Abstract
Phosphorylation of the myosin regulatory light chains (RLCs) activates contraction in smooth muscle and modulates force production in striated muscle. RLC phosphorylation changes the net charge in a critical region of the N terminus and thereby may alter interactions between the RLC and myosin heavy chain. A series of N-terminal charge mutations in the human smooth muscle RLC has been engineered, and the mutants have been evaluated for their ability to mimic the phosphorylated form of the RLC when reconstituted into scallop striated muscle bundles or into isolated smooth muscle myosin. Changing the net charge in the region from Arg-13 to Ser-19 potentiates force in scallop striated muscle and maintains smooth muscle myosin in an unfolded filamentous state without affecting ATPase activity or motility of smooth muscle myosin. Thus, the effect of RLC phosphorylation in striated muscle and its ability to regulate the folded-to-extended conformational transition in smooth muscle may be due to a simple reduction of net charge at the N terminus of the light chain. The ability of phosphorylation to regulate smooth muscle myosin's ATPase activity and motility involves a more complex mechanism.
Full text
PDF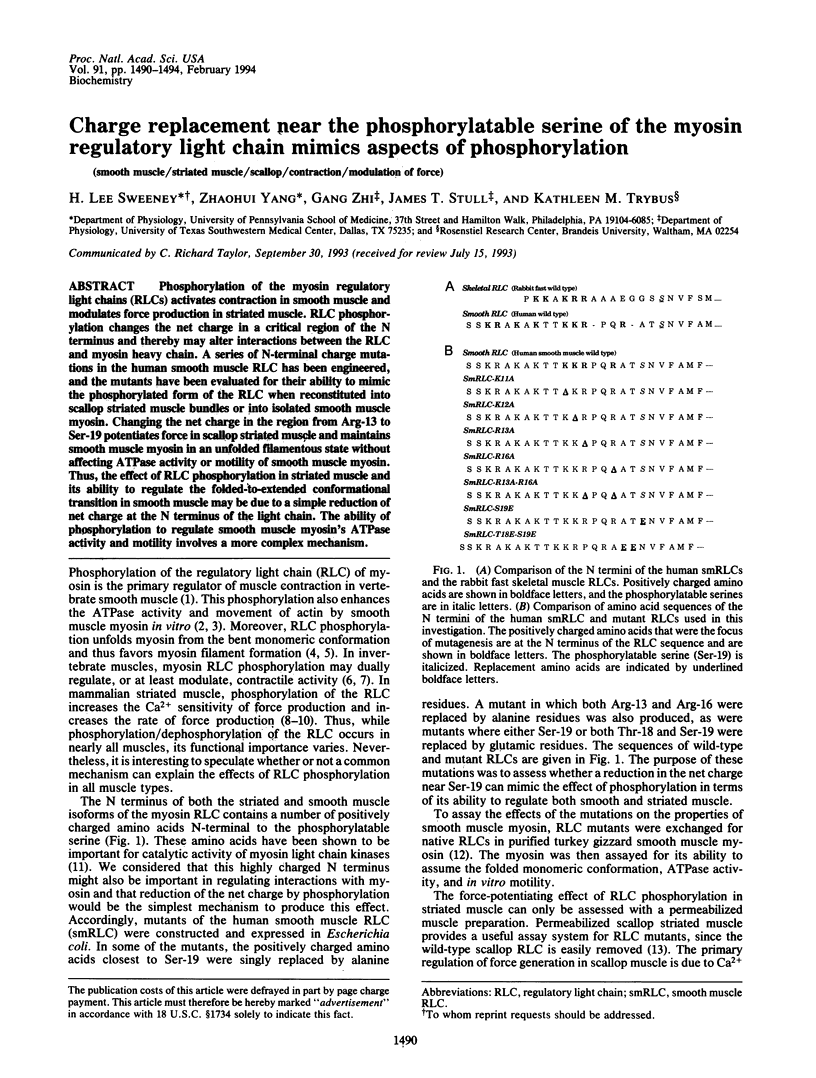
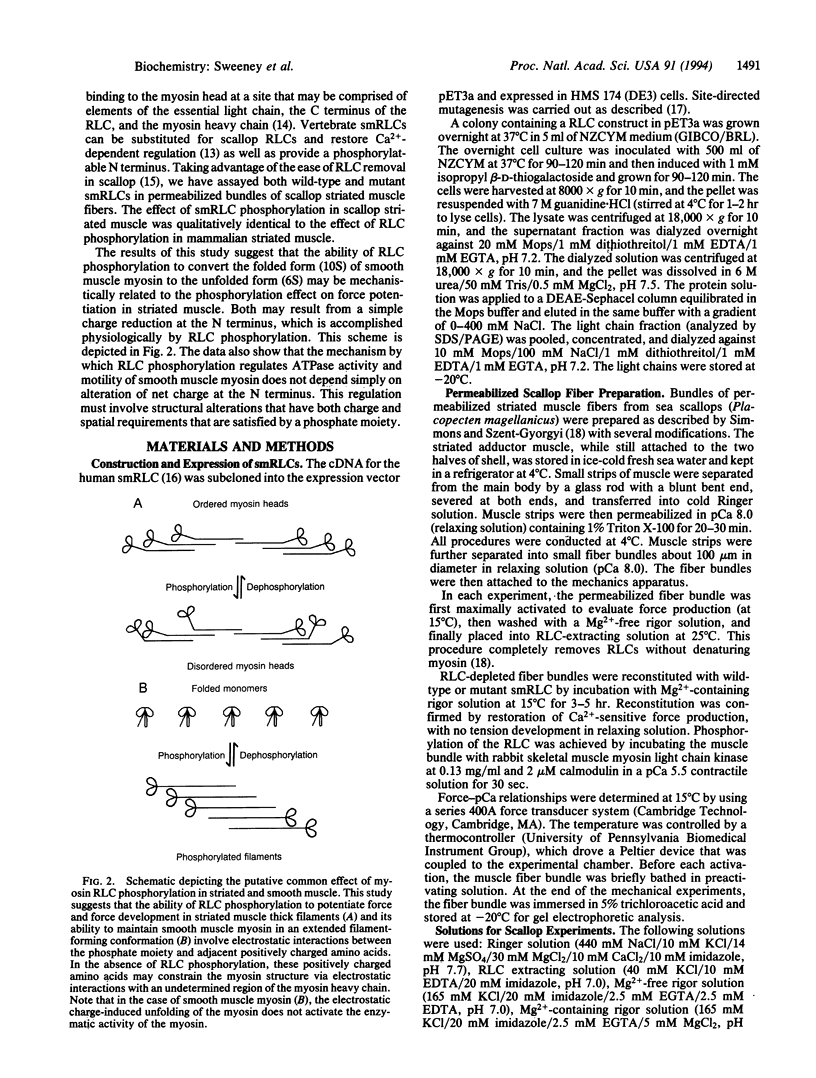
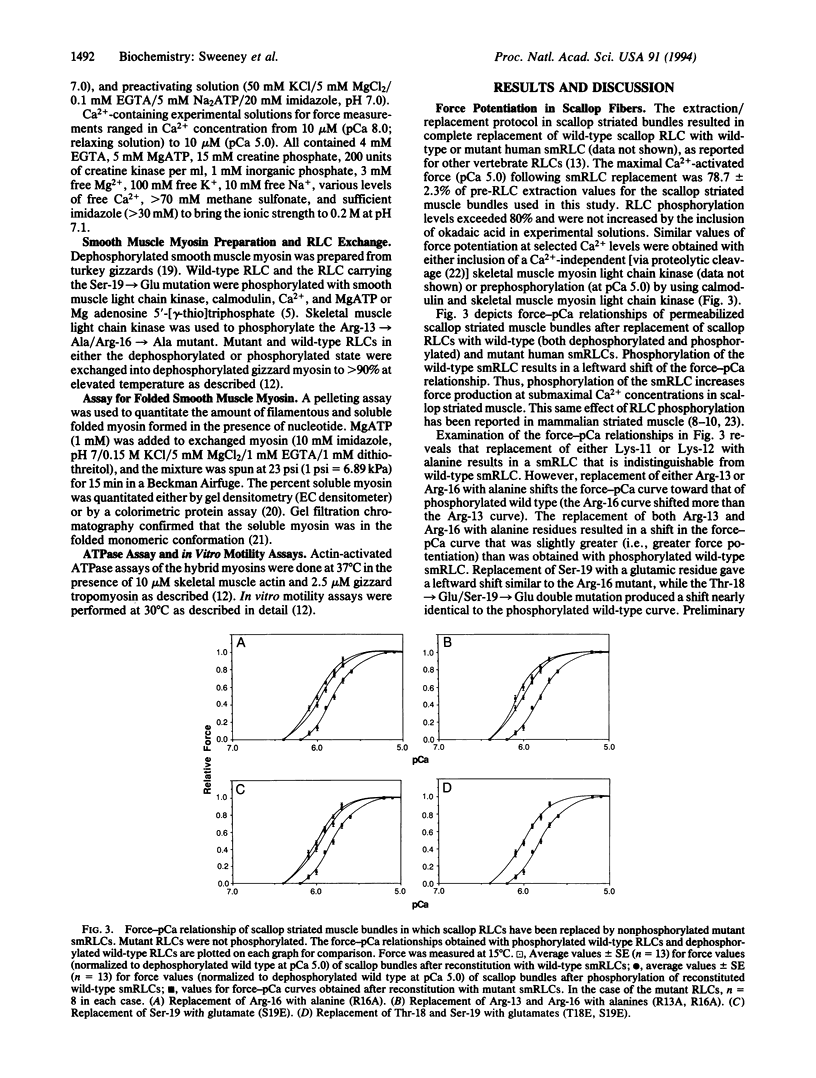
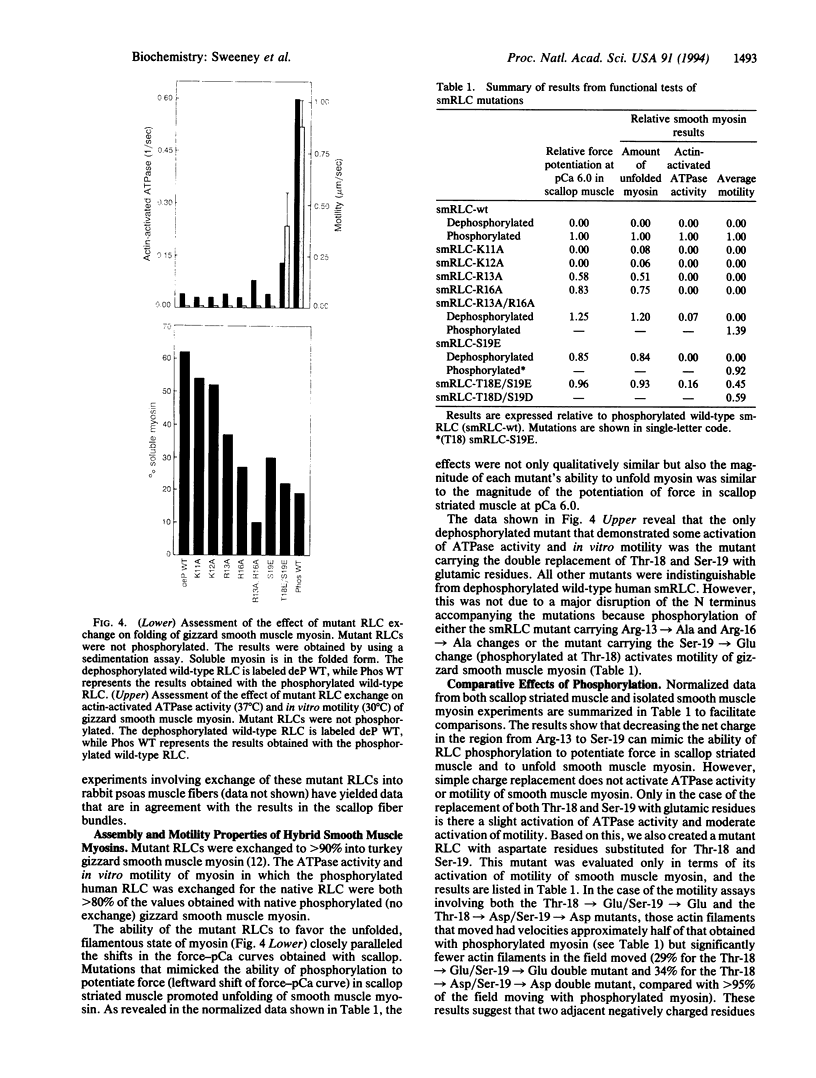
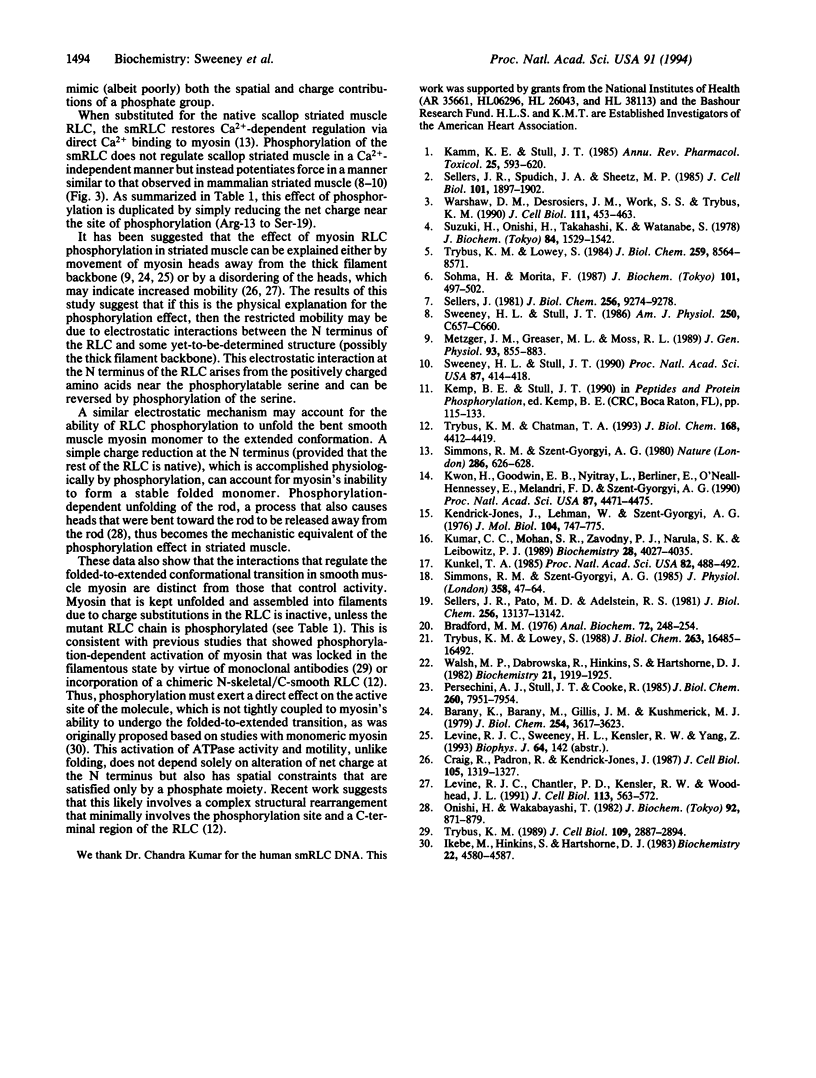
Images in this article
Selected References
These references are in PubMed. This may not be the complete list of references from this article.
- Bradford M. M. A rapid and sensitive method for the quantitation of microgram quantities of protein utilizing the principle of protein-dye binding. Anal Biochem. 1976 May 7;72:248–254. doi: 10.1006/abio.1976.9999. [DOI] [PubMed] [Google Scholar]
- Bárány K., Bárány M., Gillis J. M., Kushmerick M. J. Phosphorylation-dephosphorylation of the 18,000-dalton light chain of myosin during the contraction-relaxation cycle of frog muscle. J Biol Chem. 1979 May 10;254(9):3617–3623. [PubMed] [Google Scholar]
- Craig R., Padrón R., Kendrick-Jones J. Structural changes accompanying phosphorylation of tarantula muscle myosin filaments. J Cell Biol. 1987 Sep;105(3):1319–1327. doi: 10.1083/jcb.105.3.1319. [DOI] [PMC free article] [PubMed] [Google Scholar]
- Ikebe M., Hinkins S., Hartshorne D. J. Correlation of enzymatic properties and conformation of smooth muscle myosin. Biochemistry. 1983 Sep 13;22(19):4580–4587. doi: 10.1021/bi00288a036. [DOI] [PubMed] [Google Scholar]
- Kamm K. E., Stull J. T. The function of myosin and myosin light chain kinase phosphorylation in smooth muscle. Annu Rev Pharmacol Toxicol. 1985;25:593–620. doi: 10.1146/annurev.pa.25.040185.003113. [DOI] [PubMed] [Google Scholar]
- Kendrick-Jones J., Szentkiralyi E. M., Szent-Györgyi A. G. Regulatory light chains in myosins. J Mol Biol. 1976 Jul 15;104(4):747–775. doi: 10.1016/0022-2836(76)90180-7. [DOI] [PubMed] [Google Scholar]
- Kumar C. C., Mohan S. R., Zavodny P. J., Narula S. K., Leibowitz P. J. Characterization and differential expression of human vascular smooth muscle myosin light chain 2 isoform in nonmuscle cells. Biochemistry. 1989 May 2;28(9):4027–4035. doi: 10.1021/bi00435a059. [DOI] [PubMed] [Google Scholar]
- Kunkel T. A. Rapid and efficient site-specific mutagenesis without phenotypic selection. Proc Natl Acad Sci U S A. 1985 Jan;82(2):488–492. doi: 10.1073/pnas.82.2.488. [DOI] [PMC free article] [PubMed] [Google Scholar]
- Levine R. J., Chantler P. D., Kensler R. W., Woodhead J. L. Effects of phosphorylation by myosin light chain kinase on the structure of Limulus thick filaments. J Cell Biol. 1991 May;113(3):563–572. doi: 10.1083/jcb.113.3.563. [DOI] [PMC free article] [PubMed] [Google Scholar]
- Metzger J. M., Greaser M. L., Moss R. L. Variations in cross-bridge attachment rate and tension with phosphorylation of myosin in mammalian skinned skeletal muscle fibers. Implications for twitch potentiation in intact muscle. J Gen Physiol. 1989 May;93(5):855–883. doi: 10.1085/jgp.93.5.855. [DOI] [PMC free article] [PubMed] [Google Scholar]
- Onishi H., Wakabayashi T. Electron microscopic studies of myosin molecules from chicken gizzard muscle I: the formation of the intramolecular loop in the myosin tail. J Biochem. 1982 Sep;92(3):871–879. doi: 10.1093/oxfordjournals.jbchem.a134001. [DOI] [PubMed] [Google Scholar]
- Persechini A., Stull J. T., Cooke R. The effect of myosin phosphorylation on the contractile properties of skinned rabbit skeletal muscle fibers. J Biol Chem. 1985 Jul 5;260(13):7951–7954. [PubMed] [Google Scholar]
- Sellers J. R., Pato M. D., Adelstein R. S. Reversible phosphorylation of smooth muscle myosin, heavy meromyosin, and platelet myosin. J Biol Chem. 1981 Dec 25;256(24):13137–13142. [PubMed] [Google Scholar]
- Sellers J. R. Phosphorylation-dependent regulation of Limulus myosin. J Biol Chem. 1981 Sep 10;256(17):9274–9278. [PubMed] [Google Scholar]
- Sellers J. R., Spudich J. A., Sheetz M. P. Light chain phosphorylation regulates the movement of smooth muscle myosin on actin filaments. J Cell Biol. 1985 Nov;101(5 Pt 1):1897–1902. doi: 10.1083/jcb.101.5.1897. [DOI] [PMC free article] [PubMed] [Google Scholar]
- Simmons R. M., Szent-Györgyi A. G. A mechanical study of regulation in the striated adductor muscle of the scallop. J Physiol. 1985 Jan;358:47–64. doi: 10.1113/jphysiol.1985.sp015539. [DOI] [PMC free article] [PubMed] [Google Scholar]
- Simmons R. M., Szent-Györgyi A. G. Control of tension development in scallop muscle fibres with foreign regulatory light chains. Nature. 1980 Aug 7;286(5773):626–628. doi: 10.1038/286626a0. [DOI] [PubMed] [Google Scholar]
- Sohma H., Morita F. Characterization of regulatory light chain-a myosin kinase from smooth muscle of scallop, Patinopecten yessoensis. J Biochem. 1987 Feb;101(2):497–502. [PubMed] [Google Scholar]
- Suzuki H., Onishi H., Takahashi K., Watanabe S. Structure and function of chicken gizzard myosin. J Biochem. 1978 Dec;84(6):1529–1542. doi: 10.1093/oxfordjournals.jbchem.a132278. [DOI] [PubMed] [Google Scholar]
- Sweeney H. L., Stull J. T. Alteration of cross-bridge kinetics by myosin light chain phosphorylation in rabbit skeletal muscle: implications for regulation of actin-myosin interaction. Proc Natl Acad Sci U S A. 1990 Jan;87(1):414–418. doi: 10.1073/pnas.87.1.414. [DOI] [PMC free article] [PubMed] [Google Scholar]
- Sweeney H. L., Stull J. T. Phosphorylation of myosin in permeabilized mammalian cardiac and skeletal muscle cells. Am J Physiol. 1986 Apr;250(4 Pt 1):C657–C660. doi: 10.1152/ajpcell.1986.250.4.C657. [DOI] [PubMed] [Google Scholar]
- Trybus K. M., Chatman T. A. Chimeric regulatory light chains as probes of smooth muscle myosin function. J Biol Chem. 1993 Feb 25;268(6):4412–4419. [PubMed] [Google Scholar]
- Trybus K. M. Filamentous smooth muscle myosin is regulated by phosphorylation. J Cell Biol. 1989 Dec;109(6 Pt 1):2887–2894. doi: 10.1083/jcb.109.6.2887. [DOI] [PMC free article] [PubMed] [Google Scholar]
- Trybus K. M., Lowey S. Conformational states of smooth muscle myosin. Effects of light chain phosphorylation and ionic strength. J Biol Chem. 1984 Jul 10;259(13):8564–8571. [PubMed] [Google Scholar]
- Trybus K. M., Lowey S. The regulatory light chain is required for folding of smooth muscle myosin. J Biol Chem. 1988 Nov 5;263(31):16485–16492. [PubMed] [Google Scholar]
- Walsh M. P., Dabrowska R., Hinkins S., Hartshorne D. J. Calcium-independent myosin light chain kinase of smooth muscle. Preparation by limited chymotryptic digestion of the calcium ion dependent enzyme, purification, and characterization. Biochemistry. 1982 Apr 13;21(8):1919–1925. doi: 10.1021/bi00537a034. [DOI] [PubMed] [Google Scholar]
- Warshaw D. M., Desrosiers J. M., Work S. S., Trybus K. M. Smooth muscle myosin cross-bridge interactions modulate actin filament sliding velocity in vitro. J Cell Biol. 1990 Aug;111(2):453–463. doi: 10.1083/jcb.111.2.453. [DOI] [PMC free article] [PubMed] [Google Scholar]