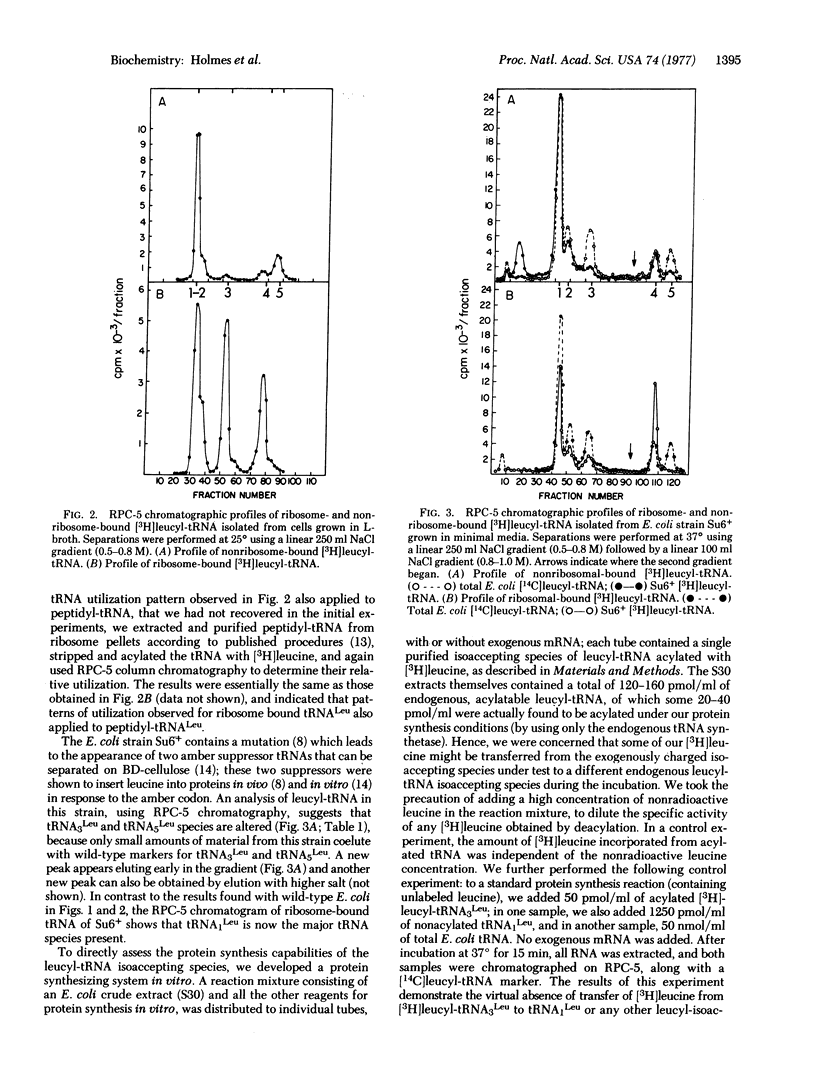
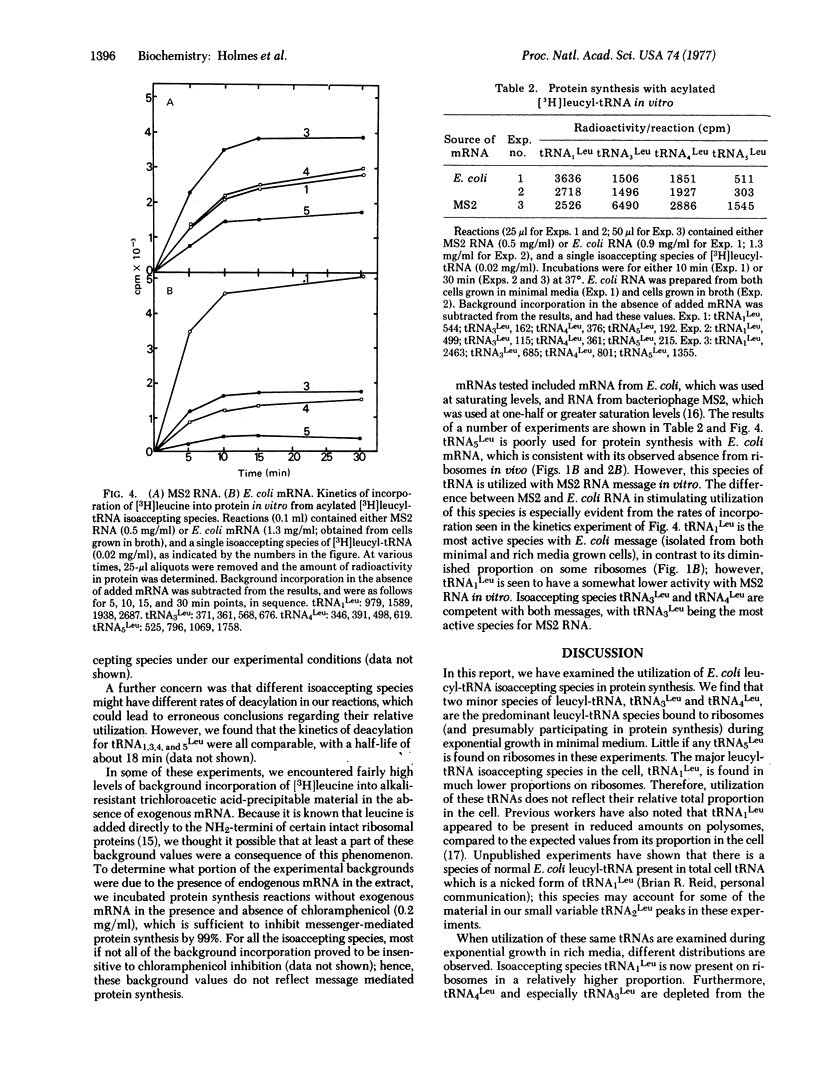
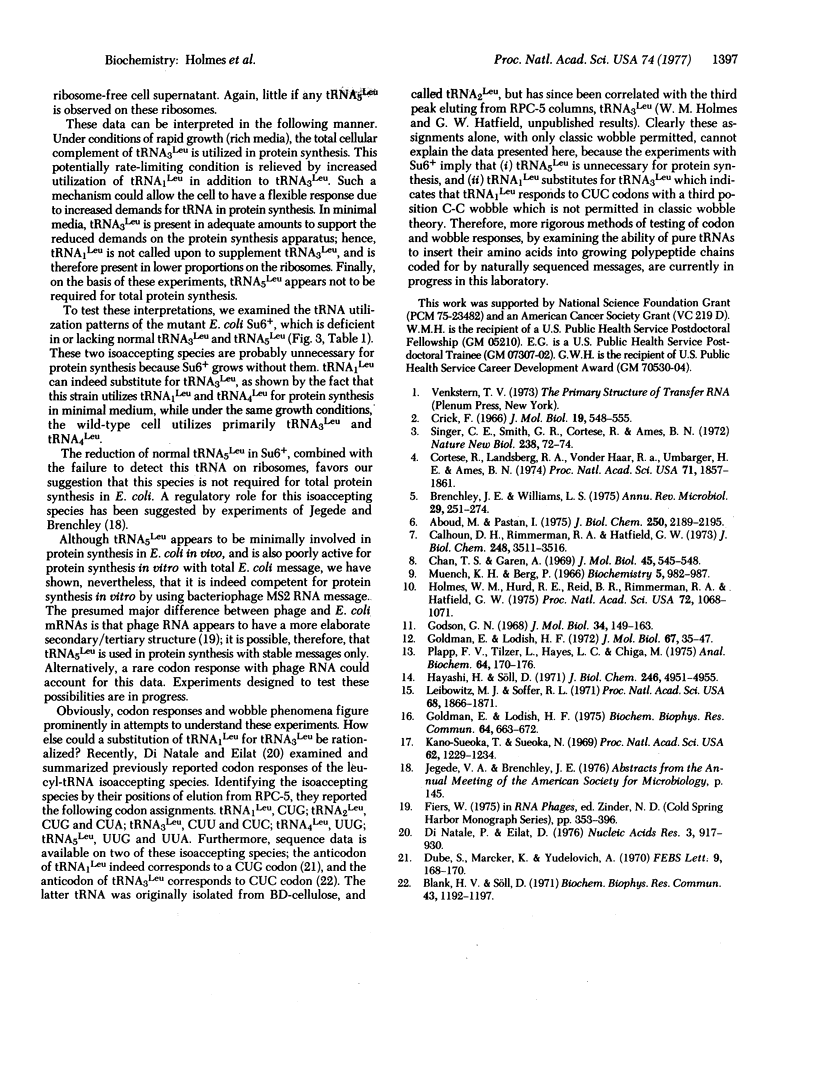
Selected References
These references are in PubMed. This may not be the complete list of references from this article.
- Aboud M., Pastan I. Activation of transcription by guanosine 5'-diphosphate,3'-diphosphate, transfer ribonucleic acid, and novel protein from Escherichia coli. J Biol Chem. 1975 Mar 25;250(6):2189–2195. [PubMed] [Google Scholar]
- Blank H. U., Söll D. The nucleotide sequence of two leucine tRNA species from Escherichia coli K12. Biochem Biophys Res Commun. 1971 Jun 4;43(5):1192–1197. doi: 10.1016/0006-291x(71)90589-4. [DOI] [PubMed] [Google Scholar]
- Calhoun D. H., Rimerman R. A., Hatfield G. W. Threonine deaminase from Escherichia coli. I. Purification and properties. J Biol Chem. 1973 May 25;248(10):3511–3516. [PubMed] [Google Scholar]
- Chan T. S., Garen A. Amino acid substitutions resulting from suppression of nonsense mutations. IV. Leucine insertion by the Su6+ suppressor gene. J Mol Biol. 1969 Nov 14;45(3):545–548. doi: 10.1016/0022-2836(69)90311-8. [DOI] [PubMed] [Google Scholar]
- Cortese R., Landsberg R., Haar R. A., Umbarger H. E., Ames B. N. Pleiotropy of hisT mutants blocked in pseudouridine synthesis in tRNA: leucine and isoleucine-valine operons. Proc Natl Acad Sci U S A. 1974 May;71(5):1857–1861. doi: 10.1073/pnas.71.5.1857. [DOI] [PMC free article] [PubMed] [Google Scholar]
- Crick F. H. Codon--anticodon pairing: the wobble hypothesis. J Mol Biol. 1966 Aug;19(2):548–555. doi: 10.1016/s0022-2836(66)80022-0. [DOI] [PubMed] [Google Scholar]
- Di Natale P., Eilat D. Patterns of E. coli leucine tRNA isoacceptors following bacteriophage MS2 infection. Nucleic Acids Res. 1976 Apr;3(4):917–930. doi: 10.1093/nar/3.4.917. [DOI] [PMC free article] [PubMed] [Google Scholar]
- Dube S. K., Marcker K. A., Yudelevich A. The nucleotide sequence of a leucine transfer RNA from E. coli. FEBS Lett. 1970 Sep 6;9(3):168–170. doi: 10.1016/0014-5793(70)80345-3. [DOI] [PubMed] [Google Scholar]
- Godson G. N. Site of synthesis of viral ribonucleic acid and phage assembly in MS2-infected Escherichia coli. J Mol Biol. 1968 May 28;34(1):149–163. doi: 10.1016/0022-2836(68)90241-6. [DOI] [PubMed] [Google Scholar]
- Goldman E., Lodish H. F. Competition between bacteriophage f2 RNA and bacteriophage T4 messenger RNA. Biochem Biophys Res Commun. 1975 May 19;64(2):663–672. doi: 10.1016/0006-291x(75)90372-1. [DOI] [PubMed] [Google Scholar]
- Goldman E., Lodish H. F. Specificity of protein synthesis by bacterial ribosomes and initiation factors: absence of change after phage T4 infection. J Mol Biol. 1972 Jun 14;67(1):35–47. doi: 10.1016/0022-2836(72)90384-1. [DOI] [PubMed] [Google Scholar]
- Hayashi H., Söll D. Purification of an Escherichia coli leucine suppressor transfer ribonucleic acid and its aminoacylation by the homologous leucyl-transfer ribonucleic acid synthetase. J Biol Chem. 1971 Aug 25;246(16):4951–4954. [PubMed] [Google Scholar]
- Holmes W. M., Hurd R. E., Reid B. R., Rimerman R. A., Hatfield G. W. Separation of transfer ribonucleic acid by sepharose chromatography using reverse salt gradients. Proc Natl Acad Sci U S A. 1975 Mar;72(3):1068–1071. doi: 10.1073/pnas.72.3.1068. [DOI] [PMC free article] [PubMed] [Google Scholar]
- Kano-Sueoka T., Sueoka N. Leucine tRNA and cessation of Escherichia coli protein synthesis upon phage T2 infection. Proc Natl Acad Sci U S A. 1969 Apr;62(4):1229–1236. doi: 10.1073/pnas.62.4.1229. [DOI] [PMC free article] [PubMed] [Google Scholar]
- Leibowitz M. J., Soffer R. L. Modification of a specific ribosomal protein catalyzed by leucyl, phenylalanyl-tRNA: protein transferase. Proc Natl Acad Sci U S A. 1971 Aug;68(8):1866–1869. doi: 10.1073/pnas.68.8.1866. [DOI] [PMC free article] [PubMed] [Google Scholar]
- Muench K. H., Berg P. Resolution of aminoacyl transfer ribonucleic acid by hydroxylapatite chromatography. Biochemistry. 1966 Mar;5(3):982–987. doi: 10.1021/bi00867a025. [DOI] [PubMed] [Google Scholar]
- Plapp F. V., Tilzer L., Hayes L. C., Chiga M. A rapid method for isolation of peptidyl tRNA from liver polysomes. Anal Biochem. 1975 Mar;64(1):170–176. doi: 10.1016/0003-2697(75)90418-2. [DOI] [PubMed] [Google Scholar]
- Singer C. E., Smith G. R., Cortese R., Ames B. N. [Mutant tRNA His ineffective in repression and lacking two pseudouridine modifications]. Nat New Biol. 1972 Jul 19;238(81):72–74. doi: 10.1038/newbio238072a0. [DOI] [PubMed] [Google Scholar]