Abstract
Electron microscope studies have been conducted on individual fibers of human deoxyhemoglobin S (sickle cell hemoglobin). The fibers are obtained by injection of gelled samples into a large excess of glutaraldehyde, which quickly stabilizes the fibers by cross-linking. The fibers are negatively stained with phosphotungstic acid or shadowed with platinum-carbon. The fibers are approximately 200 Å in diameter, and display long and short helical striations with an opposite handedness. The long striations occur at an angle of about 15° from the fiber axis, and complete one turn around the helix at a distance of about 2 × 103 Å along the fiber axis. The short striations occur at an angle of about 80° from the fiber axis, with a spacing of about 65 Å, and complete one turn around the helix at a distance along the fiber axis of about 130 Å. The structure of the fiber appears to be a sextuple helix in terms of the long striations, and a double helix in terms of the short striations. The shadowed samples are consistent with a left-handed screw sense for the short striations, thus implying a right-handed sense for the long striations. A structural model incorporating these features is compatible with the atomic structure of hemoglobin, with individual molecules oriented with their dyad axis of symmetry perpendicular to the fiber axis and their α1-β1 pseudo-dyad axis roughly parallel to the fiber axis. This orientation places the two β-6 regions of each molecule (sites of the sickle cell mutation) in contact with the β-6 regions of the molecules above and below along the long striations. Both the long and short striations are accounted for by individual hemoglobin molecules arranged in double helical arrays with 6.4 molecules per turn in each array.
Keywords: blood, anemia, electron microscopy, double helix
Full text
PDF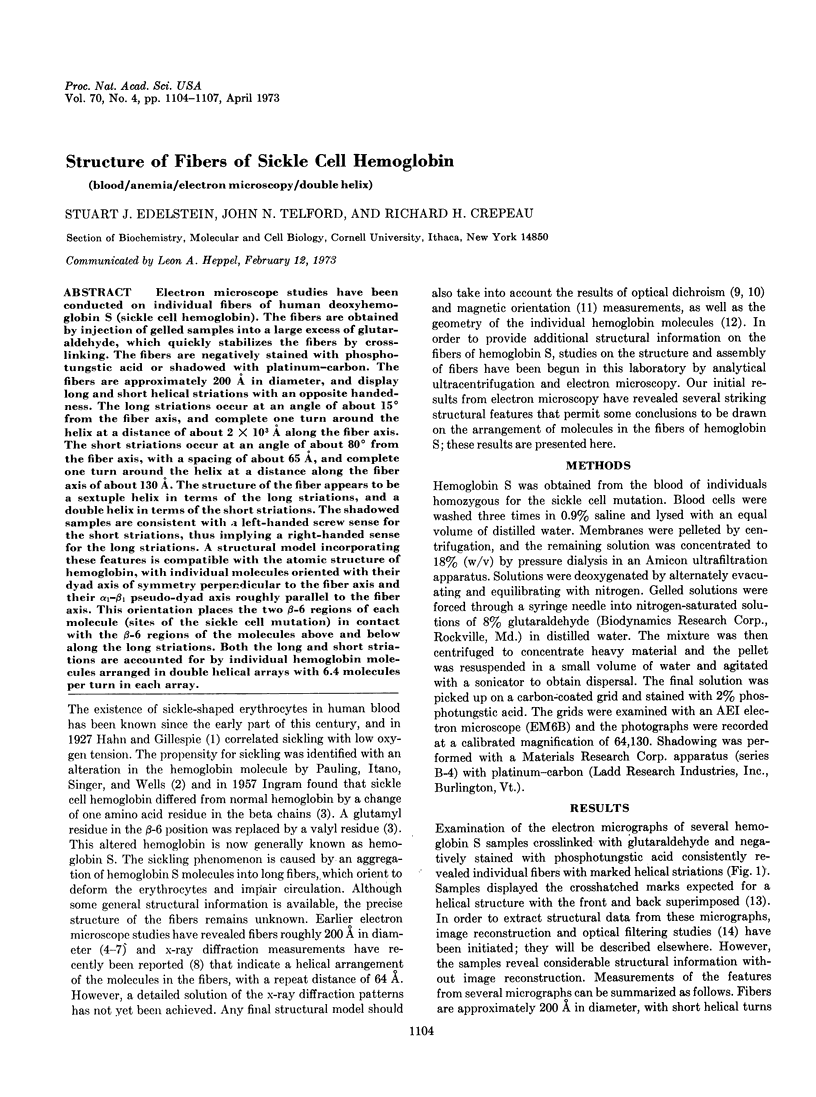
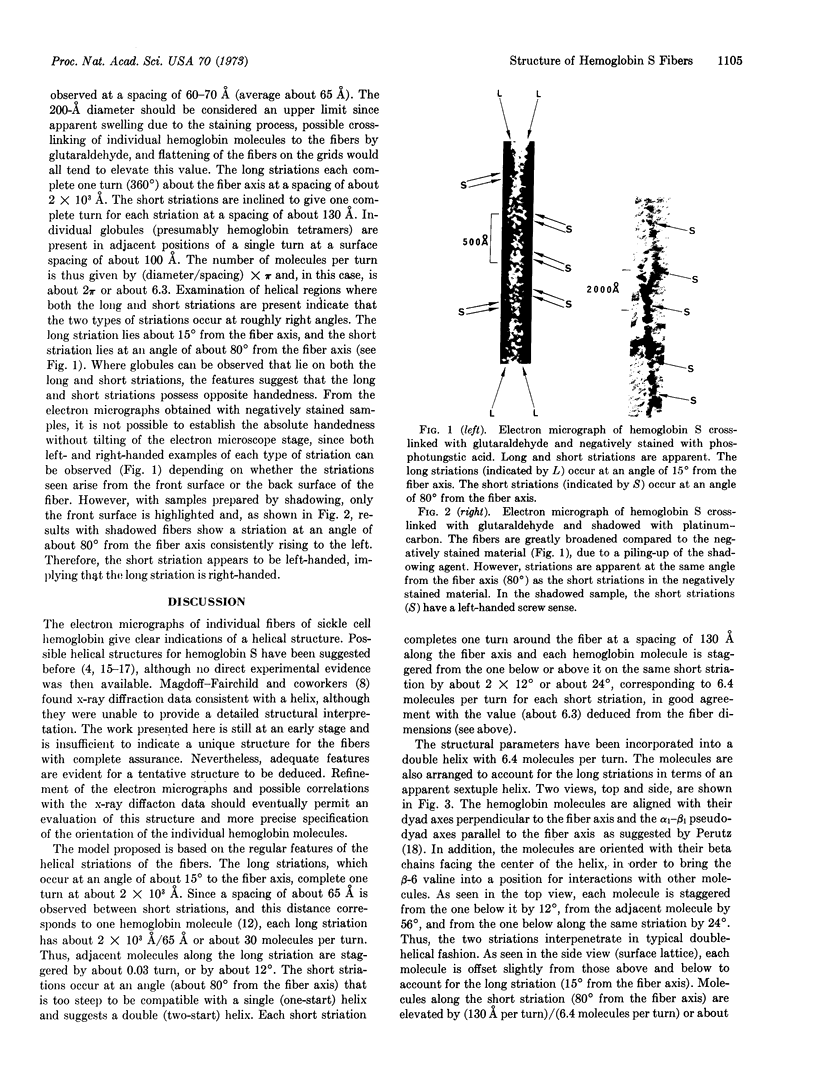
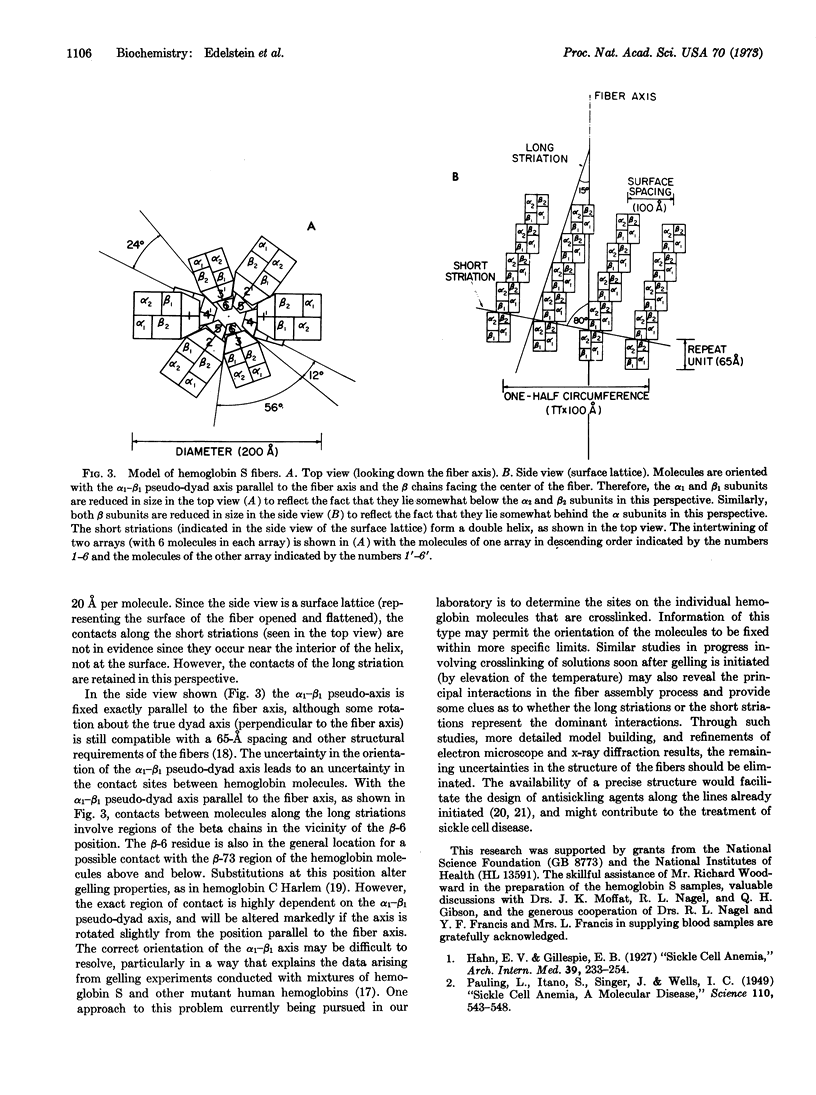
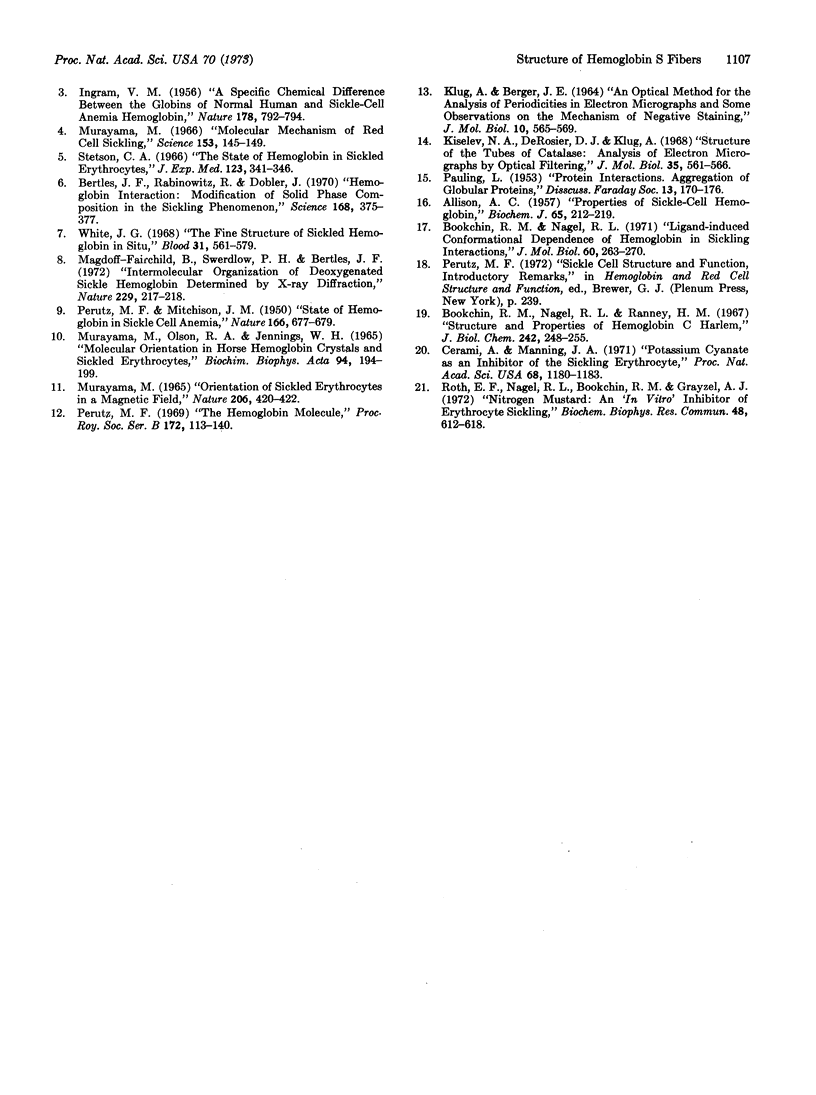
Images in this article
Selected References
These references are in PubMed. This may not be the complete list of references from this article.
- ALLISON A. C. Properties of sickle-cell haemoglobin. Biochem J. 1957 Feb;65(2):212–219. doi: 10.1042/bj0650212. [DOI] [PMC free article] [PubMed] [Google Scholar]
- Bertles J. F., Rabinowitz R., Döbler J. Hemoglobin interaction: modification of solid phase composition in the sickling phenomenon. Science. 1970 Jul 24;169(3943):375–377. doi: 10.1126/science.169.3943.375. [DOI] [PubMed] [Google Scholar]
- Bookchin R. M., Nagel R. L. Ligand-induced conformational dependence of hemoglobin in sickling interactios. J Mol Biol. 1971 Sep 14;60(2):263–270. doi: 10.1016/0022-2836(71)90292-0. [DOI] [PubMed] [Google Scholar]
- Bookchin R. M., Nagel R. L., Ranney H. M. Structure and properties of hemoglobin C-Harlem, a human hemoglobin variant with amino acid substitutions in 2 residues of the beta-polypeptide chain. J Biol Chem. 1967 Jan 25;242(2):248–255. [PubMed] [Google Scholar]
- Cerami A., Manning J. M. Potassium cyanate as an inhibitor of the sickling of erythrocytes in vitro. Proc Natl Acad Sci U S A. 1971 Jun;68(6):1180–1183. doi: 10.1073/pnas.68.6.1180. [DOI] [PMC free article] [PubMed] [Google Scholar]
- INGRAM V. M. A specific chemical difference between the globins of normal human and sickle-cell anaemia haemoglobin. Nature. 1956 Oct 13;178(4537):792–794. doi: 10.1038/178792a0. [DOI] [PubMed] [Google Scholar]
- KLUG A., BERGER J. E. AN OPTICAL METHOD FOR THE ANALYSIS OF PERIODICITIES IN ELECTRON MICROGRAPHS, AND SOME OBSERVATIONS ON THE MECHANISM OF NEGATIVE STAINING. J Mol Biol. 1964 Dec;10:565–569. doi: 10.1016/s0022-2836(64)80081-4. [DOI] [PubMed] [Google Scholar]
- Kiselev N. A., De Rosier D. J., Klug A. Structure of the tubes of catalase: analysis of electron micrographs by optical filtering. J Mol Biol. 1968 Aug 14;35(3):561–566. doi: 10.1016/s0022-2836(68)80014-2. [DOI] [PubMed] [Google Scholar]
- MURAYAMA M., OLSON R. A., JENNINGS W. H. MOLECULAR ORIENTATION IN HORSE HEMOGLOBIN CRYSTALS AND SICKLED ERYTHROCYTES. Biochim Biophys Acta. 1965 Jan 25;94:194–199. doi: 10.1016/0926-6585(65)90024-5. [DOI] [PubMed] [Google Scholar]
- Magdoff-Fairchild B., Swerdlow P. H., Bertles J. F. Intermolecular organization of deoxygenated sickle haemoglobin determined by x-ray diffraction. Nature. 1972 Sep 22;239(5369):217–219. doi: 10.1038/239217a0. [DOI] [PubMed] [Google Scholar]
- Murayama M. Molecular mechanism of red cell "sickling". Science. 1966 Jul 8;153(3732):145–149. doi: 10.1126/science.153.3732.145. [DOI] [PubMed] [Google Scholar]
- Murayama M. Orientation of sickled erythrocytes in a magnetic field. Nature. 1965 Apr 24;206(982):420–422. doi: 10.1038/206420a0. [DOI] [PubMed] [Google Scholar]
- PAULING L., ITANO H. A. Sickle cell anemia a molecular disease. Science. 1949 Nov 25;110(2865):543–548. doi: 10.1126/science.110.2865.543. [DOI] [PubMed] [Google Scholar]
- PERUTZ M. F., MITCHISON J. M. State of haemoglobin in sickle-cell anaemia. Nature. 1950 Oct 21;166(4225):677–679. doi: 10.1038/166677a0. [DOI] [PubMed] [Google Scholar]
- Perutz M. F. The Croonian Lecture, 1968. The haemoglobin molecule. Proc R Soc Lond B Biol Sci. 1969 May 20;173(1031):113–140. doi: 10.1098/rspb.1969.0043. [DOI] [PubMed] [Google Scholar]
- Roth E. F., Jr, Nagel R. L., Bookchin R. M., Grayzel A. I. Nitrogen mustard: an "in vitro" inhibitor of erythrocyte sickling. Biochem Biophys Res Commun. 1972 Aug 7;48(3):612–618. doi: 10.1016/0006-291x(72)90392-0. [DOI] [PubMed] [Google Scholar]
- Stetson C. A., Jr The state of hemoglobin in sickled erythrocytes. J Exp Med. 1966 Feb 1;123(2):341–346. doi: 10.1084/jem.123.2.341. [DOI] [PMC free article] [PubMed] [Google Scholar]
- White J. G. The fine structure of sickled hemoglobin in situ. Blood. 1968 May;31(5):561–579. [PubMed] [Google Scholar]