Abstract
Addition of ubiquinone-1 to E. coli ML 308-225 membrane vesicles dramatically increases coupling between NADH oxidation and active transport such that initial rates and steady-state levels of lactose and amino-acid accumulation are comparable to those observed during D-lactate oxidation. Similar but less dramatic effects are observed with the quinone and succinate or L-lactate. In the presence of NADH and ubiquinone-1, the vesicles also generate a membrane potential (interior negative) that is similar in magnitude to that observed in the presence of D-lactate. Stimulation of NADH-dependent transport by ubiquinone-1 cannot be accounted for by increased rates of oxidation of NADH, and the effect of the quinone on NADH-dependent lactose transport is not observed in vesicles depleted of NADH dehydrogenase activity. Thus, it is apparent that ubiquinone-1 shunts electrons from NADH dehydrogenase [NADH:(acceptor)oxidoreductase; EC 1.6.99.3] to the portion of the respiratory chain containing the energy-coupling site. The findings demonstrate unequivocally that inefficient coupling of NADH oxidation to active transport cannot be due to the presence of inverted vesicles. In addition, they provide further support for specific localization of the energy-coupling site.
Full text
PDF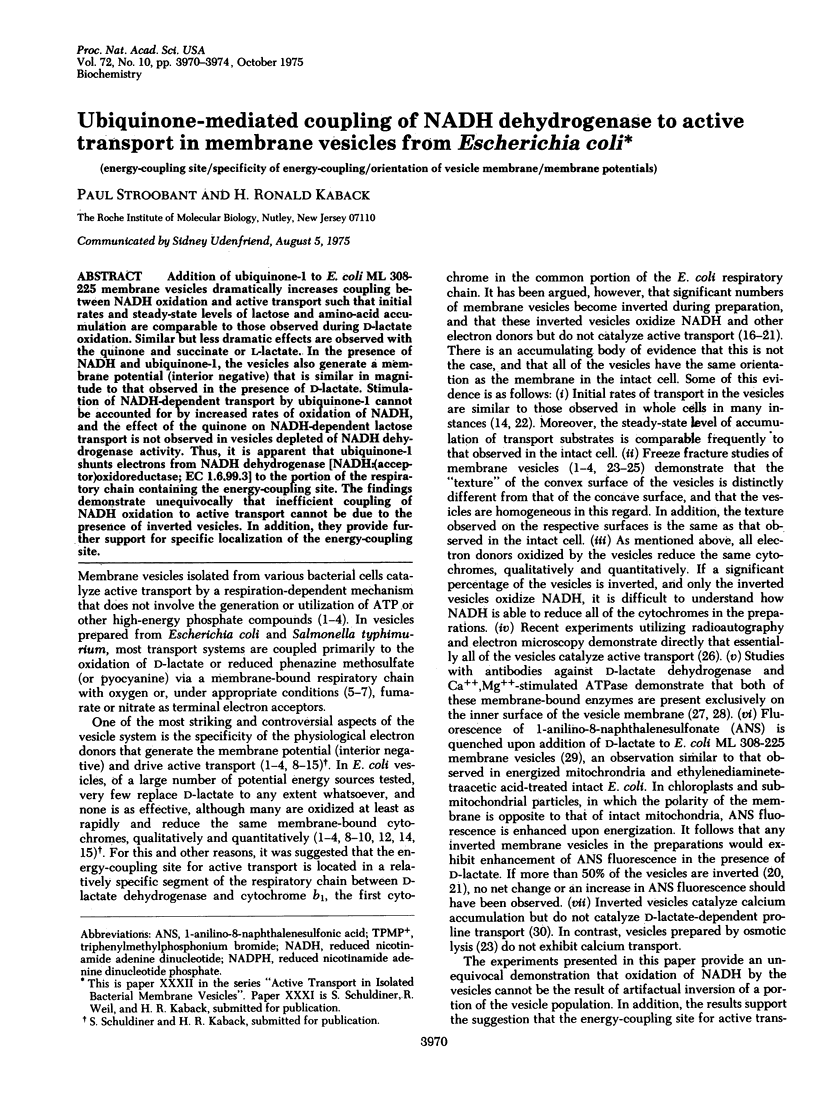
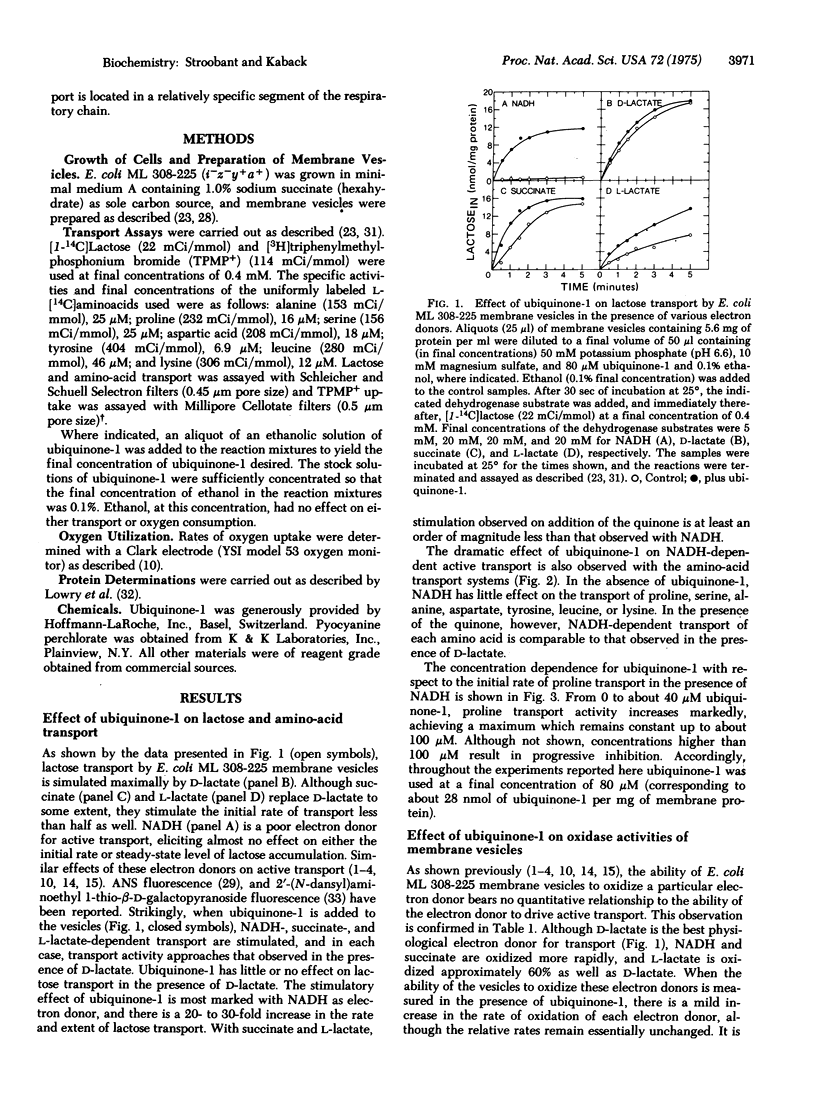
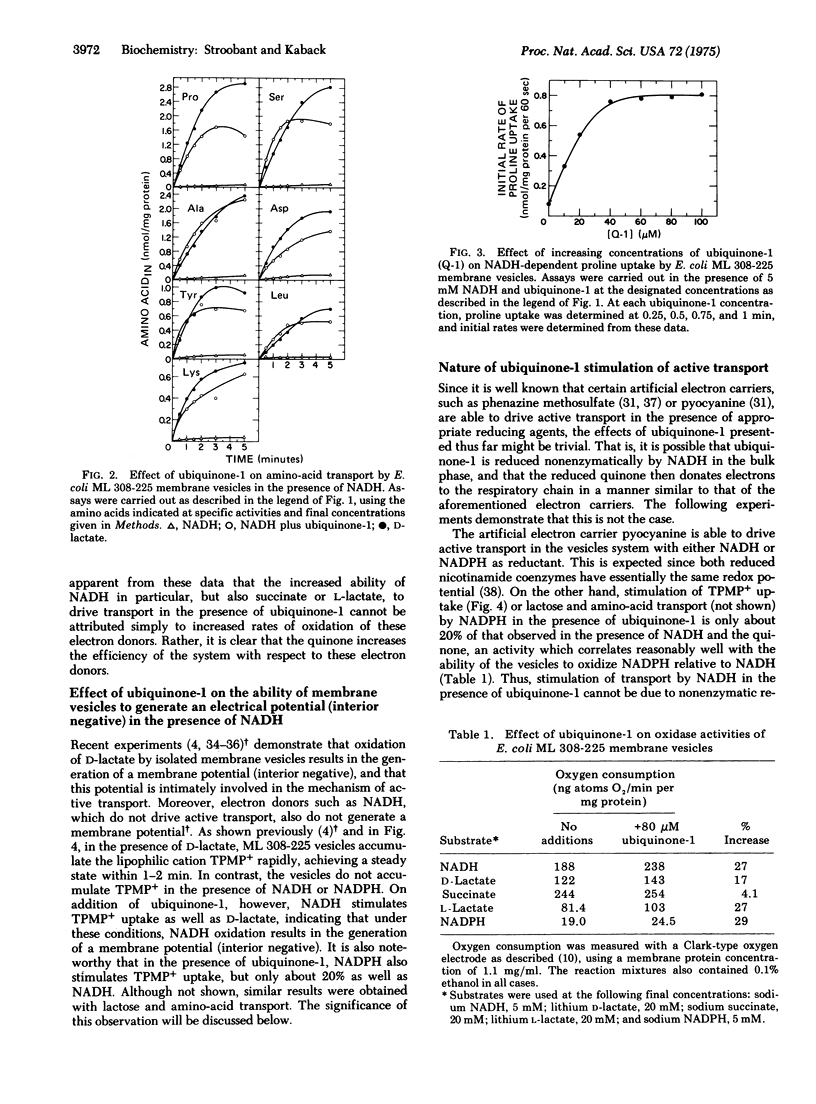
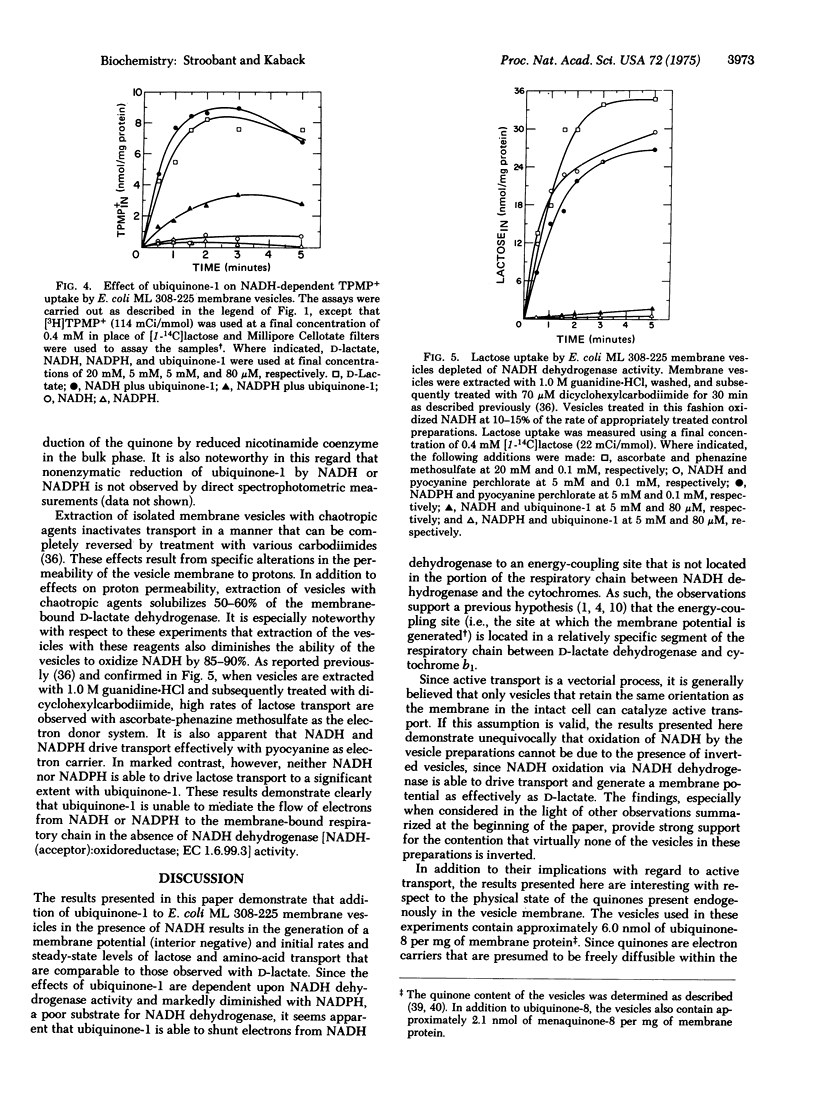
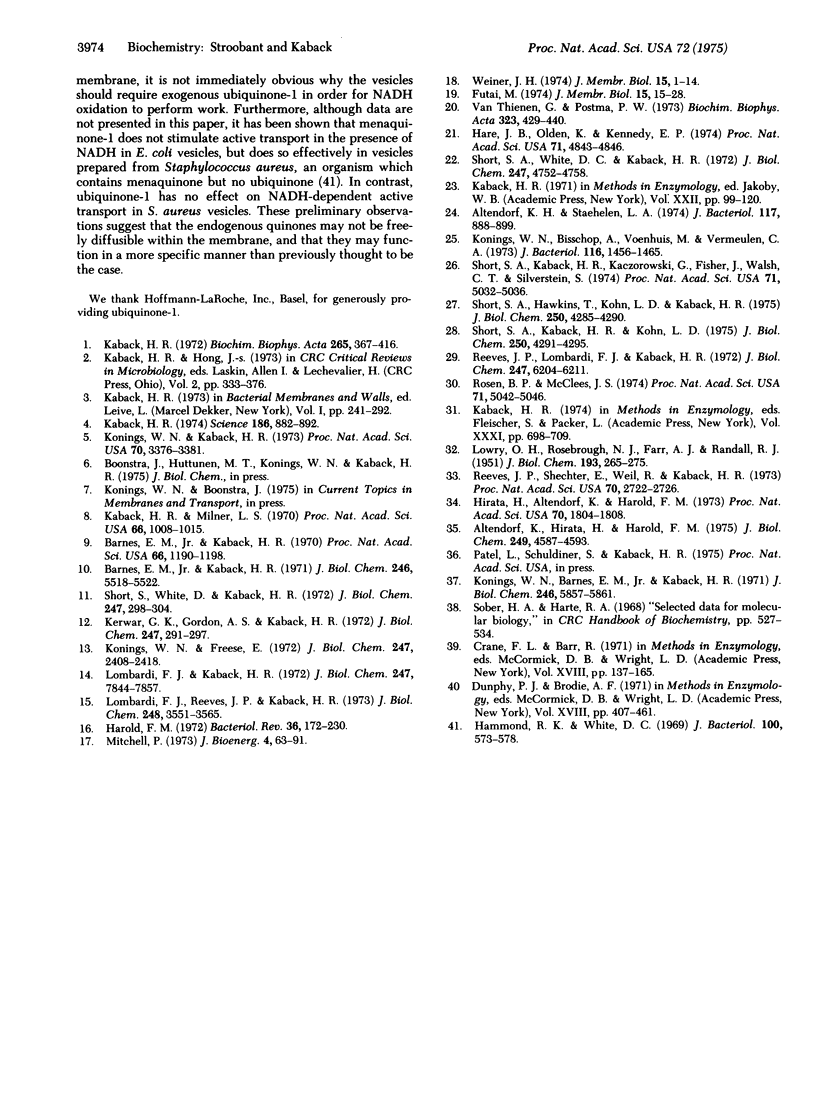
Selected References
These references are in PubMed. This may not be the complete list of references from this article.
- Altendorf K. H., Staehelin L. A. Orientation of membrane vesicles from Escherichia coli as detected by freeze-cleave electron microscopy. J Bacteriol. 1974 Feb;117(2):888–899. doi: 10.1128/jb.117.2.888-899.1974. [DOI] [PMC free article] [PubMed] [Google Scholar]
- Altendorf K., Harold F. M., Simoni R. D. Impairment and restoration of the energized state in membrane vesicles of a mutant of Escherichia coli lacking adenosine triphosphatase. J Biol Chem. 1974 Jul 25;249(14):4587–4593. [PubMed] [Google Scholar]
- Barnes E. M., Jr, Kaback H. R. Beta-galactoside transport in bacterial membrane preparations: energy coupling via membrane-bounded D-lactic dehydrogenase. Proc Natl Acad Sci U S A. 1970 Aug;66(4):1190–1198. doi: 10.1073/pnas.66.4.1190. [DOI] [PMC free article] [PubMed] [Google Scholar]
- Barnes E. M., Jr, Kaback H. R. Mechanisms of active transport in isolated membrane vesicles. I. The site of energy coupling between D-lactic dehydrogenase and beta-galactoside transport in Escherichia coli membrane vesicles. J Biol Chem. 1971 Sep 10;246(17):5518–5522. [PubMed] [Google Scholar]
- Futai M. Orientation of membrane vesicles from Escherichia coli prepared by different procedures. J Membr Biol. 1974;15(1):15–28. doi: 10.1007/BF01870079. [DOI] [PubMed] [Google Scholar]
- Hammond R. K., White D. C. Formation of vitamin K2 isoprenologues by Staphylococcus aureus. J Bacteriol. 1969 Nov;100(2):573–578. doi: 10.1128/jb.100.2.573-578.1969. [DOI] [PMC free article] [PubMed] [Google Scholar]
- Hare J. F., Olden K., Kennedy E. P. Heterogeneity of membrane vesicles from Escherichia coli and their subfractionation with antibody to ATPase. Proc Natl Acad Sci U S A. 1974 Dec;71(12):4843–4846. doi: 10.1073/pnas.71.12.4843. [DOI] [PMC free article] [PubMed] [Google Scholar]
- Harold F. M. Conservation and transformation of energy by bacterial membranes. Bacteriol Rev. 1972 Jun;36(2):172–230. doi: 10.1128/br.36.2.172-230.1972. [DOI] [PMC free article] [PubMed] [Google Scholar]
- Hirata H., Altendorf K., Harold F. M. Role of an electrical potential in the coupling of metabolic energy to active transport by membrane vesicles of Escherichia coli. Proc Natl Acad Sci U S A. 1973 Jun;70(6):1804–1808. doi: 10.1073/pnas.70.6.1804. [DOI] [PMC free article] [PubMed] [Google Scholar]
- Kaback H. R., Milner L. S. Relationship of a membrane-bound D-(-)-lactic dehydrogenase to amino acid transport in isolated bacterial membrane preparations. Proc Natl Acad Sci U S A. 1970 Jul;66(3):1008–1015. doi: 10.1073/pnas.66.3.1008. [DOI] [PMC free article] [PubMed] [Google Scholar]
- Kaback H. R. Transport across isolated bacterial cytoplasmic membranes. Biochim Biophys Acta. 1972 Aug 4;265(3):367–416. doi: 10.1016/0304-4157(72)90014-7. [DOI] [PubMed] [Google Scholar]
- Kaback H. R. Transport studies in bacterial membrane vesicles. Science. 1974 Dec 6;186(4167):882–892. doi: 10.1126/science.186.4167.882. [DOI] [PubMed] [Google Scholar]
- Kerwar G. K., Gordon A. S., Kaback H. R. Mechanisms of active transport in isolated membrane vesicles. IV. Galactose transport by isolated membrane vesicles from Escherichia coli. J Biol Chem. 1972 Jan 10;247(1):291–297. [PubMed] [Google Scholar]
- Konings W. N., Barnes E. M., Jr, Kaback H. R. Mechanisms of active transport in isolated membrane vesicles. 2. The coupling of reduced phenazine methosulfate to the concentrative uptake of beta-galactosides and amino acids. J Biol Chem. 1971 Oct 10;246(19):5857–5861. [PubMed] [Google Scholar]
- Konings W. N., Bisschop A., Veenhuis M., Vermeulen C. A. New procedure for the isolation of membrane vesicles of Bacillus subtilis and an electron microscopy study of their ultrastructure. J Bacteriol. 1973 Dec;116(3):1456–1465. doi: 10.1128/jb.116.3.1456-1465.1973. [DOI] [PMC free article] [PubMed] [Google Scholar]
- Konings W. N., Freese E. Amino acid transport in membrane vesicles of Bacillus subtilis. J Biol Chem. 1972 Apr 25;247(8):2408–2418. [PubMed] [Google Scholar]
- Konings W. N., Kaback H. R. Anaerobic transport in Escherichia coli membrane vesicles. Proc Natl Acad Sci U S A. 1973 Dec;70(12):3376–3381. doi: 10.1073/pnas.70.12.3376. [DOI] [PMC free article] [PubMed] [Google Scholar]
- LOWRY O. H., ROSEBROUGH N. J., FARR A. L., RANDALL R. J. Protein measurement with the Folin phenol reagent. J Biol Chem. 1951 Nov;193(1):265–275. [PubMed] [Google Scholar]
- Lombardi F. J., Kaback H. R. Mechanisms of active transport in isolated bacterial membrane vesicles. 8. The transport of amino acids by membranes prepared from Escherichia coli. J Biol Chem. 1972 Dec 25;247(24):7844–7857. [PubMed] [Google Scholar]
- Lombardi F. J., Reeves J. P., Kaback H. R. Mechanisms of active transport in isolated bacterial membrane vesicles. 8. Valinomycin-induced rubidium transport. J Biol Chem. 1973 May 25;248(10):3551–3565. [PubMed] [Google Scholar]
- Mitchell P. Performance and conservation of osmotic work by proton-coupled solute porter systems. J Bioenerg. 1973 Jan;4(1):63–91. doi: 10.1007/BF01516051. [DOI] [PubMed] [Google Scholar]
- Reeves J. P., Lombardi F. J., Kaback H. R. Mechanisms of active transport in isolated bacterial membrane vesicles. VII. Fluorescence of 1-anilino-8-naphthalenesulfonate during D-lactate oxidation by membrane vesicles from Escherichia coli. J Biol Chem. 1972 Oct 10;247(19):6204–6211. [PubMed] [Google Scholar]
- Reeves J. P., Shechter E., Weil R., Kaback H. R. Dansyl-galactoside, a fluorescent probe of active transport in bacterial membrane vesicles. Proc Natl Acad Sci U S A. 1973 Oct;70(10):2722–2726. doi: 10.1073/pnas.70.10.2722. [DOI] [PMC free article] [PubMed] [Google Scholar]
- Rosen B. P., McClees J. S. Active transport of calcium in inverted membrane vesicles of Escherichia coli. Proc Natl Acad Sci U S A. 1974 Dec;71(12):5042–5046. doi: 10.1073/pnas.71.12.5042. [DOI] [PMC free article] [PubMed] [Google Scholar]
- Short S. A., Kaback H. R., Hawkins T., Kohn L. D. Immunochemical properties of the membrane-bound D-lactate dehydrogenase from Escherichia coli. J Biol Chem. 1975 Jun 10;250(11):4285–4290. [PubMed] [Google Scholar]
- Short S. A., Kaback H. R., Kaczorowski G., Fisher J., Walsh C. T., Silverstein S. C. Determination of the absolute number of Escherichia coli membrane vesicles that catalyze active transport. Proc Natl Acad Sci U S A. 1974 Dec;71(12):5032–5036. doi: 10.1073/pnas.71.12.5032. [DOI] [PMC free article] [PubMed] [Google Scholar]
- Short S. A., Kaback H. R., Kohn L. D. Localization of D-lactate dehydrogenase in native and reconstituted Escherichia coli membrane vesicles. J Biol Chem. 1975 Jun 10;250(11):4291–4296. [PubMed] [Google Scholar]
- Short S. A., White D. C., Kaback H. R. Active transport in isolated bacterial membrane vesicles. V. The transport of amino acids by membrane vesicles prepared from Staphylococcus aureus. J Biol Chem. 1972 Jan 10;247(1):298–304. [PubMed] [Google Scholar]
- Weiner J. H. The localization of glycerol-3-phosphate dehydrogenase in Escherichia coli. J Membr Biol. 1974;15(1):1–14. doi: 10.1007/BF01870078. [DOI] [PubMed] [Google Scholar]
- van Thienen G., Postma P. W. Coupling between energy conservation and active transport of serine in Escherichia coli. Biochim Biophys Acta. 1973 Oct 25;323(3):429–440. doi: 10.1016/0005-2736(73)90188-0. [DOI] [PubMed] [Google Scholar]