Abstract
The decrease in amplitude of the electron spin resonance spectrum of the cysteine-bound spin-label, 3-(maleimidomethyl)-2,2,5,5-tetramethyl-1-pyrrolidinoxyl, brought about by the magnetic interaction with tightly bound manganous ion, was used as a probe of conformational change in actin on binding myosin. The magnitude of this "spin--spin" interaction first decreased then increased on increasing saturation of the actin filament with heavy meromyosin subfragment-1. That the "spin--spin" interaction occurred between spins of adjacent monomers was demonstrated by the observation that the change in magnitude of the "spin--spin" interaction was maintained on binding of heavy meromyosin subfragment-1 to copolymers in which actin monomers containing both manganous ion and spin label were diluted 7-fold with native actin monomers. These data provide evidence for a conformational change in actin on interacting with heavy meromyosin subfragment-1. Further, the fact that not only the magnitude but also the sense of the change in the "spin--spin" interaction is a function of increasing saturation with heavy meromyosin subfragment-1 indicates that the monomers of the actin filament are capable of cooperative interaction in the absence of tropomyosin.
Full text
PDF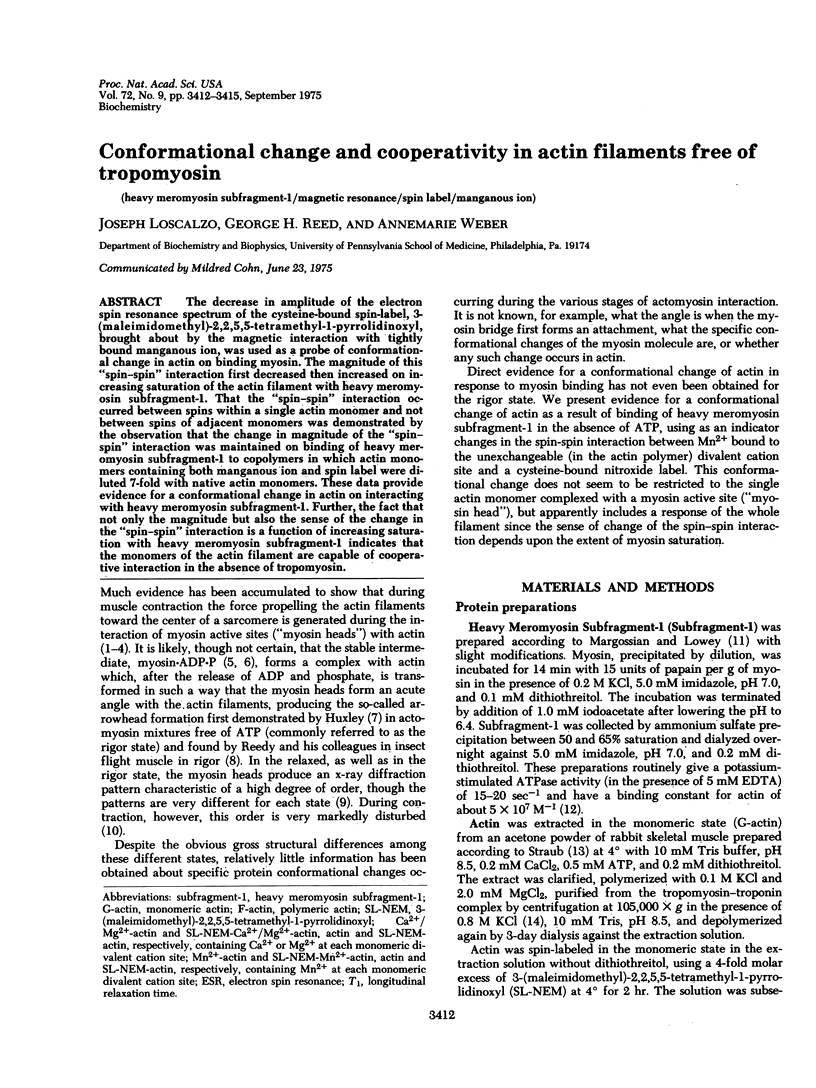
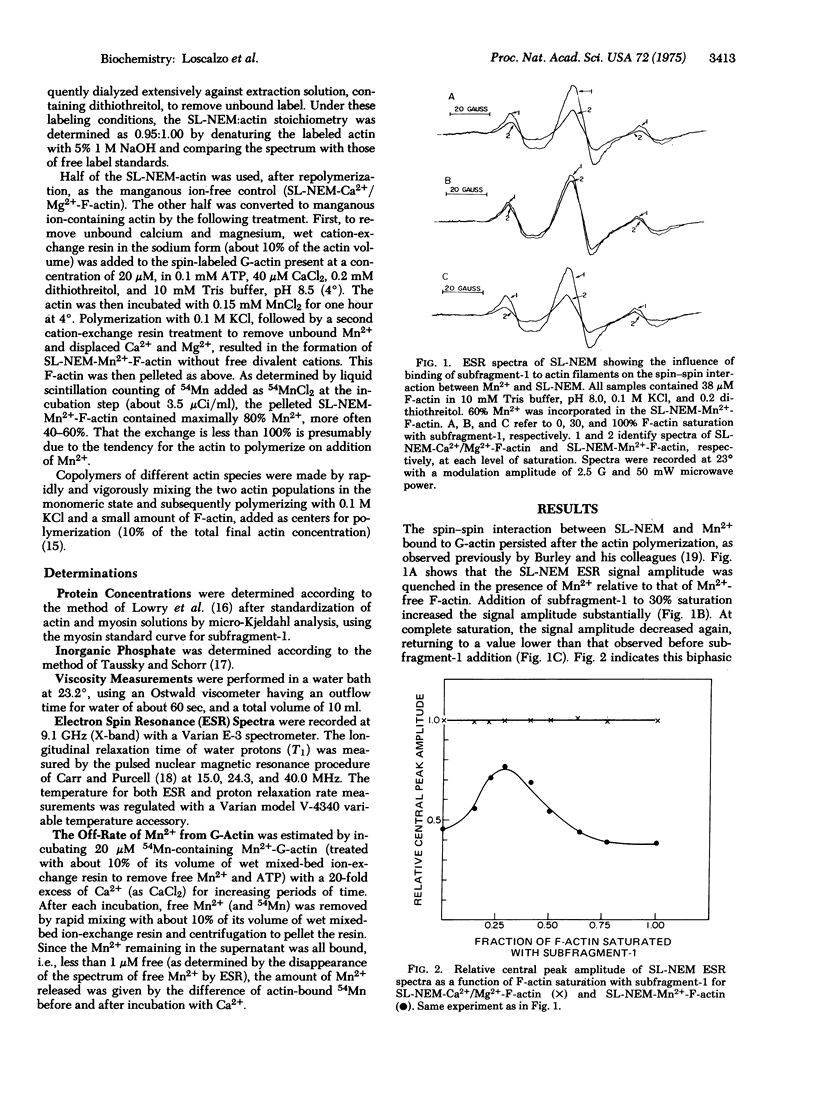
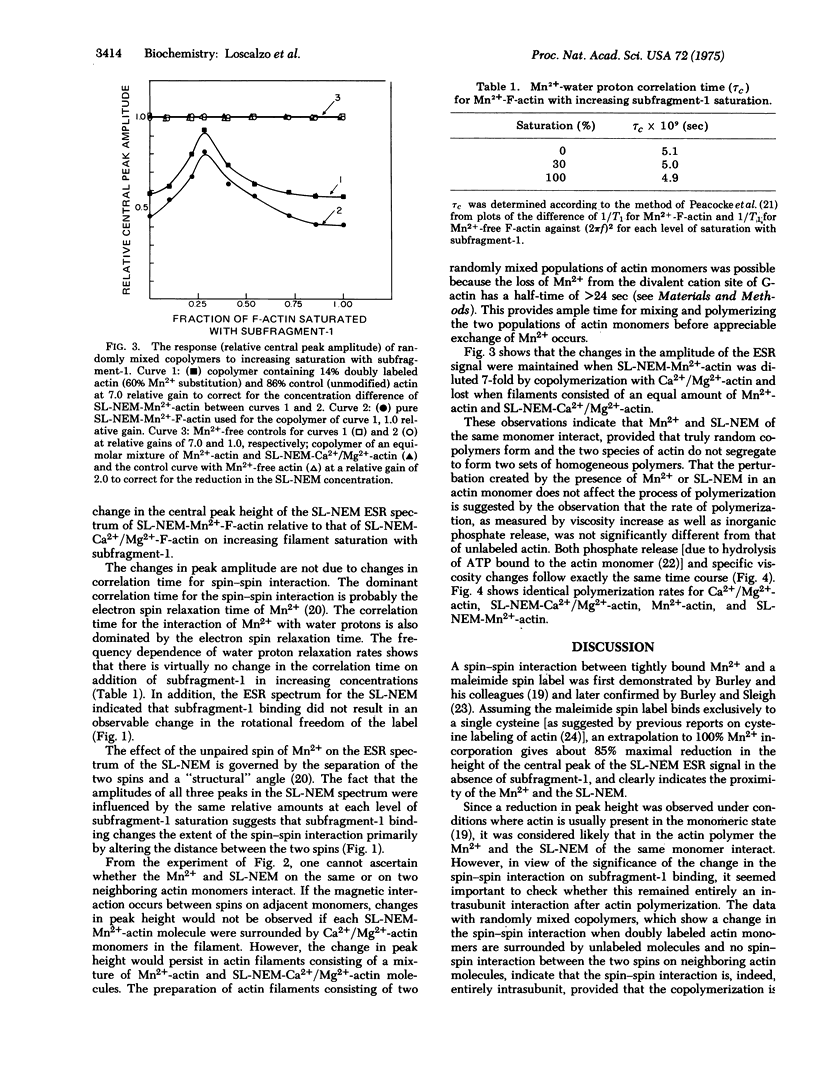
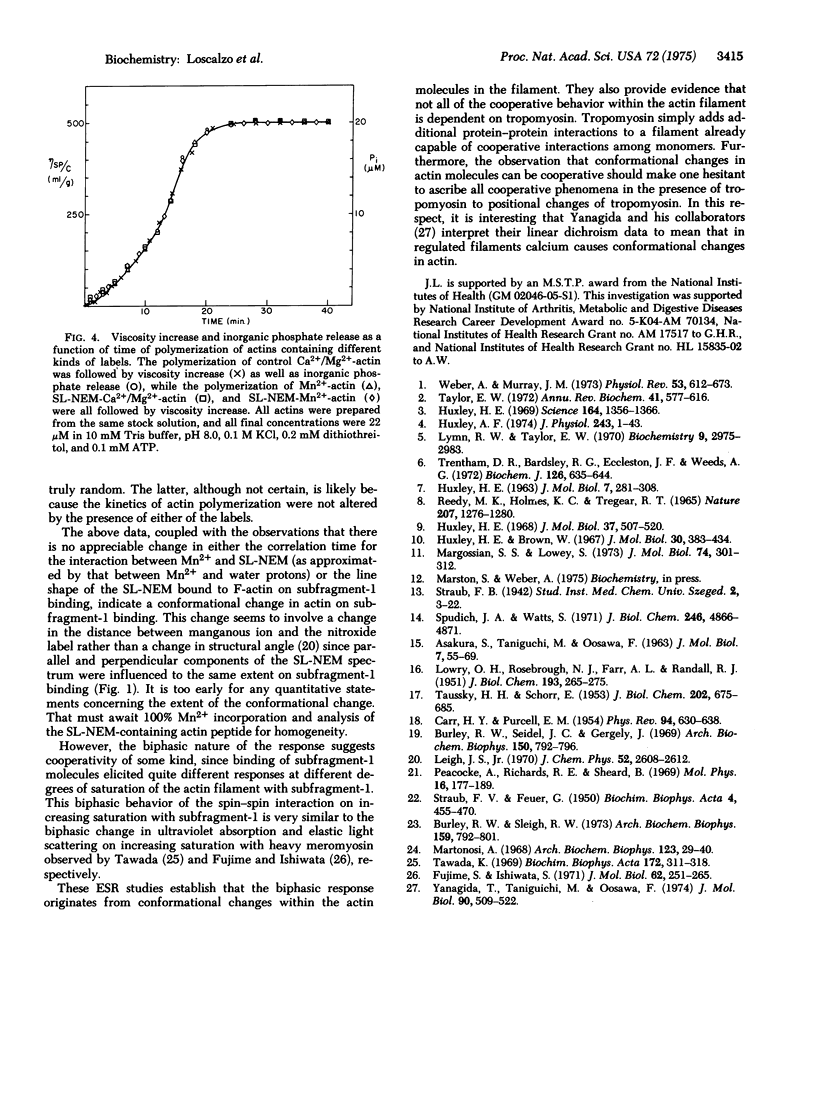
Selected References
These references are in PubMed. This may not be the complete list of references from this article.
- Burley R. W., Seidel J. C., Gergely J. The effect of divalent metal binding on the electron spin resonance spectra of spin-labeled actin. Evidence for spin-spin interactions involving manganese. II. Arch Biochem Biophys. 1972 Jun;150(2):792–796. doi: 10.1016/0003-9861(72)90100-2. [DOI] [PubMed] [Google Scholar]
- Fujime S., Ishiwata S. Dynamic study of F-actin by quasielastic scattering of laser light. J Mol Biol. 1971 Nov 28;62(1):251–265. doi: 10.1016/0022-2836(71)90144-6. [DOI] [PubMed] [Google Scholar]
- Huxley A. F. Muscular contraction. J Physiol. 1974 Nov;243(1):1–43. [PMC free article] [PubMed] [Google Scholar]
- Huxley H. E., Brown W. The low-angle x-ray diagram of vertebrate striated muscle and its behaviour during contraction and rigor. J Mol Biol. 1967 Dec 14;30(2):383–434. doi: 10.1016/s0022-2836(67)80046-9. [DOI] [PubMed] [Google Scholar]
- Huxley H. E. Structural difference between resting and rigor muscle; evidence from intensity changes in the lowangle equatorial x-ray diagram. J Mol Biol. 1968 Nov 14;37(3):507–520. doi: 10.1016/0022-2836(68)90118-6. [DOI] [PubMed] [Google Scholar]
- Huxley H. E. The mechanism of muscular contraction. Science. 1969 Jun 20;164(3886):1356–1365. doi: 10.1126/science.164.3886.1356. [DOI] [PubMed] [Google Scholar]
- LOWRY O. H., ROSEBROUGH N. J., FARR A. L., RANDALL R. J. Protein measurement with the Folin phenol reagent. J Biol Chem. 1951 Nov;193(1):265–275. [PubMed] [Google Scholar]
- Lymn R. W., Taylor E. W. Transient state phosphate production in the hydrolysis of nucleoside triphosphates by myosin. Biochemistry. 1970 Jul 21;9(15):2975–2983. doi: 10.1021/bi00817a007. [DOI] [PubMed] [Google Scholar]
- Margossian S. S., Lowey S. Substructure of the myosin molecule. 3. Preparation of single-headed derivatives of myosin. J Mol Biol. 1973 Mar 5;74(3):301–311. doi: 10.1016/0022-2836(73)90375-6. [DOI] [PubMed] [Google Scholar]
- Martonosi A. The sulfhydryl groups of actin. Arch Biochem Biophys. 1968 Jan;123(1):29–40. doi: 10.1016/0003-9861(68)90100-8. [DOI] [PubMed] [Google Scholar]
- Reedy M. K., Holmes K. C., Tregear R. T. Induced changes in orientation of the cross-bridges of glycerinated insect flight muscle. Nature. 1965 Sep 18;207(5003):1276–1280. doi: 10.1038/2071276a0. [DOI] [PubMed] [Google Scholar]
- Spudich J. A., Watt S. The regulation of rabbit skeletal muscle contraction. I. Biochemical studies of the interaction of the tropomyosin-troponin complex with actin and the proteolytic fragments of myosin. J Biol Chem. 1971 Aug 10;246(15):4866–4871. [PubMed] [Google Scholar]
- TAUSSKY H. H., SHORR E. A microcolorimetric method for the determination of inorganic phosphorus. J Biol Chem. 1953 Jun;202(2):675–685. [PubMed] [Google Scholar]
- Tawada K. Physicochemical studies of F-actin-heavy meromyosin solutions. Biochim Biophys Acta. 1969 Feb 25;172(2):311–318. doi: 10.1016/0005-2728(69)90073-5. [DOI] [PubMed] [Google Scholar]
- Taylor E. W. Chemistry of muscle contraction. Annu Rev Biochem. 1972;41(10):577–616. doi: 10.1146/annurev.bi.41.070172.003045. [DOI] [PubMed] [Google Scholar]
- Trentham D. R., Bardsley R. G., Eccleston J. F., Weeds A. G. Elementary processes of the magnesium ion-dependent adenosine triphosphatase activity of heavy meromyosin. A transient kinetic approach to the study of kinases and adenosine triphosphatases and a colorimetric inorganic phosphate assay in situ. Biochem J. 1972 Feb;126(3):635–644. doi: 10.1042/bj1260635. [DOI] [PMC free article] [PubMed] [Google Scholar]
- Weber A., Murray J. M. Molecular control mechanisms in muscle contraction. Physiol Rev. 1973 Jul;53(3):612–673. doi: 10.1152/physrev.1973.53.3.612. [DOI] [PubMed] [Google Scholar]
- Yanagida T., Taniguchi M., Oosawa F. Conformational changes of F-actin in the thin filaments of muscle induced in vivo and in vitro by calcium ions. J Mol Biol. 1974 Dec 15;90(3):509–522. doi: 10.1016/0022-2836(74)90231-9. [DOI] [PubMed] [Google Scholar]