Abstract
Ehrlich cells treated with dinitrophenol and iodoacetate rapidly recover their 30-sec uptake of 2-(methyl-amino)-isobutyrate on treatment with 0.1 mM phenazine methosulfate + 20 mM sodium ascorbate before they begin to recover from the severely depressed ATP levels and alkali-ion gradients. Addition of 10 mM pyruvate also restores uptake of methylaminoisobutyrate before the alkali-ion gradients rise. This restoration is prevented by rotenone, but rotenone does not handicap restoration by phenazine methosulfate/ascorbate. Na+-independent uptake of 2-aminonorbornane-2-carboxylate by Ehrlich cells is affected the same way. Quinacrine almost completely suppresses uptake of methylaminoisobutyrate within the 30-sec uptake test, even when ATP levels are sustained by pyruvate and alkali-ion gradients are not depressed. Ouabain prevents restoration of both Na+-dependent and Na+-independent amino-acid transport by phenazine methosulfate/ascorbate or pyruvate. We interpret these results to indicate that amino-acid transport can be energized not only by known means, but also by reducing equivalents, which presumably reach the plasma membrane in the form of NADH from the mitochondria when the source of energy is pyruvate. In support of this hypothesis, the distribution of methylaminoisobutyrate between plasma membrane vesicles and their supporting media was influenced in the predictable way by NADH, quinacrine, and an uncoupling agent, proceeding on the assumption that more of the vesicles had the everted rather than the natural orientation.
Full text
PDF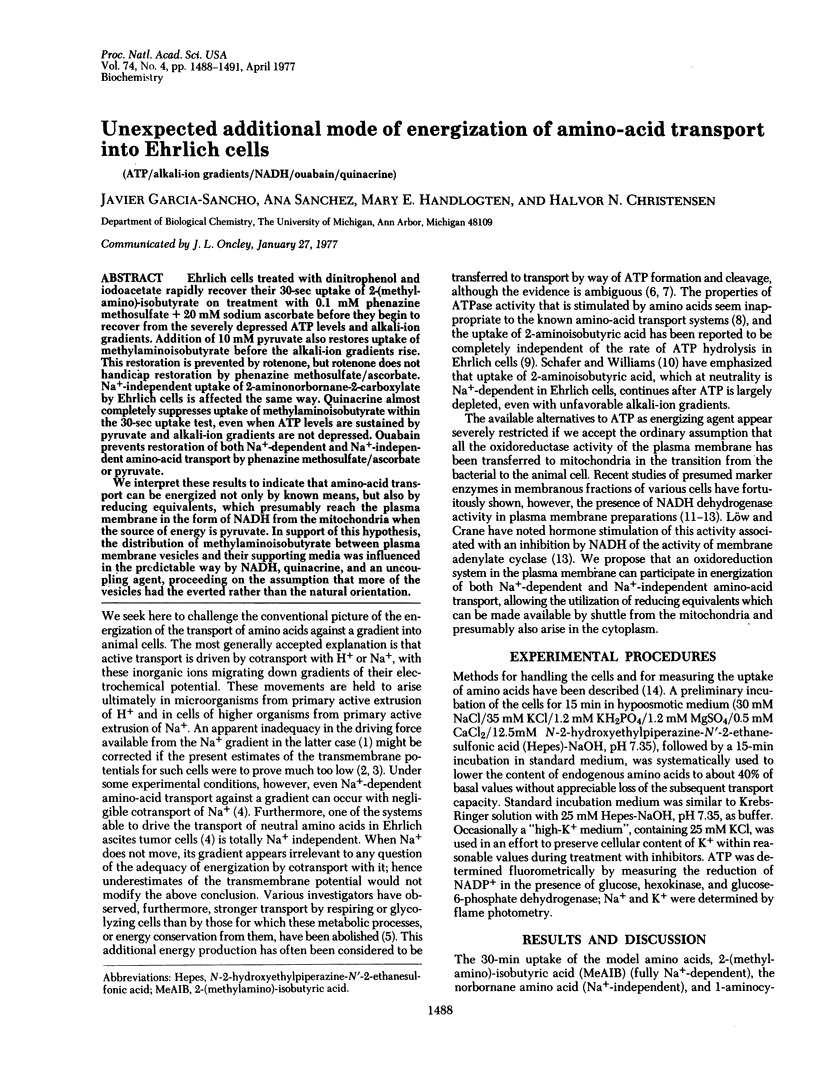
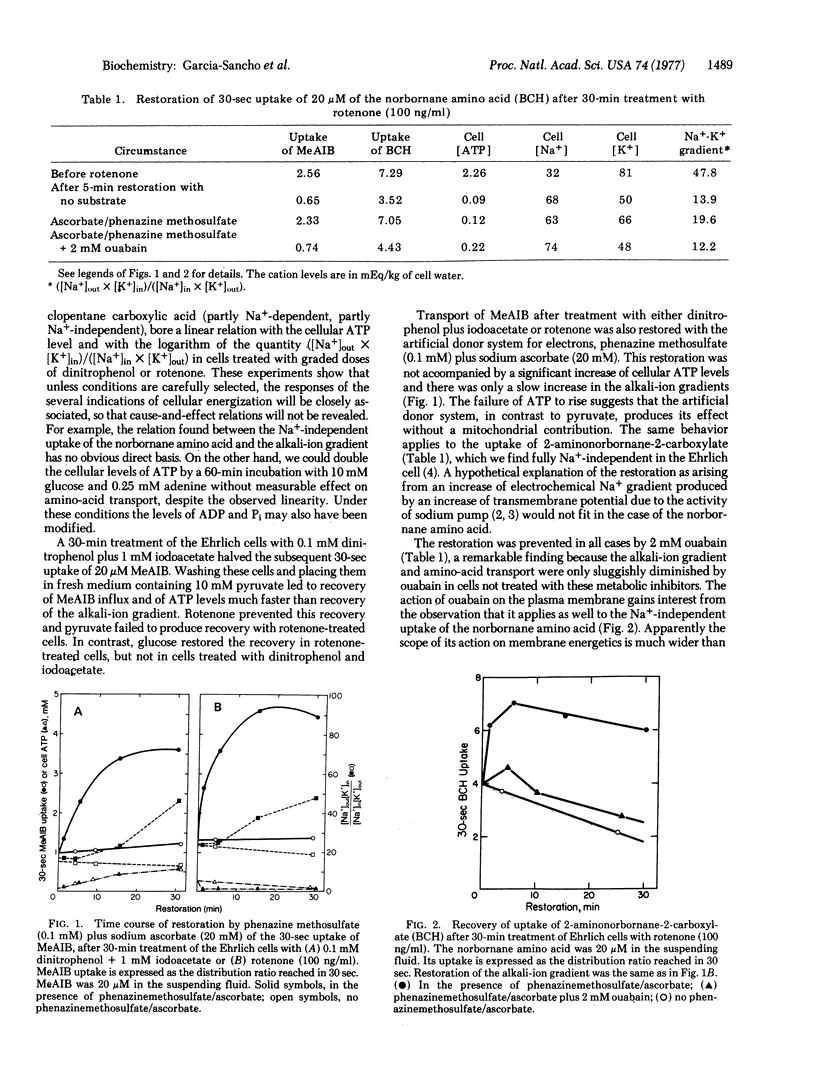
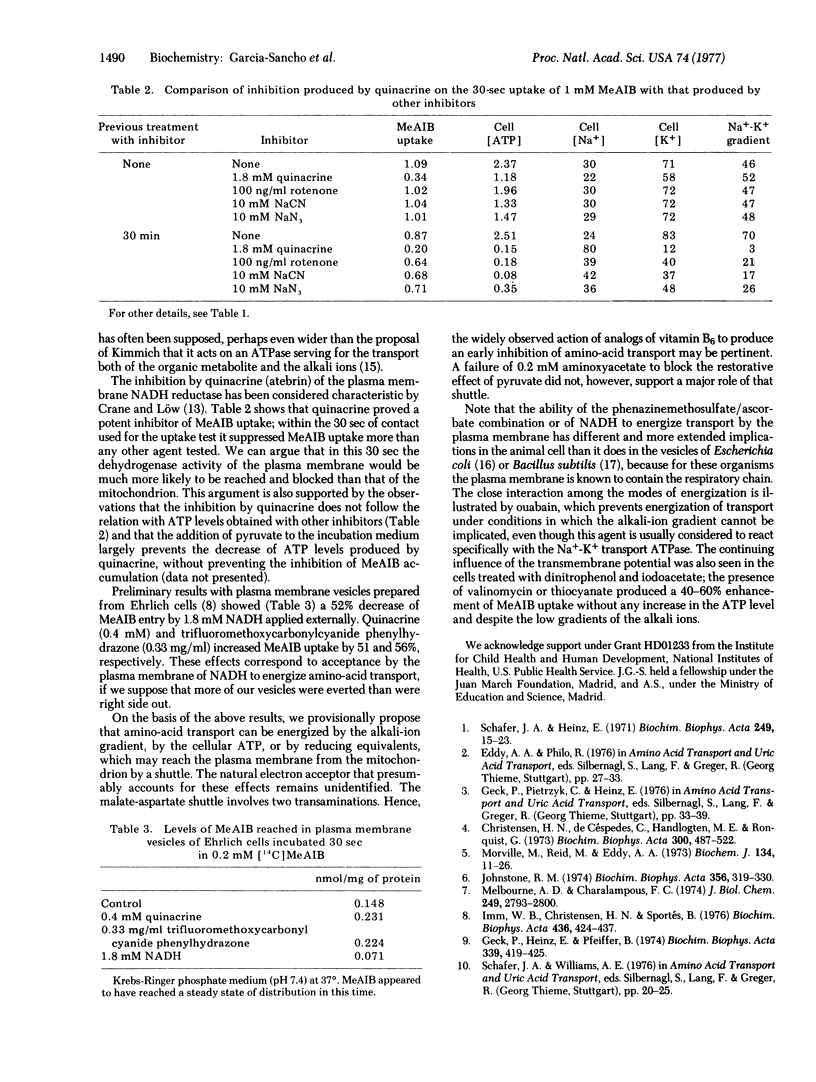
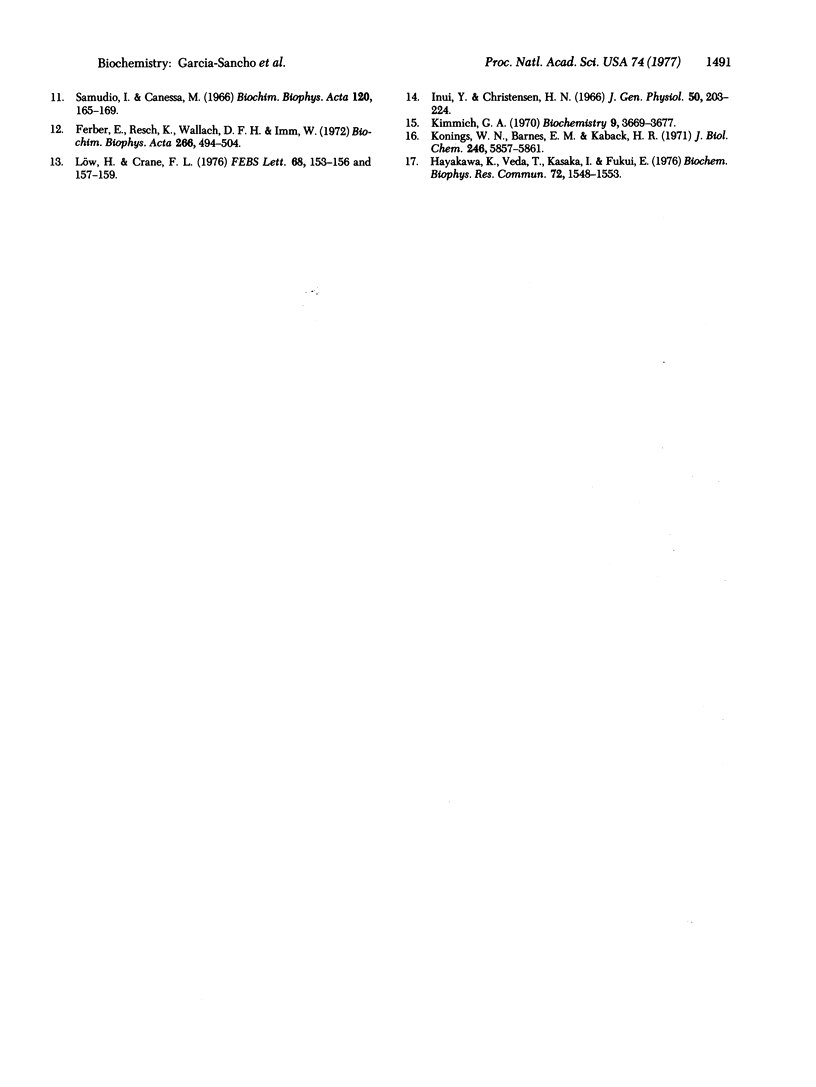
Selected References
These references are in PubMed. This may not be the complete list of references from this article.
- Christensen H. N., de Cespedes C., Handlogten M. E., Ronquist G. Energization of amino acid transport, studied for the Ehrlich ascites tumor cell. Biochim Biophys Acta. 1973 Dec 28;300(4):487–522. doi: 10.1016/0304-4157(73)90017-8. [DOI] [PubMed] [Google Scholar]
- Crane F. L., Löw H. NADH oxidation in liver and fat cell plasma membranes. FEBS Lett. 1976 Oct 1;68(2):153–156. doi: 10.1016/0014-5793(76)80425-5. [DOI] [PubMed] [Google Scholar]
- Ferber E., Resch K., Wallach D. F., Imm W. Isolation and characterization of lymphocyte plasma membranes. Biochim Biophys Acta. 1972 May 9;266(2):494–504. doi: 10.1016/0005-2736(72)90105-8. [DOI] [PubMed] [Google Scholar]
- Geck P., Heinz E., Pfeiffer B. Evidence against direct coupling between amino acid transport and ATP hydrolysis. Biochim Biophys Acta. 1974 Mar 29;339(3):419–425. doi: 10.1016/0005-2736(74)90169-2. [DOI] [PubMed] [Google Scholar]
- Hayakawa K., Ueda T., Kusaka I., Fukui S. Preparation of artifical vesicles having an L-alanine uptake activity which requires NADH as energy source. Biochem Biophys Res Commun. 1976 Oct 18;72(4):1548–1553. doi: 10.1016/s0006-291x(76)80190-8. [DOI] [PubMed] [Google Scholar]
- Im W. B., Christensen H. N., Sportés B. Amino acid stimulation of ATP cleavage by two Ehrlich cell membrane preparations in the presence of ouabain. Biochim Biophys Acta. 1976 Jun 17;436(2):424–437. doi: 10.1016/0005-2736(76)90205-4. [DOI] [PubMed] [Google Scholar]
- Inui Y., Christensen H. N. Discrimination of single transport systems. The Na plus-sensitive transport of neutral amino acids in the Ehrlich cell. J Gen Physiol. 1966 Sep;50(1):203–224. doi: 10.1085/jgp.50.1.203. [DOI] [PMC free article] [PubMed] [Google Scholar]
- Johnstone R. M. Role of ATP on the initial rate of amino acid uptake in Ehrlich ascites cells. Biochim Biophys Acta. 1974 Aug 9;356(3):319–330. doi: 10.1016/0005-2736(74)90272-7. [DOI] [PubMed] [Google Scholar]
- Kimmich G. A. Active sugar accumulation by isolated intestinal epithelial cells. A new model for sodium-dependent metabolite transport. Biochemistry. 1970 Sep 15;9(19):3669–3677. doi: 10.1021/bi00821a004. [DOI] [PubMed] [Google Scholar]
- Konings W. N., Barnes E. M., Jr, Kaback H. R. Mechanisms of active transport in isolated membrane vesicles. 2. The coupling of reduced phenazine methosulfate to the concentrative uptake of beta-galactosides and amino acids. J Biol Chem. 1971 Oct 10;246(19):5857–5861. [PubMed] [Google Scholar]
- Melbourne A. D., Charalampous F. C. Energy source for active transport of alpha-aminoisobutyric acid in KB cells. J Biol Chem. 1974 May 10;249(9):2793–2800. [PubMed] [Google Scholar]
- Morville M., Reid M., Eddy A. A. Amino acid absorption by mouse ascites-tumour cells depleted of both endogenous amino acids and adenosine triphosphate. Biochem J. 1973 May;134(1):11–26. doi: 10.1042/bj1340011. [DOI] [PMC free article] [PubMed] [Google Scholar]
- Schafer J. A., Heinz E. The effect of reversal on Na + and K + electrochemical potential gradients on the active transport of amino acids in Ehrlich ascites tumor cells. Biochim Biophys Acta. 1971 Oct 12;249(1):15–33. doi: 10.1016/0005-2736(71)90079-4. [DOI] [PubMed] [Google Scholar]
- Zamudio I., Canessa M. Nicotinamide-adenine dinucleotide dehydrogenase activity of human erythrocyte membranes. Biochim Biophys Acta. 1966 May 12;120(1):165–169. doi: 10.1016/0926-6585(66)90290-1. [DOI] [PubMed] [Google Scholar]