Abstract
Uptake studies of simple sugars were performed on a membrane fractions containing osmotically active vesicles prepared from normal human kidney cortex. The uptake of D-glucose was found to be sodium-dependent and phlorizin-sensitive. The specificity of the D-glucose transport mechanism is such that it is shared by alpha-methyl-D-glucoside, D-galactose, and 5-thio-D-glucose, while 2-deoxy-D-glucose, 3-O-methyl-D-glucose, D-mannose, and D-fructose show little, if any, affinity. Measurement of the sodium-dependent component of the initial D-glucose uptake as a function of glucose concentration resulted in a curvilinear Scatchard plot, indicating the possibility of cooperative effects, or alternatively, the existence of two (or more) sodium-dependent D-glucose transporters. In the case of two transporters, we estimate that Km congruent to 0.3 mM and Vmax congruent to 2.5 nmol/min per mg of protein for the "high-affinity transporter," and Km approximately 6 mM and Vmax approximately 8 nmol/min per mg of protein for the "low-affinity transporter." These specificity and kinetic properties strongly suggest that the sodium-dependent D-glucose transport mechanism characterized in our studies is localized to the brush border of the proximal tubule.
Full text
PDF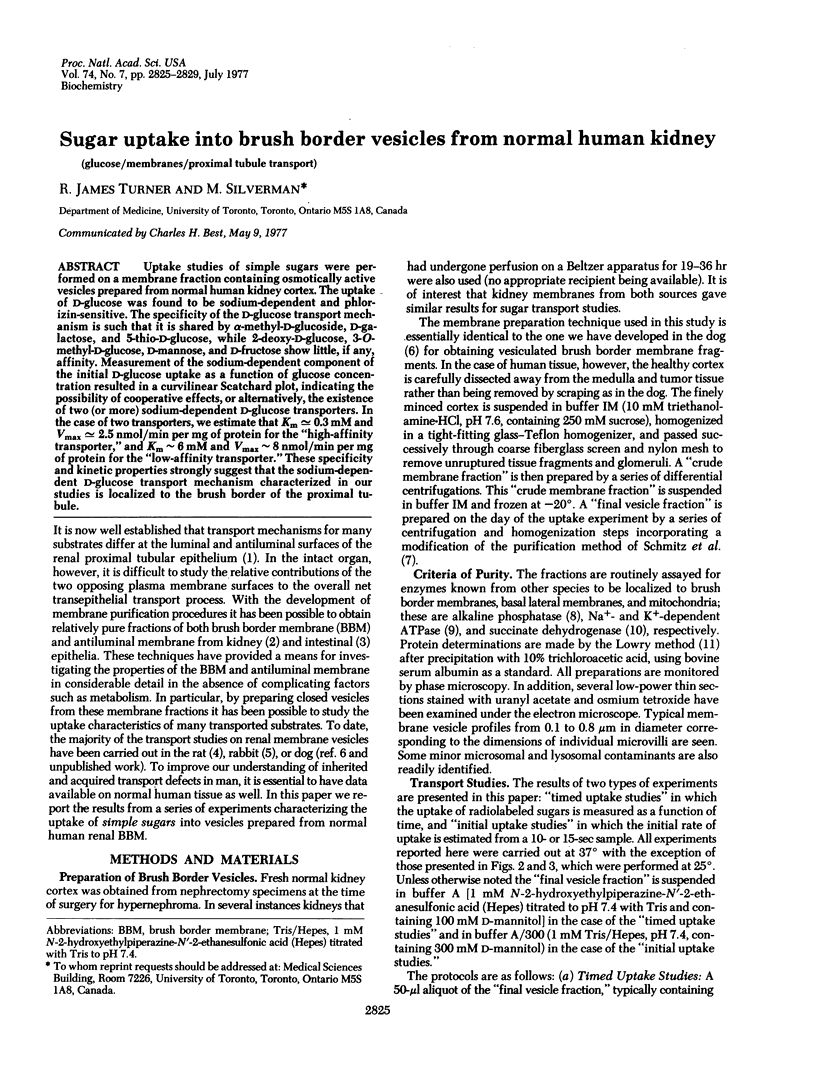
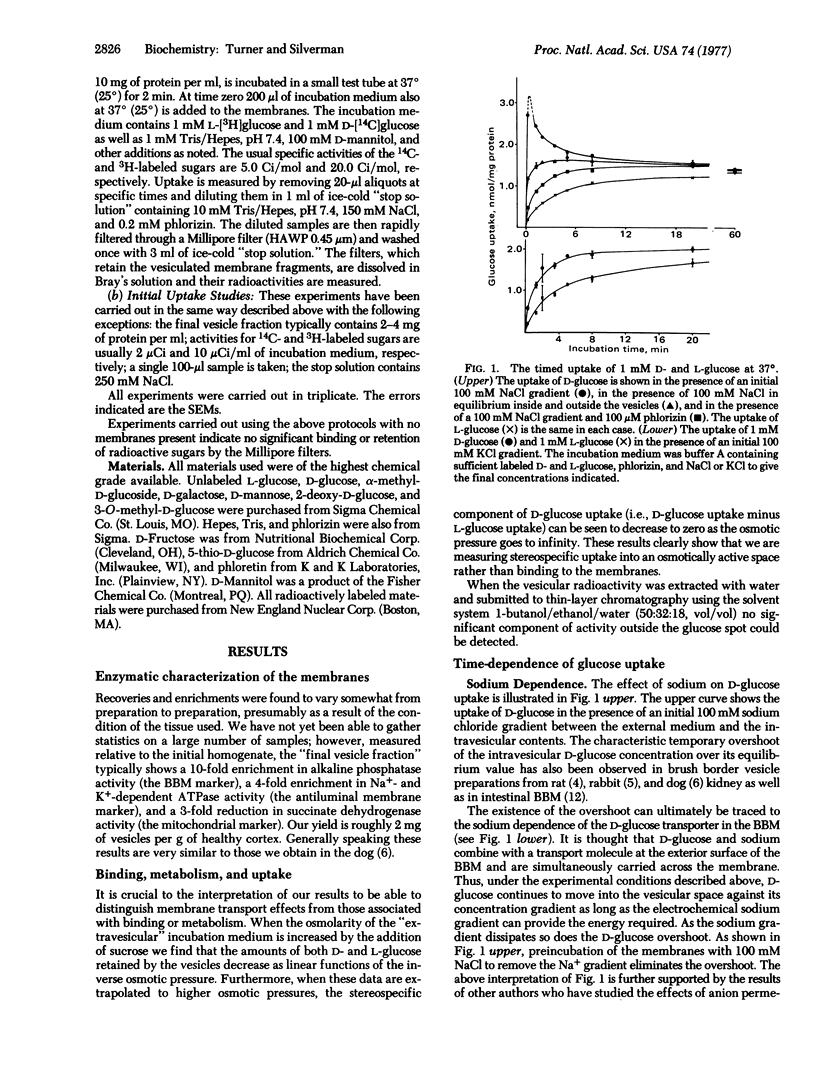
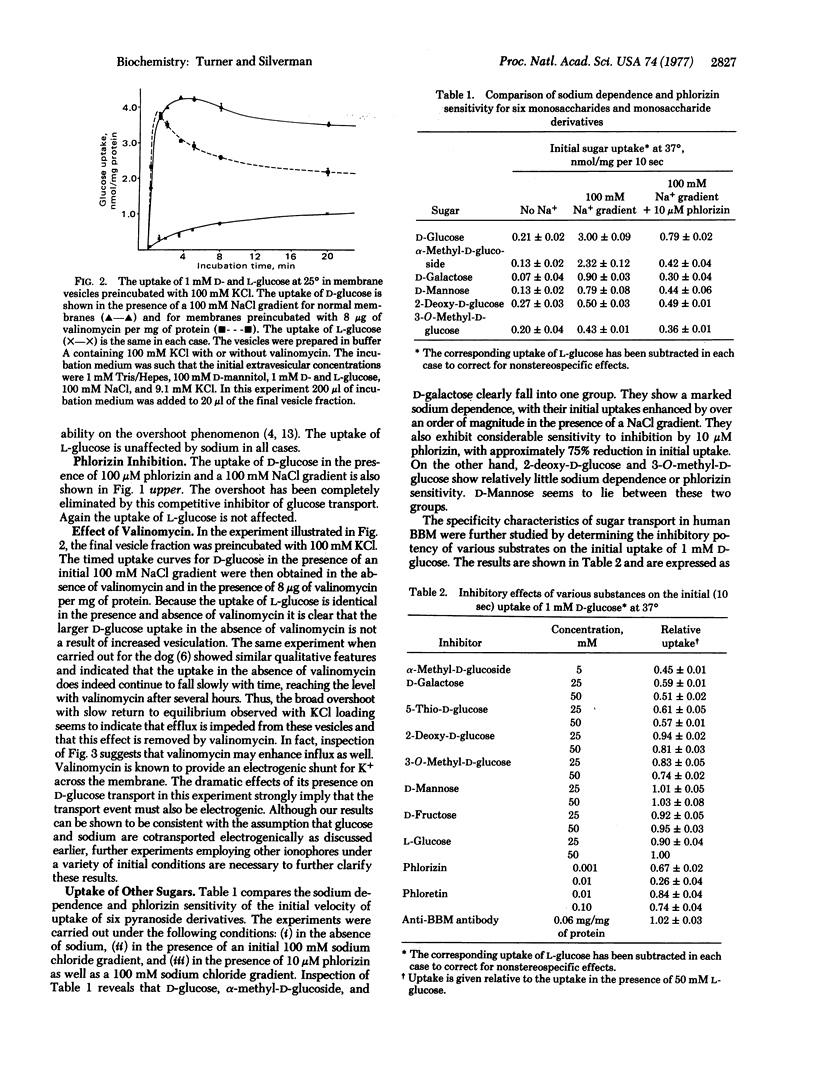
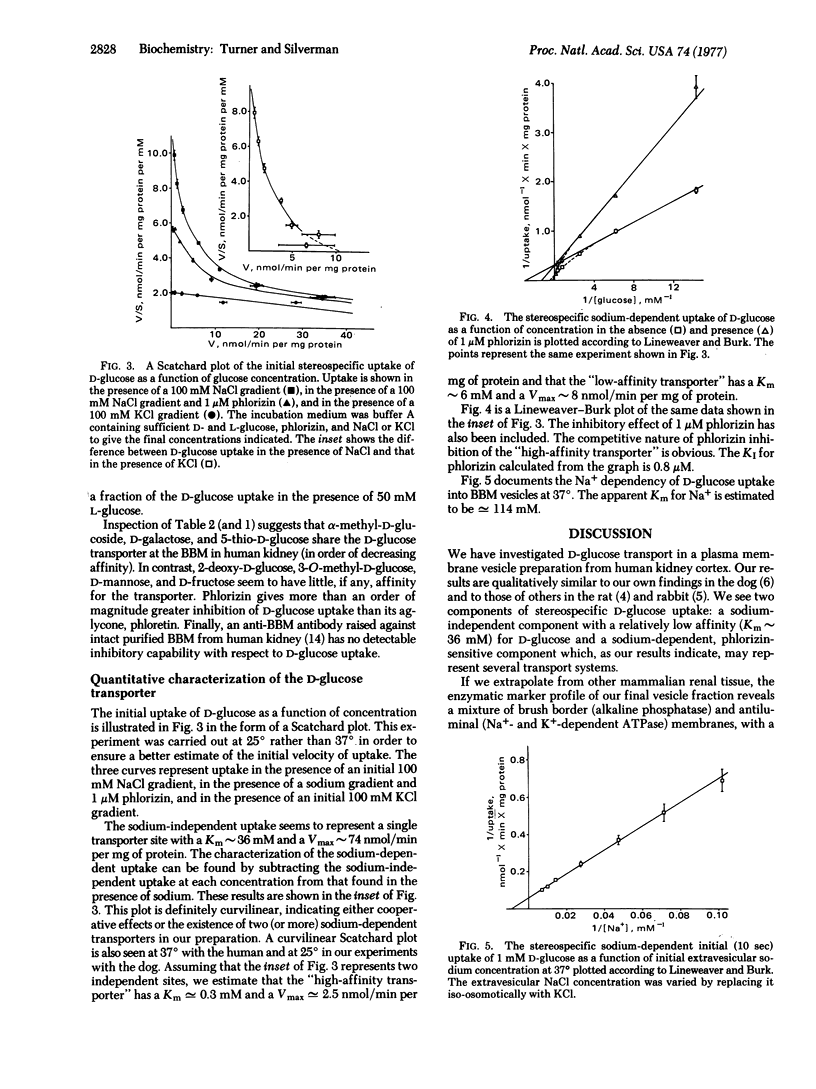
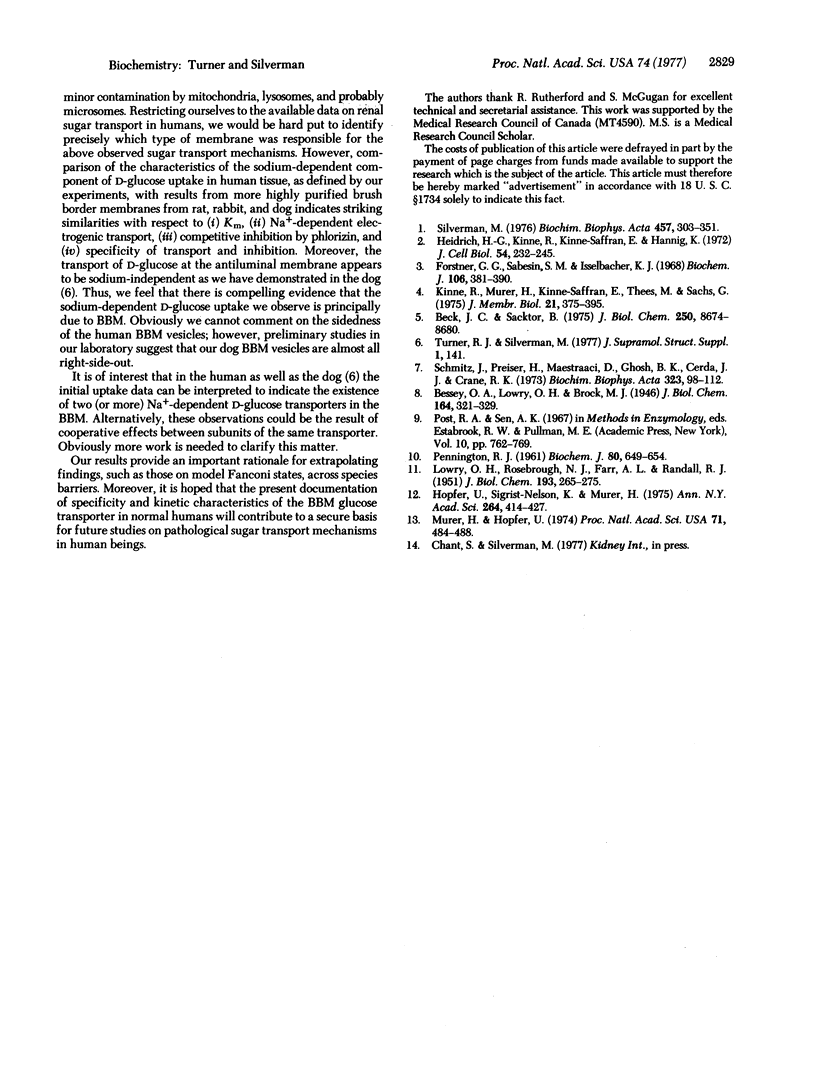
Selected References
These references are in PubMed. This may not be the complete list of references from this article.
- Beck J. C., Sacktor B. Energetics of the Na+-dependent transport of D-glucose in renal brush border membrane vesicles. J Biol Chem. 1975 Nov 25;250(22):8674–8680. [PubMed] [Google Scholar]
- Forstner G. G., Sabesin S. M., Isselbacher K. J. Rat intestinal microvillus membranes. Purification and biochemical characterization. Biochem J. 1968 Jan;106(2):381–390. doi: 10.1042/bj1060381. [DOI] [PMC free article] [PubMed] [Google Scholar]
- Heidrich H. G., Kinne R., Kinne-Saffran E., Hannig K. The polarity of the proximal tubule cell in rat kidney. Different surface charges for the brush-border microvilli and plasma membranes from the basal infoldings. J Cell Biol. 1972 Aug;54(2):232–245. doi: 10.1083/jcb.54.2.232. [DOI] [PMC free article] [PubMed] [Google Scholar]
- Hopfer U. INtestinal sugar transport: studies with isolated plasma membranes. Ann N Y Acad Sci. 1975 Dec 30;264:414–427. doi: 10.1111/j.1749-6632.1975.tb31500.x. [DOI] [PubMed] [Google Scholar]
- LOWRY O. H., ROSEBROUGH N. J., FARR A. L., RANDALL R. J. Protein measurement with the Folin phenol reagent. J Biol Chem. 1951 Nov;193(1):265–275. [PubMed] [Google Scholar]
- Murer H., Hopfer U. Demonstration of electrogenic Na+-dependent D-glucose transport in intestinal brush border membranes. Proc Natl Acad Sci U S A. 1974 Feb;71(2):484–488. doi: 10.1073/pnas.71.2.484. [DOI] [PMC free article] [PubMed] [Google Scholar]
- PENNINGTON R. J. Biochemistry of dystrophic muscle. Mitochondrial succinate-tetrazolium reductase and adenosine triphosphatase. Biochem J. 1961 Sep;80:649–654. doi: 10.1042/bj0800649. [DOI] [PMC free article] [PubMed] [Google Scholar]
- Schmitz J., Preiser H., Maestracci D., Ghosh B. K., Cerda J. J., Crane R. K. Purification of the human intestinal brush border membrane. Biochim Biophys Acta. 1973 Sep 27;323(1):98–112. doi: 10.1016/0005-2736(73)90434-3. [DOI] [PubMed] [Google Scholar]
- Silverman M. Glucose transport in the kidney. Biochim Biophys Acta. 1976 Dec 14;457(3-4):303–351. doi: 10.1016/0304-4157(76)90003-4. [DOI] [PubMed] [Google Scholar]