Abstract
The cooperativity and feedback inhibition exhibited by the regulatory enzyme, aspartate transcarbamoylase (carbamoylphosphate: L-aspartate carbamoyltransferase; EC 2.1.3.2), from Escherichia coli are generally attributed to ligand-promoted conformational changes involving alterations in the subunit interactions. However, no quantitative estimates have been made of the effect of ligands on the strength of the intersubunit bonding domains. The native enzyme, composed of two catalytic trimers “crosslinked” by three regulatory dimers, shows little tendency to dissociate in neutral buffers at room temperature. In addition, very little exchange was observed in 2 hr between subunits within the intact enzyme and free subunits. Although exchange was enhanced in solutions of low ionic strength containing the bisubstrate analog, N-(phosphonacetyl)-L-aspartate, the rates of exchange were too small to permit reliable estimates of the weakening of the bonding domains caused by the ligand. Studies were conducted, therefore, on a less stable oligomeric complex which resembles the native enzyme in structure and allosteric behavior but lacks one regulatory subunit. These molecules, containing only four bonding domains between the catalytic and regulatory polypeptide chains (compared to six in the native enzyme), disproportionate to form the more stable native enzyme and free catalytic subunits. An electrophoretic technique is described for measuring the rate of disproportionation which is controlled by the rupture of the intersubunit bonding domains. This rate is enhanced about 300-fold upon the addition of the active-site ligand. Hence the ligand-promoted allosteric conversion of the enzyme-like complex from the constrained to the relaxed conformation involves a substantial weakening of the intersubunit interactions corresponding to about 1.7 kcal/mole (7.1 kJ/mole) per bonding domain between a catalytic and a regulatory chain.
Keywords: protein-protein interactions, conformational changes, allosteric enzymes, kinetics of assembly
Full text
PDF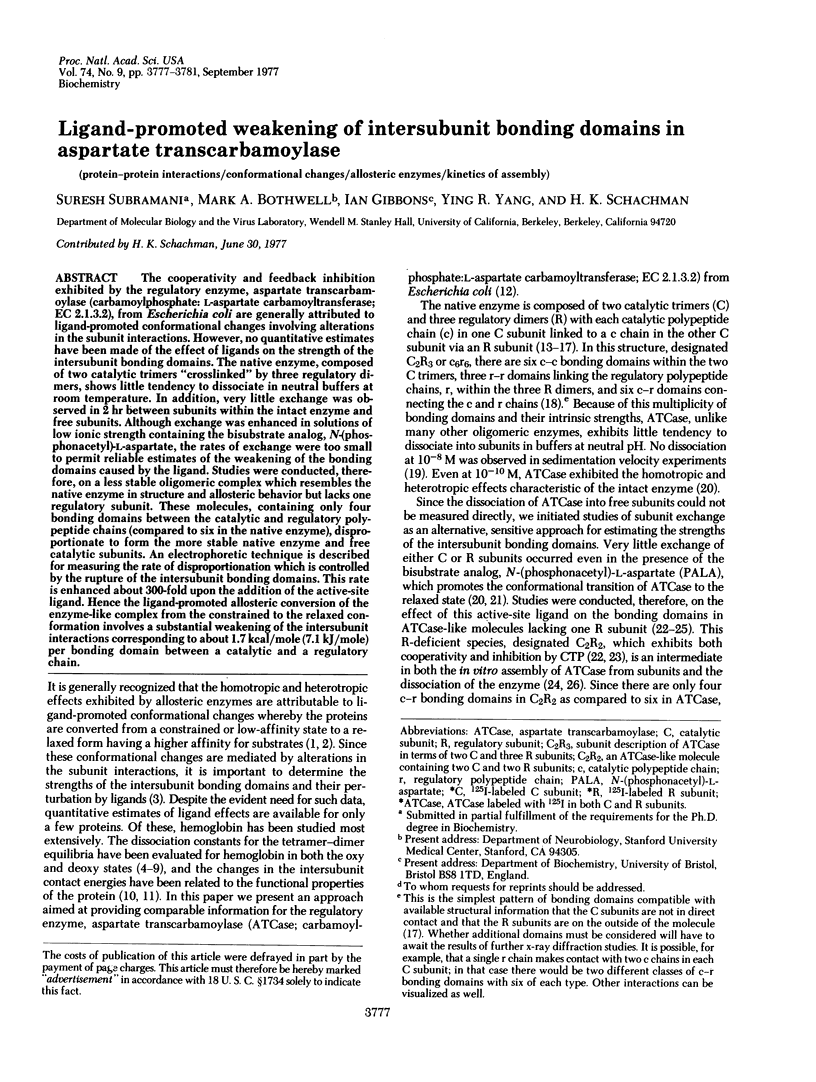
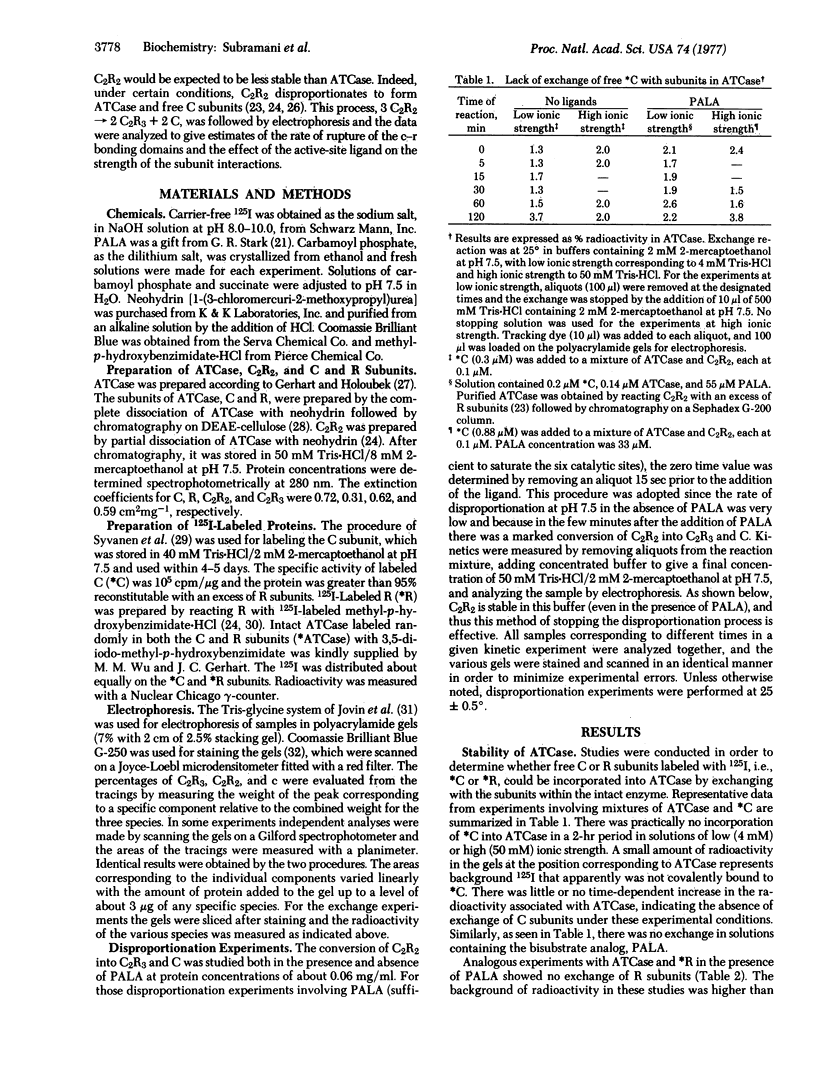
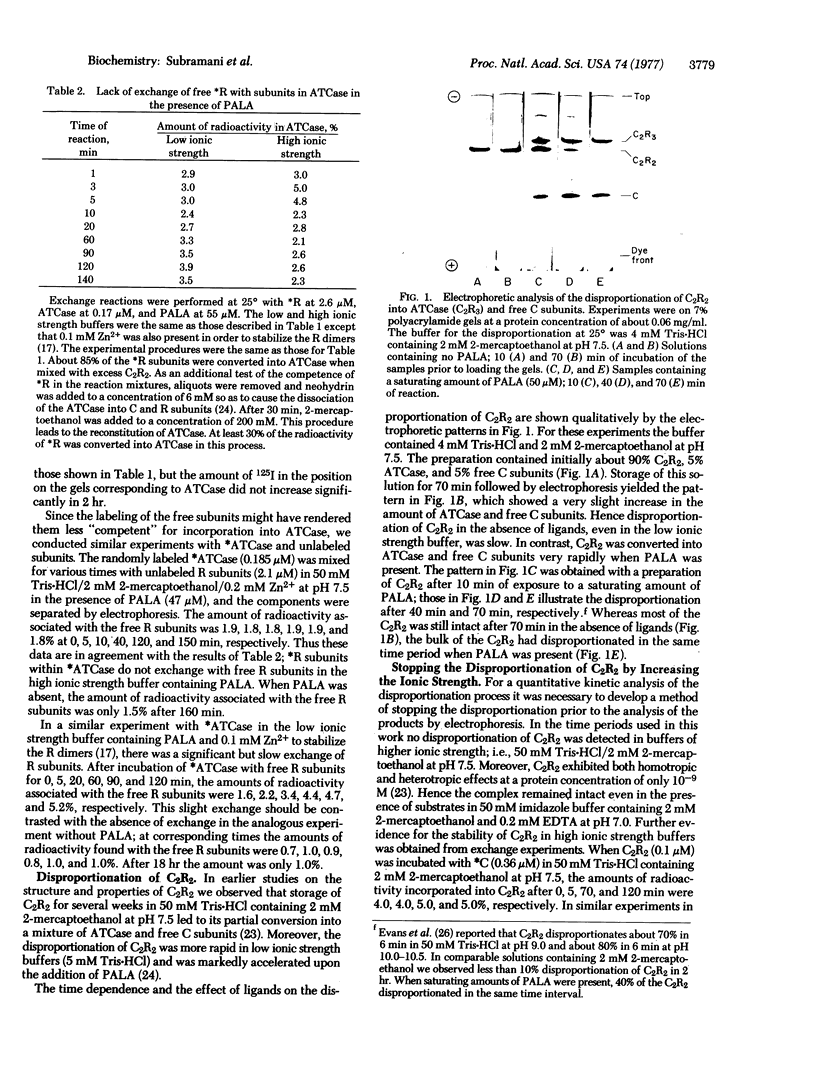
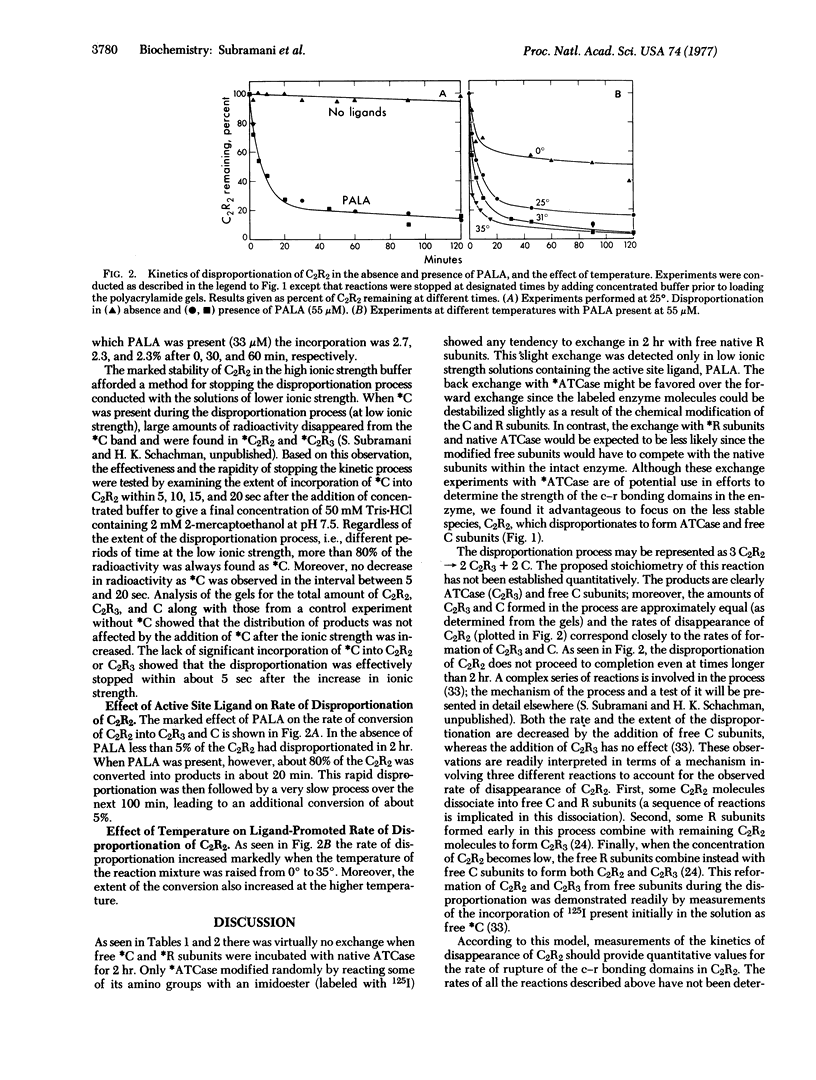
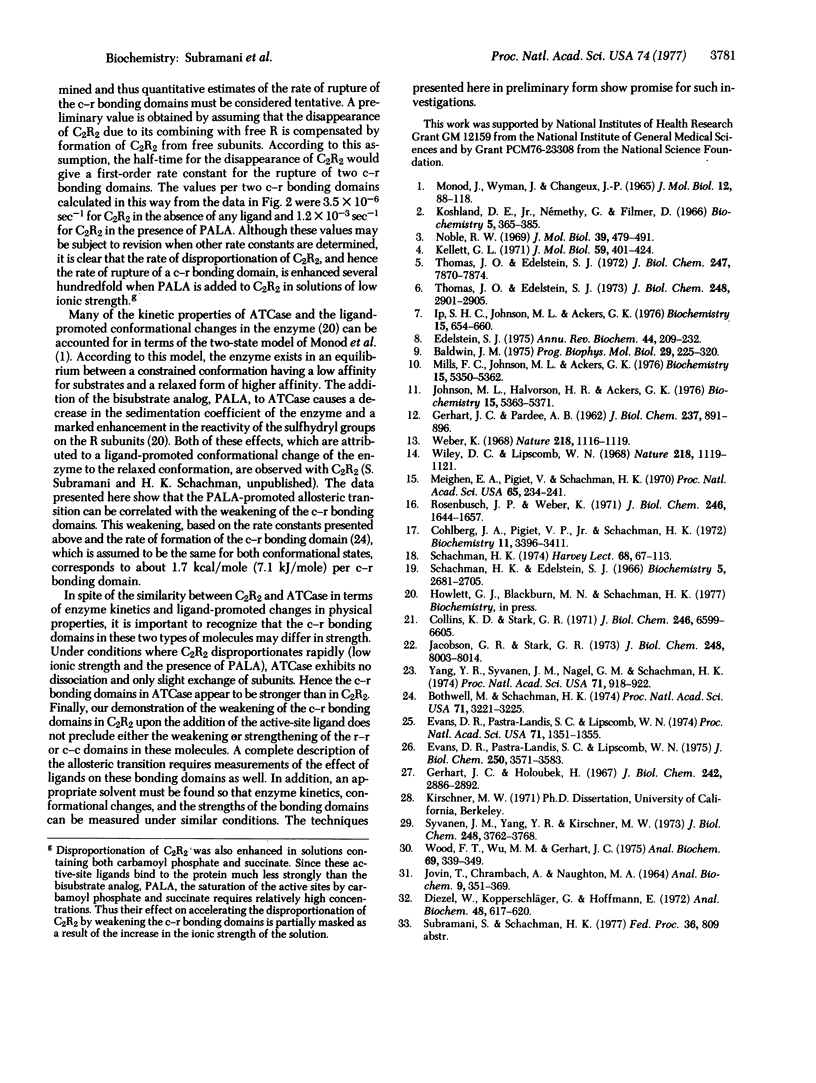
Images in this article
Selected References
These references are in PubMed. This may not be the complete list of references from this article.
- Baldwin J. M. Structure and function of haemoglobin. Prog Biophys Mol Biol. 1975;29(3):225–320. doi: 10.1016/0079-6107(76)90024-9. [DOI] [PubMed] [Google Scholar]
- Bothwell M., Schachman H. K. Pathways of assembly of aspartate transcarbamoylase from catalytic and regulatory subunits. Proc Natl Acad Sci U S A. 1974 Aug;71(8):3221–3225. doi: 10.1073/pnas.71.8.3221. [DOI] [PMC free article] [PubMed] [Google Scholar]
- Cohlberg J. A., Pigiet V. P., Jr, Schachman H. K. Structure and arrangement of the regulatory subunits in aspartate transcarbamylase. Biochemistry. 1972 Aug 29;11(18):3396–3411. doi: 10.1021/bi00768a013. [DOI] [PubMed] [Google Scholar]
- Collins K. D., Stark G. R. Aspartate transcarbamylase. Interaction with the transition state analogue N-(phosphonacetyl)-L-aspartate. J Biol Chem. 1971 Nov;246(21):6599–6605. [PubMed] [Google Scholar]
- Diezel W., Kopperschläger G., Hofmann E. An improved procedure for protein staining in polyacrylamide gels with a new type of Coomassie Brilliant Blue. Anal Biochem. 1972 Aug;48(2):617–620. doi: 10.1016/0003-2697(72)90117-0. [DOI] [PubMed] [Google Scholar]
- Edelstein S. J. Cooperative interactions of hemoglobin. Annu Rev Biochem. 1975;44:209–232. doi: 10.1146/annurev.bi.44.070175.001233. [DOI] [PubMed] [Google Scholar]
- Evans D. R., Pastra-Landis S. C., Lipscomb W. N. An intermediate complex in the dissociation of aspartate transcarbamylase. Proc Natl Acad Sci U S A. 1974 Apr;71(4):1351–1355. doi: 10.1073/pnas.71.4.1351. [DOI] [PMC free article] [PubMed] [Google Scholar]
- Evans D. R., Pastra-Landis S. C., Lipscomb W. N. Isolation and properties of a species produced by the partial dissociation of aspartate transcarbamylase from Escherichia coli. J Biol Chem. 1975 May 25;250(10):3571–3583. [PubMed] [Google Scholar]
- GERHART J. C., PARDEE A. B. The enzymology of control by feedback inhibition. J Biol Chem. 1962 Mar;237:891–896. [PubMed] [Google Scholar]
- Gerhart J. C., Holoubek H. The purification of aspartate transcarbamylase of Escherichia coli and separation of its protein subunits. J Biol Chem. 1967 Jun 25;242(12):2886–2892. [PubMed] [Google Scholar]
- Ip S. H., Johnson M. L., Ackers G. K. Kinetics of deoxyhemoglobin subunit dissociation determined by haptoglobin binding: estimation of the equilibrium constant from forward and reverse rates. Biochemistry. 1976 Feb 10;15(3):654–660. doi: 10.1021/bi00648a032. [DOI] [PubMed] [Google Scholar]
- JOVIN T., CHRAMBACH A., NAUGHTON M. A. AN APPARATUS FOR PREPARATIVE TEMPERATURE-REGULATED POLYACRYLAMIDE GEL ELECTROPHORESIS. Anal Biochem. 1964 Nov;9:351–369. doi: 10.1016/0003-2697(64)90192-7. [DOI] [PubMed] [Google Scholar]
- Jacobson G. R., Stark G. R. Aspartate transcarbamylase. A study of possible roles for the sulfhydryl group at the active site. J Biol Chem. 1973 Dec 10;248(23):8003–8014. [PubMed] [Google Scholar]
- Johnson M. L., Halvorson H. R., Ackers G. K. Oxygenation-linked subunit interactions in human hemoglobin: analysis of linkage functions for constituent energy terms. Biochemistry. 1976 Nov 30;15(24):5363–5371. doi: 10.1021/bi00669a024. [DOI] [PubMed] [Google Scholar]
- Kellett G. L. Dissociation of hemoglobin into subunits. Ligand-linked dissociation at neutral pH. J Mol Biol. 1971 Aug 14;59(3):401–424. doi: 10.1016/0022-2836(71)90307-x. [DOI] [PubMed] [Google Scholar]
- Koshland D. E., Jr, Némethy G., Filmer D. Comparison of experimental binding data and theoretical models in proteins containing subunits. Biochemistry. 1966 Jan;5(1):365–385. doi: 10.1021/bi00865a047. [DOI] [PubMed] [Google Scholar]
- MONOD J., WYMAN J., CHANGEUX J. P. ON THE NATURE OF ALLOSTERIC TRANSITIONS: A PLAUSIBLE MODEL. J Mol Biol. 1965 May;12:88–118. doi: 10.1016/s0022-2836(65)80285-6. [DOI] [PubMed] [Google Scholar]
- Meighen E. A., Pigiet V., Schachman H. K. Hybridization of native and chemically modified enzymes. 3. The catalytic subunits of aspartate transcarbamylase. Proc Natl Acad Sci U S A. 1970 Jan;65(1):234–241. doi: 10.1073/pnas.65.1.234. [DOI] [PMC free article] [PubMed] [Google Scholar]
- Mills F. C., Johnson M. L., Ackers G. K. Oxygenation-linked subunit interactions in human hemoglobin: experimental studies on the concentration dependence of oxygenation curves. Biochemistry. 1976 Nov 30;15(24):5350–5362. doi: 10.1021/bi00669a023. [DOI] [PubMed] [Google Scholar]
- Noble R. W. Relation between allosteric effects and changes in the energy of bonding between molecular subunits. J Mol Biol. 1969 Feb 14;39(3):479–491. doi: 10.1016/0022-2836(69)90139-9. [DOI] [PubMed] [Google Scholar]
- Rosenbusch J. P., Weber K. Subunit structure of aspartate transcarbamylase from Escherichia coli. J Biol Chem. 1971 Mar 25;246(6):1644–1657. [PubMed] [Google Scholar]
- Schachman H. K. Anatomy and physiology of a regulatory enzyme-aspartate transcarbamylase. Harvey Lect. 1974;68:67–113. [PubMed] [Google Scholar]
- Schachman H. K., Edelstein S. J. Ultracentrifuge studies with absorption optics. IV. Molecular weight determinations at the microgram level. Biochemistry. 1966 Aug;5(8):2681–2705. doi: 10.1021/bi00872a029. [DOI] [PubMed] [Google Scholar]
- Syvanen J. M., Yang Y. R., Kirschiner M. W. Preparation of 125 I-Catalytic subunit of asparatate transcarbamylase and its use in studies of the regulatory subunit. J Biol Chem. 1973 Jun 10;248(11):3762–3768. [PubMed] [Google Scholar]
- Thomas J. O., Edelstein S. J. Observation of the dissociation of unliganded hemoglobin. II. Effect of pH, salt, and dioxane. J Biol Chem. 1973 Apr 25;248(8):2901–2905. [PubMed] [Google Scholar]
- Thomas J. O., Edelstein S. J. Observation of the dissociation of unliganded hemoglobin. J Biol Chem. 1972 Dec 25;247(24):7870–7874. [PubMed] [Google Scholar]
- Weber K. New structural model of E. coli aspartate transcarbamylase and the amino-acid sequence of the regulatory polypeptide chain. Nature. 1968 Jun 22;218(5147):1116–1119. doi: 10.1038/2181116a0. [DOI] [PubMed] [Google Scholar]
- Wiley D. C., Lipscomb W. N. Crystallographic determination of symmetry of aspartate transcarbamylase. Nature. 1968 Jun 22;218(5147):1119–1121. doi: 10.1038/2181119a0. [DOI] [PubMed] [Google Scholar]
- Wood F. T., Wu M. M., Gerhart J. C. The radioactive labeling of proteins with an iodinated amidination reagent. Anal Biochem. 1975 Dec;69(2):339–349. doi: 10.1016/0003-2697(75)90136-0. [DOI] [PubMed] [Google Scholar]
- Yang Y. R., Syvanen J. M., Nagel G. M., Schachman H. K. Aspartate transcarbamoylase molecules lacking one regulatory subunit. Proc Natl Acad Sci U S A. 1974 Mar;71(3):918–922. doi: 10.1073/pnas.71.3.918. [DOI] [PMC free article] [PubMed] [Google Scholar]