Abstract
Picolinic acid reversibly inhibits the growth of cultured cells. Fourteen other pyridine derivatives were ineffective or toxic. Untransformed normal rat kidney (NRK) cells are reversibly arrested in the G1 stage of the growth cycle as shown by cell counts, mitotic index, [3H]thymidine incorporation, and flow microfluorometry. Flow microfluorometry was used to monitor the effects of picolinic acid on numerous other cell lines. Normal cells are blocked in G1, whereas transformed cells show responses that are dependent upon the transforming virus and independent of species or origin of the cell line. Kirsten sarcoma virus-transformed cells are blocked in G1. Simian virus 40-transformed cells progress to a G2 block. Cells transformed by polyoma or Harvey sarcoma virus with Moloney virus coat have flow microfluorometry profiles that indicate blocks in both G1 and G2. Cells transformed with Moloney sarcoma virus are not blocked in a specific phase of the cell cycle. Picolinic acid does not change the levels of NAD+ plus NADH; however, the growth inhibition by picolinic acid is partially overcome by nicotinamide. These results suggest that picolinic acid interacts with a specific growth control mechanism that may involve NAD+ and that this control mechanism is altered by different transforming viruses in different manners.
Keywords: cell synchrony, chelating agents, NAD+, pyridine derivatives, viral transformation
Full text
PDF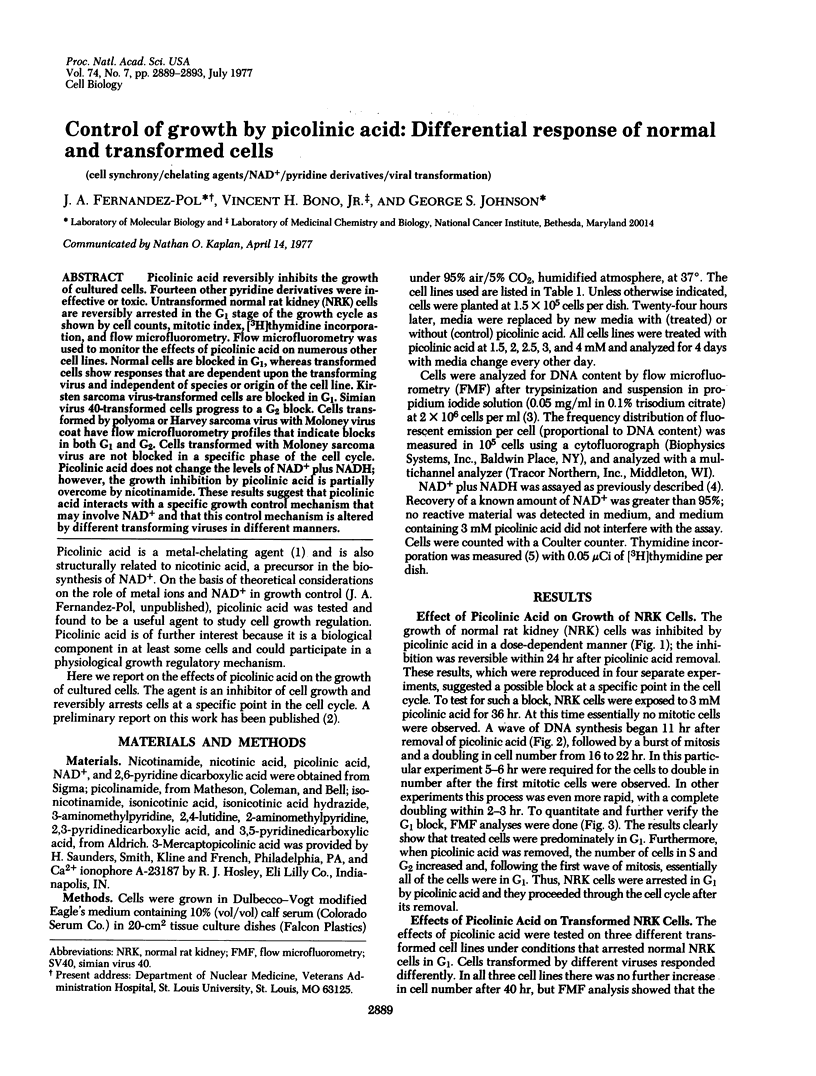
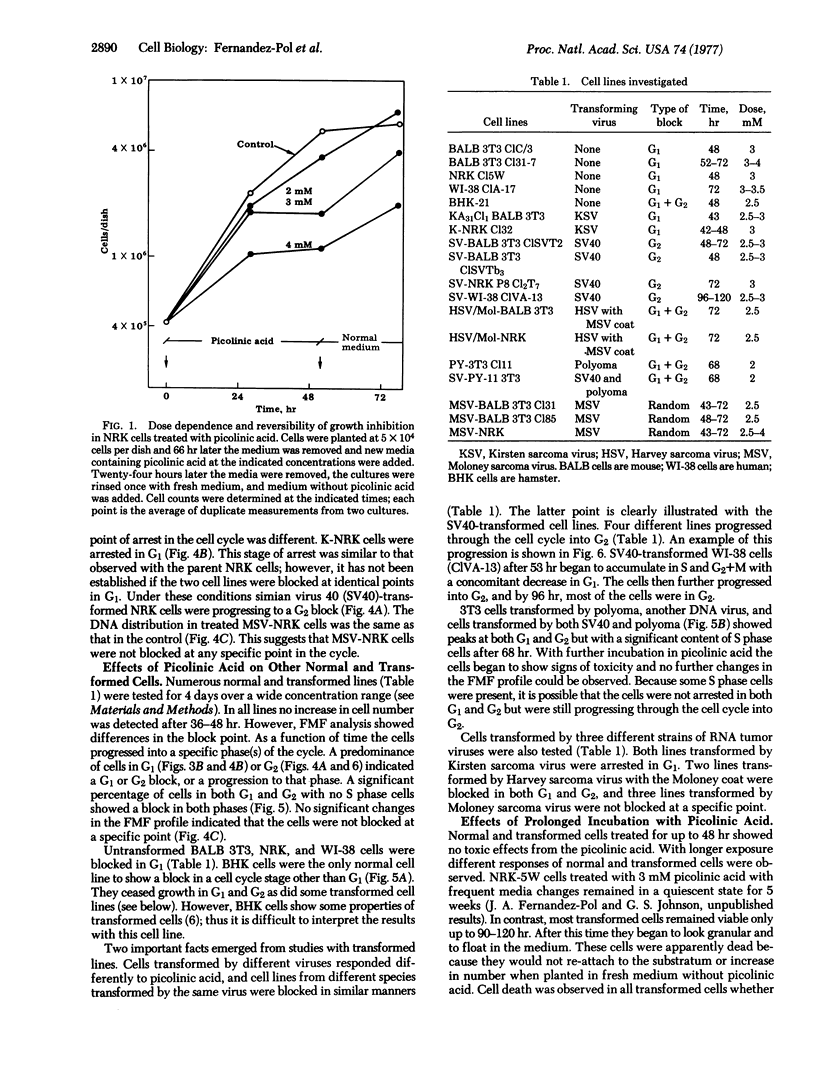
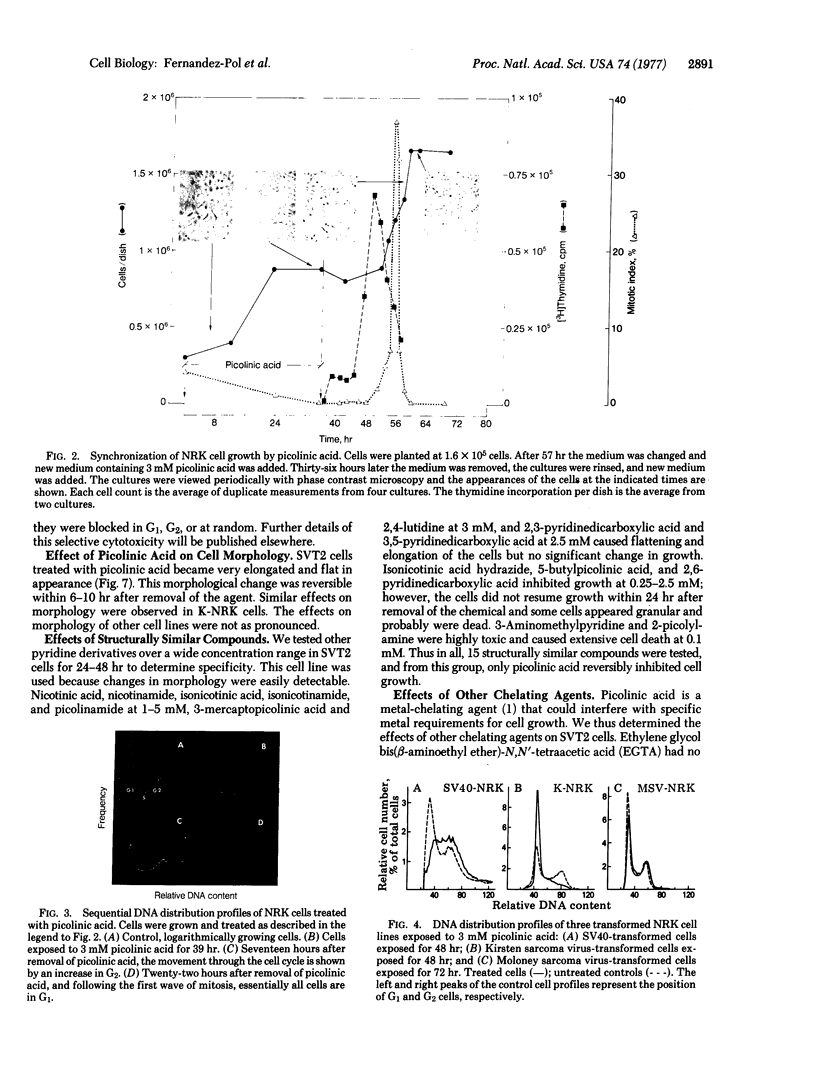
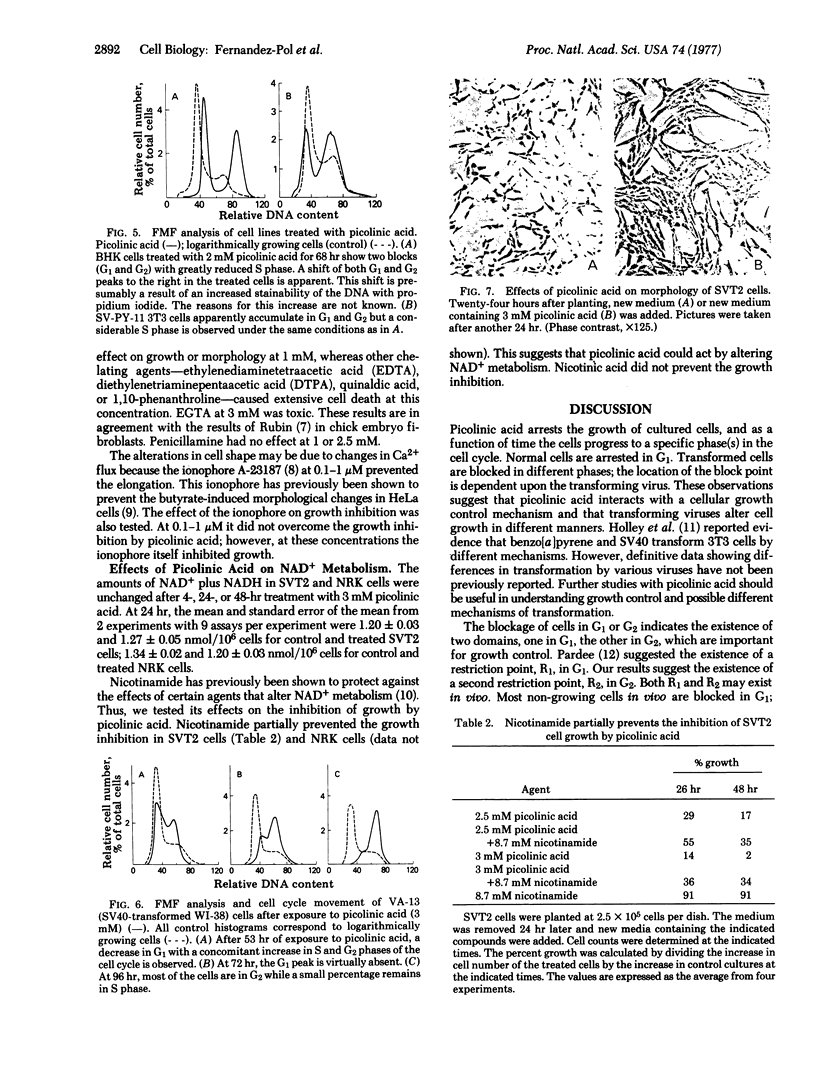
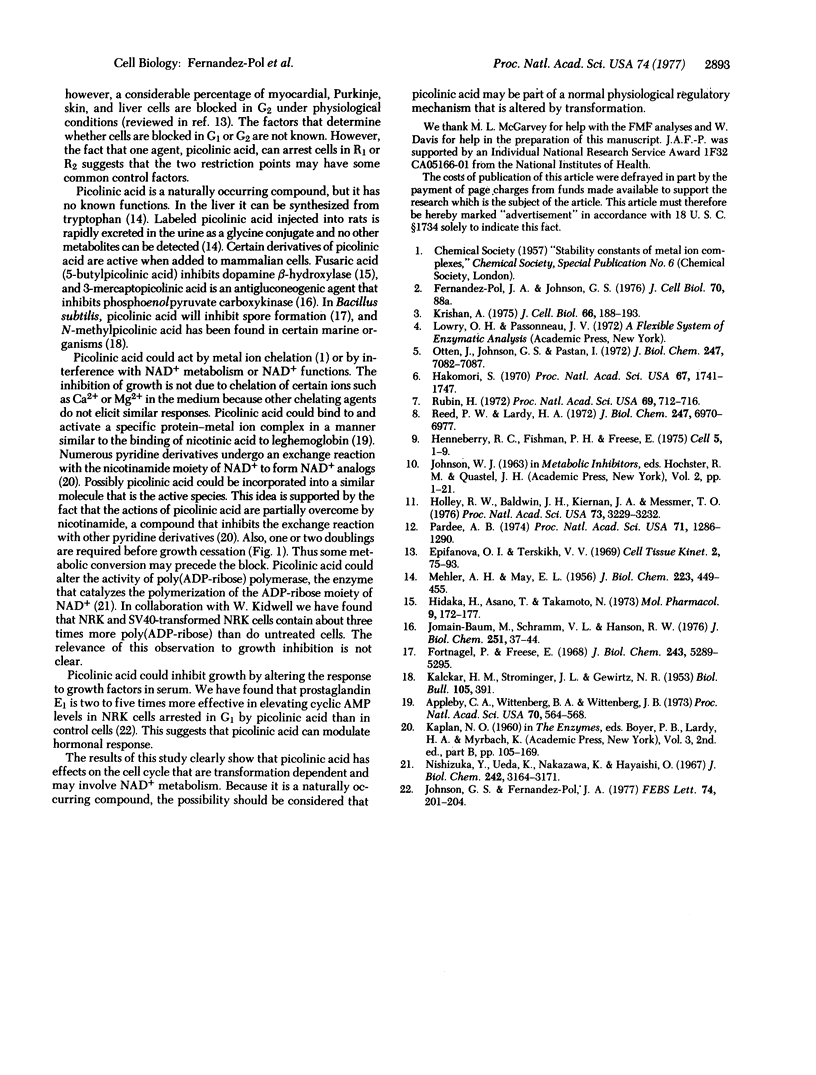
Images in this article
Selected References
These references are in PubMed. This may not be the complete list of references from this article.
- Appleby C. A., Wittenberg B. A., Wittenberg J. B. Nicotinic Acid as a ligand affecting leghemoglobin structure and oxygen reactivity. Proc Natl Acad Sci U S A. 1973 Feb;70(2):564–568. doi: 10.1073/pnas.70.2.564. [DOI] [PMC free article] [PubMed] [Google Scholar]
- Fortnagel P., Freese E. Inhibition of aconitase by chelation of transition metals causing inhibition of sporulation in Bacillus subtilis. J Biol Chem. 1968 Oct 25;243(20):5289–5295. [PubMed] [Google Scholar]
- Hakomori S. Cell density-dependent changes of glycolipid concentrations in fibroblasts, and loss of this response in virus-transformed cells. Proc Natl Acad Sci U S A. 1970 Dec;67(4):1741–1747. doi: 10.1073/pnas.67.4.1741. [DOI] [PMC free article] [PubMed] [Google Scholar]
- Henneberry R. C., Fishman P. H., Freese E. Morphological changes in cultured mammalian cells: prevention by the calcium ionophore A23187. Cell. 1975 May;5(1):1–9. doi: 10.1016/0092-8674(75)90085-9. [DOI] [PubMed] [Google Scholar]
- Hidaka H., Asano T., Takemoto N. Analogues of fusaric (5-butylpicolinic) acid as potent inhibitors of dopamine -hydroxylase. Mol Pharmacol. 1973 Mar;9(2):172–177. [PubMed] [Google Scholar]
- Holley R. W., Baldwin J. H., Kiernan J. A., Messmer T. O. Control of growth of benzo(a)pyrene-transformed 3T3 cells. Proc Natl Acad Sci U S A. 1976 Sep;73(9):3229–3232. doi: 10.1073/pnas.73.9.3229. [DOI] [PMC free article] [PubMed] [Google Scholar]
- Johnson G. S., Fernandez-Pol J. A. NRK cells synchronized in G1 by picolinic acid are super-sensitive to prostaglandin E1 stimulation. FEBS Lett. 1977 Mar 1;74(2):201–204. doi: 10.1016/0014-5793(77)80846-6. [DOI] [PubMed] [Google Scholar]
- Jomain-Baum M., Schramm V. L., Hanson R. W. Mechanism of 3-mercaptopicolinic acid inhibition of hepatic phosphoenolpyruvate carboxykinase (GTP). J Biol Chem. 1976 Jan 10;251(1):37–44. [PubMed] [Google Scholar]
- Krishan A. Rapid flow cytofluorometric analysis of mammalian cell cycle by propidium iodide staining. J Cell Biol. 1975 Jul;66(1):188–193. doi: 10.1083/jcb.66.1.188. [DOI] [PMC free article] [PubMed] [Google Scholar]
- MAY E. L., MEHLER A. H. Studies with carboxyl-labeled 3-hydroxyanthranilic and picolinic acids in vivo and in vitro. J Biol Chem. 1956 Nov;223(1):449–455. [PubMed] [Google Scholar]
- Nishizuka Y., Ueda K., Nakazawa K., Hayaishi O. Studies on the polymer of adenosine diphosphate ribose. I. Enzymic formation from nicotinamide adenine dinuclotide in mammalian nuclei. J Biol Chem. 1967 Jul 10;242(13):3164–3171. [PubMed] [Google Scholar]
- Otten J., Johnson G. S., Pastan I. Regulation of cell growth by cyclic adenosine 3',5'-monophosphate. Effect of cell density and agents which alter cell growth on cyclic adenosine 3',5'-monophosphate levels in fibroblasts. J Biol Chem. 1972 Nov 10;247(21):7082–7087. [PubMed] [Google Scholar]
- Pardee A. B. A restriction point for control of normal animal cell proliferation. Proc Natl Acad Sci U S A. 1974 Apr;71(4):1286–1290. doi: 10.1073/pnas.71.4.1286. [DOI] [PMC free article] [PubMed] [Google Scholar]
- Reed P. W., Lardy H. A. A23187: a divalent cation ionophore. J Biol Chem. 1972 Nov 10;247(21):6970–6977. [PubMed] [Google Scholar]
- Rubin H. Inhibition of DNA synthesis in animal cells by ethylene diamine tetraacetate, and its reversal by zinc. Proc Natl Acad Sci U S A. 1972 Mar;69(3):712–716. doi: 10.1073/pnas.69.3.712. [DOI] [PMC free article] [PubMed] [Google Scholar]