Abstract
A theoretical analysis of monocyclic cascade models shows that the steady-state fraction of covalently modified interconvertible enzyme is a function of 10 different cascade parameters. Because each parameter can be varied independently, or several can be varied simultaneously, by single or multiple allosteric interactions of ligands with one or more of the cascade enzymes, interconvertible enzymes are exquisitely designed for the rigorous control of key metabolic steps. Compared with other reglatory enzymes, they can respond to a greater number of allosteric stimuli, they exhibit greater flexibility in overall control patterns, and they can generate a greatly amplified response to primary allosteric interactions of effectors with the converter enzymes. Contrary to earlier views, the decomposition of ATP associated with cyclic coupling of the covalent modification and demodification reactions is not a futile process. ATP decomposition supplies the energy needed to maintain concentrations of modified enzyme at steady-state levels that are in excess of those obtainable at true thermodynamic equilibrium.
Full text
PDF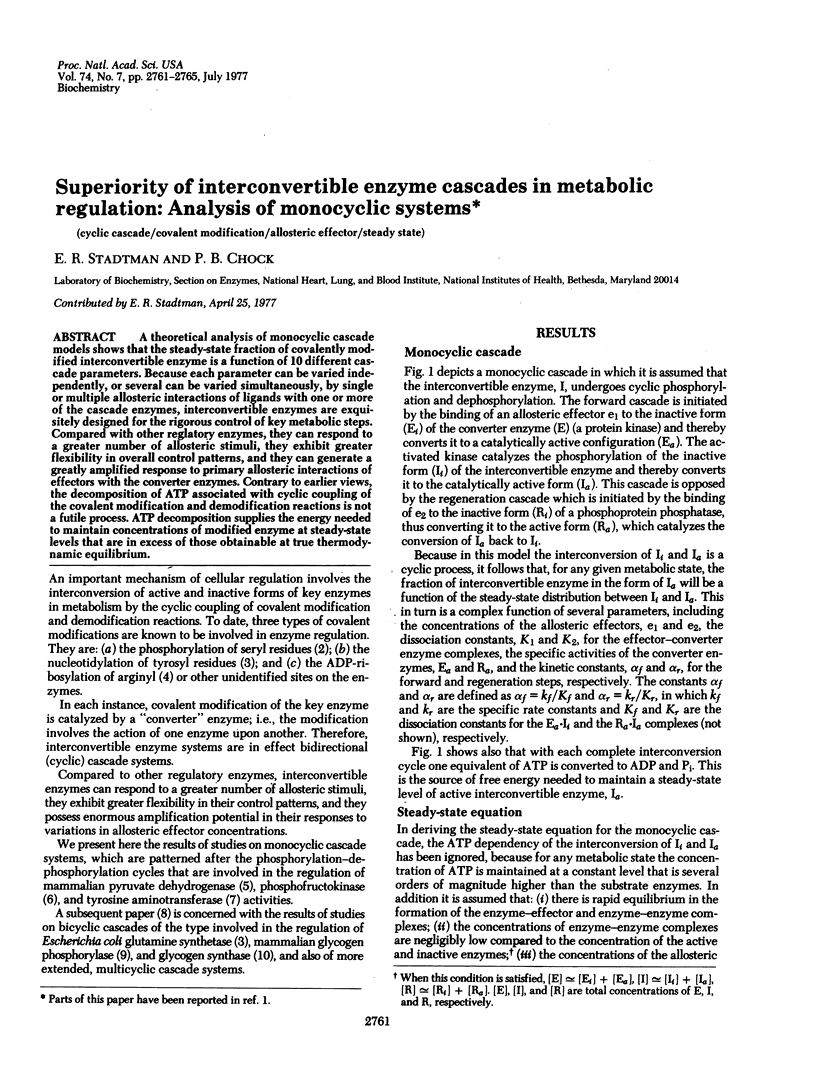
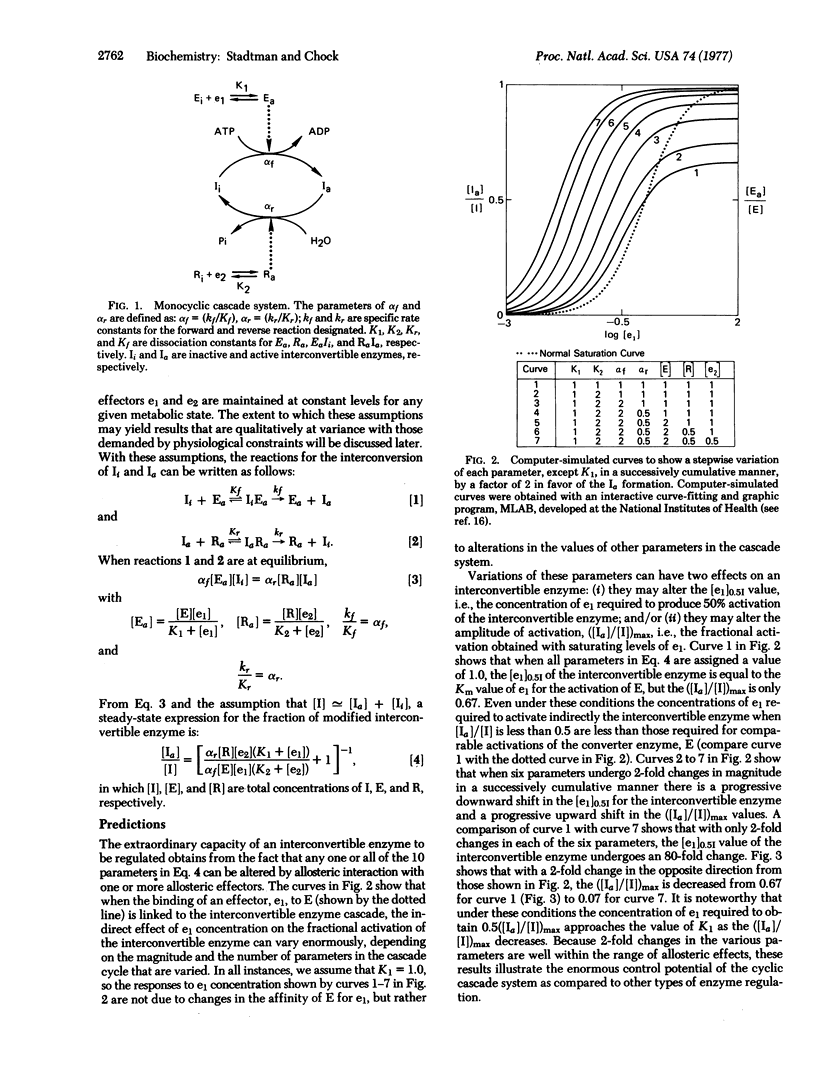
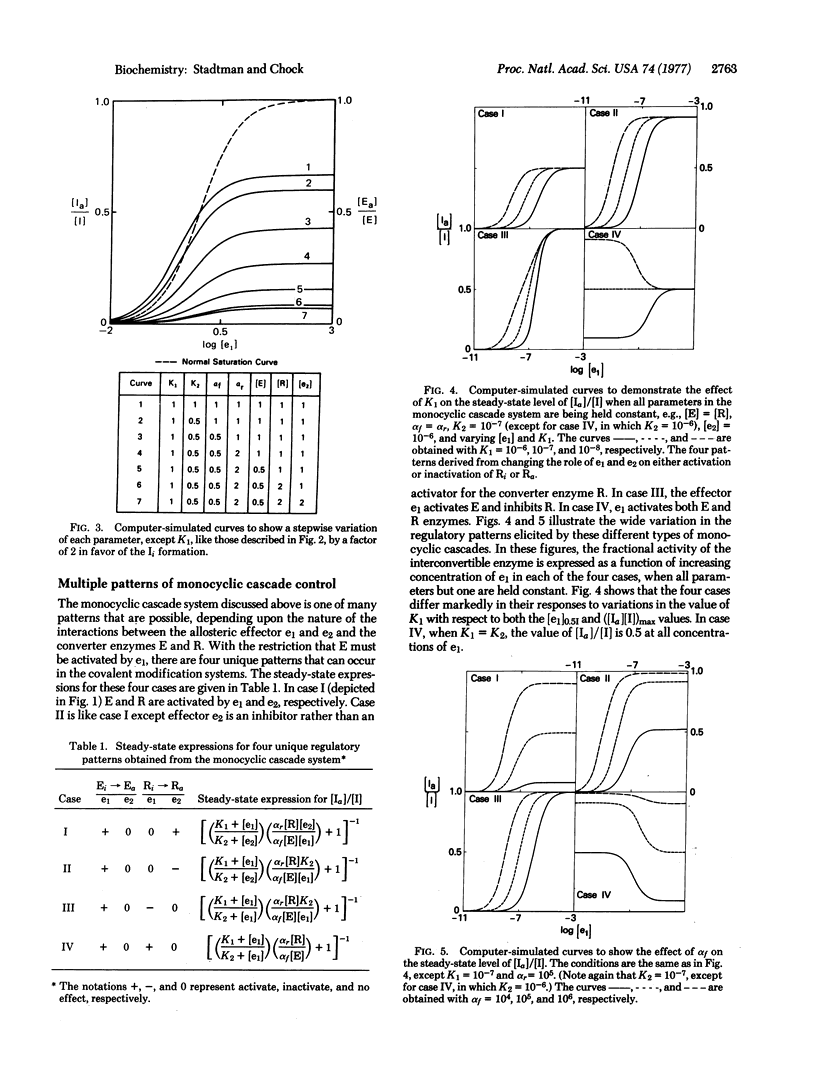
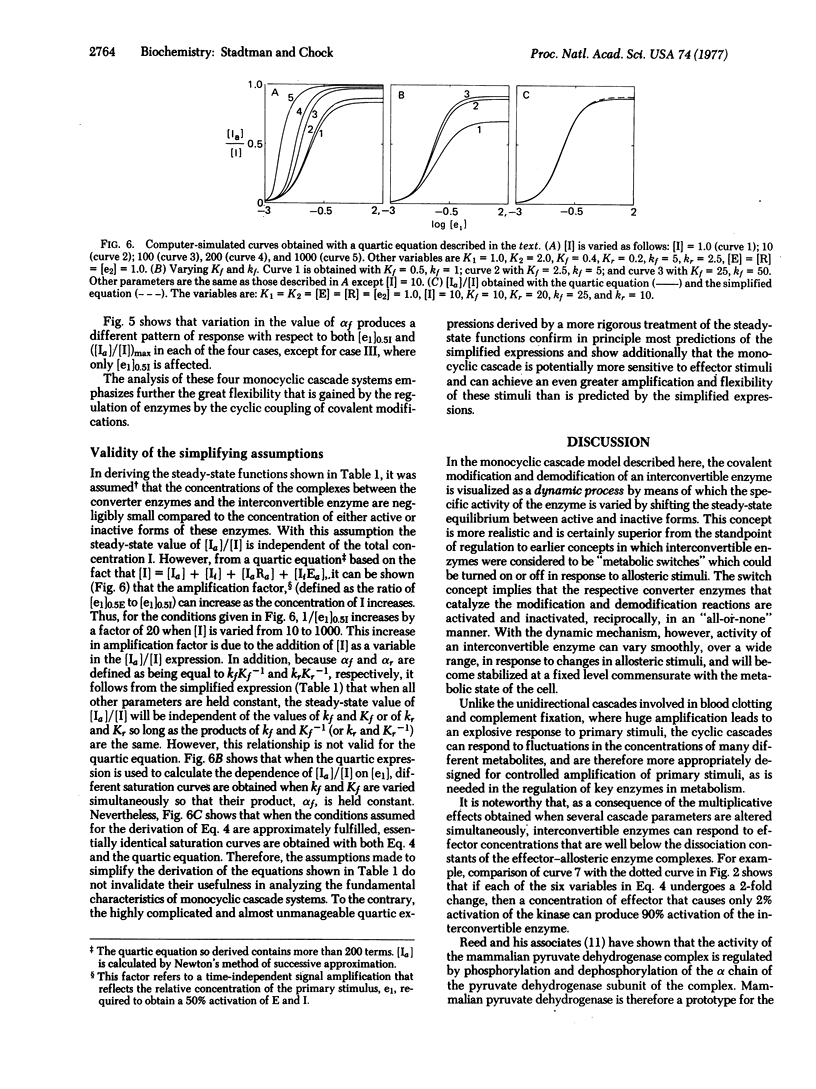
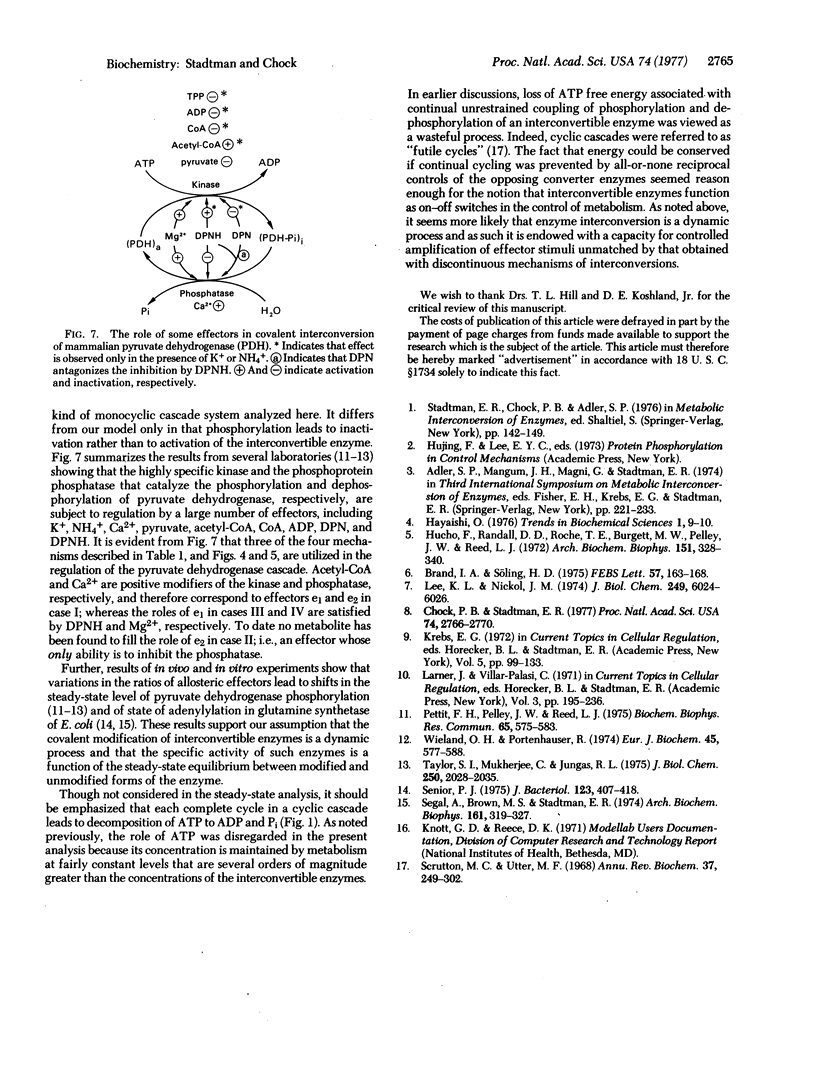
Selected References
These references are in PubMed. This may not be the complete list of references from this article.
- Brand I. A., Söling H. D. Activation and inactivation of rat liver phosphofructokinase by phosphorylation-dephosphorylation. FEBS Lett. 1975 Sep 15;57(2):163–168. doi: 10.1016/0014-5793(75)80707-1. [DOI] [PubMed] [Google Scholar]
- Chock P. B., Stadtman E. R. Superiority of interconvertible enzyme cascades in metabolite regulation: analysis of multicyclic systems. Proc Natl Acad Sci U S A. 1977 Jul;74(7):2766–2770. doi: 10.1073/pnas.74.7.2766. [DOI] [PMC free article] [PubMed] [Google Scholar]
- Hucho F., Randall D. D., Roche T. E., Burgett M. W., Pelley J. W., Reed L. J. -Keto acid dehydrogenase complexes. XVII. Kinetic and regulatory properties of pyruvate dehydrogenase kinase and pyruvate dehydrogenase phosphatase from bovine kidney and heart. Arch Biochem Biophys. 1972 Jul;151(1):328–340. doi: 10.1016/0003-9861(72)90504-8. [DOI] [PubMed] [Google Scholar]
- Lee K. L., Nickol J. M. Phosphorylation of tyrosine aminotransferase in vivo. J Biol Chem. 1974 Sep 25;249(18):6024–6026. [PubMed] [Google Scholar]
- Pettit F. H., Pelley J. W., Reed L. J. Regulation of pyruvate dehydrogenase kinase and phosphatase by acetyl-CoA/CoA and NADH/NAD ratios. Biochem Biophys Res Commun. 1975 Jul 22;65(2):575–582. doi: 10.1016/s0006-291x(75)80185-9. [DOI] [PubMed] [Google Scholar]
- Senior P. J. Regulation of nitrogen metabolism in Escherichia coli and Klebsiella aerogenes: studies with the continuous-culture technique. J Bacteriol. 1975 Aug;123(2):407–418. doi: 10.1128/jb.123.2.407-418.1975. [DOI] [PMC free article] [PubMed] [Google Scholar]
- Taylor S. I., Mukherjee C., Jungas R. L. Regulation of pyruvate dehydrogenase in isolated rat liver mitochondria. Effects of octanoate, oxidation-reduction state, and adenosine triphosphate to adenosine diphosphate ratio. J Biol Chem. 1975 Mar 25;250(6):2028–2035. [PubMed] [Google Scholar]
- Wieland O. H., Portenhauser R. Regulation of pyruvate-dehydrogenase interconversion in rat-liver mitochondria as related to the phosphorylation state of intramitochondrial adenine nucleotides. Eur J Biochem. 1974 Jun 15;45(2):577–588. doi: 10.1111/j.1432-1033.1974.tb03584.x. [DOI] [PubMed] [Google Scholar]