Abstract
Membrane vesicles from E. coli can oxidize D-lactate and other substrates and couple respiration to the active transport of sugars and amino acids. The present experiments bear on the nature of the link between respiration and transport.
Respiring vesicles were found to accumulate dibenzyldimethylammonium ion, a synthetic lipid-soluble cation that serves as an indicator of an electrical potential. The results suggest that oxidation of D-lactate generates a membrane potential, vesicle interior negative, of the order of -100 mV. In vesicles lacking substrate, an electrical potential was created by induction of electrogenic efflux of K+ with the aid of the K+ ionophores, valinomycin and monactin. These conditions induced transient accumulation by the vesicles of [14C]proline and other metabolites. Experiments with inhibitors and ionophores indicate that neither ATP nor the respiratory chain is involved; the electrical potential generated by K+ efflux is coupled directly to the transport systems. The results verify two predictions derived from Mitchell's chemiosmotic hypothesis: respiring vesicles generate an electrical potential of the proper polarity and magnitude; and a membrane potential is in itself sufficient to drive the active transport of amino acids and other metabolites.
Keywords: chemiosmotic hypothesis, lipid-soluble ions, valinomycin, ionophores, amino-acid transport
Full text
PDF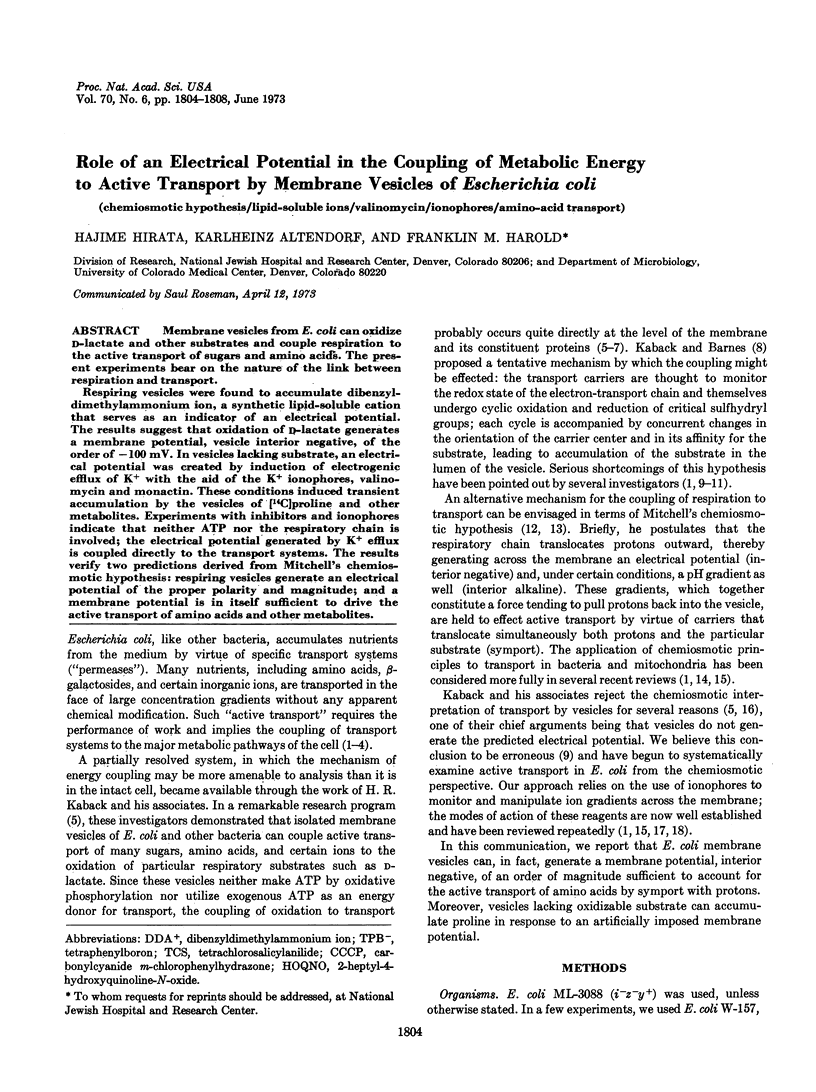
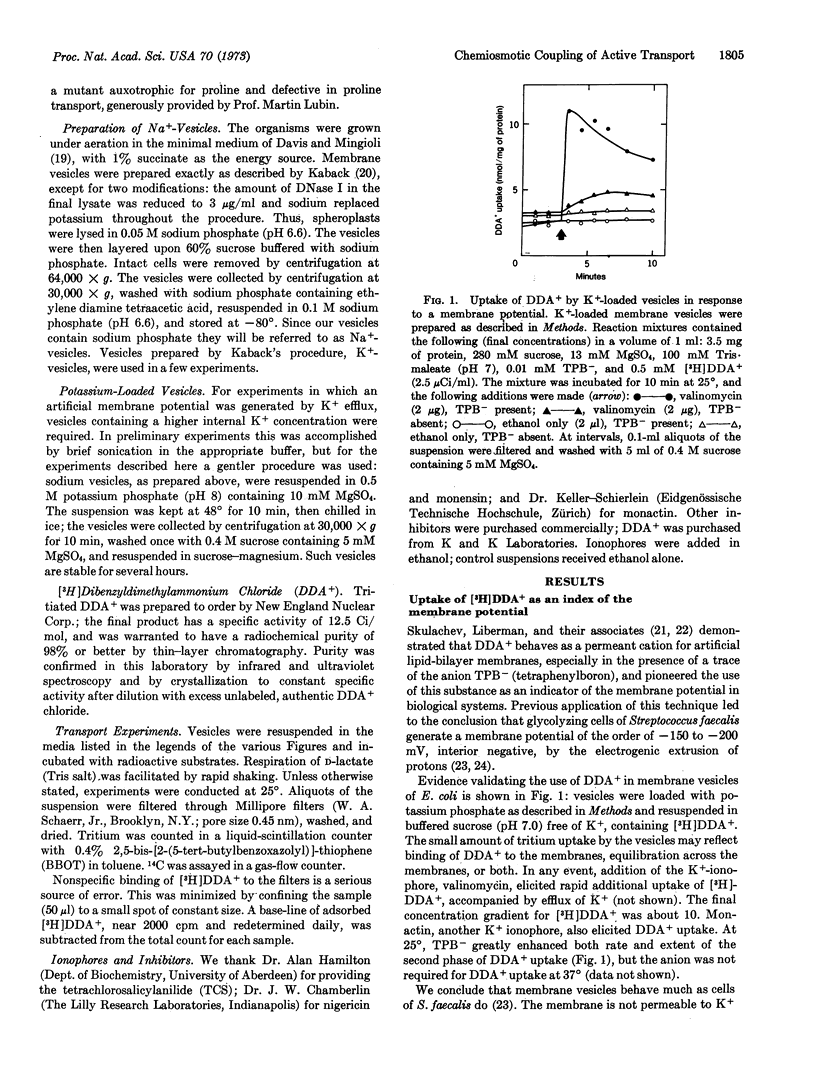
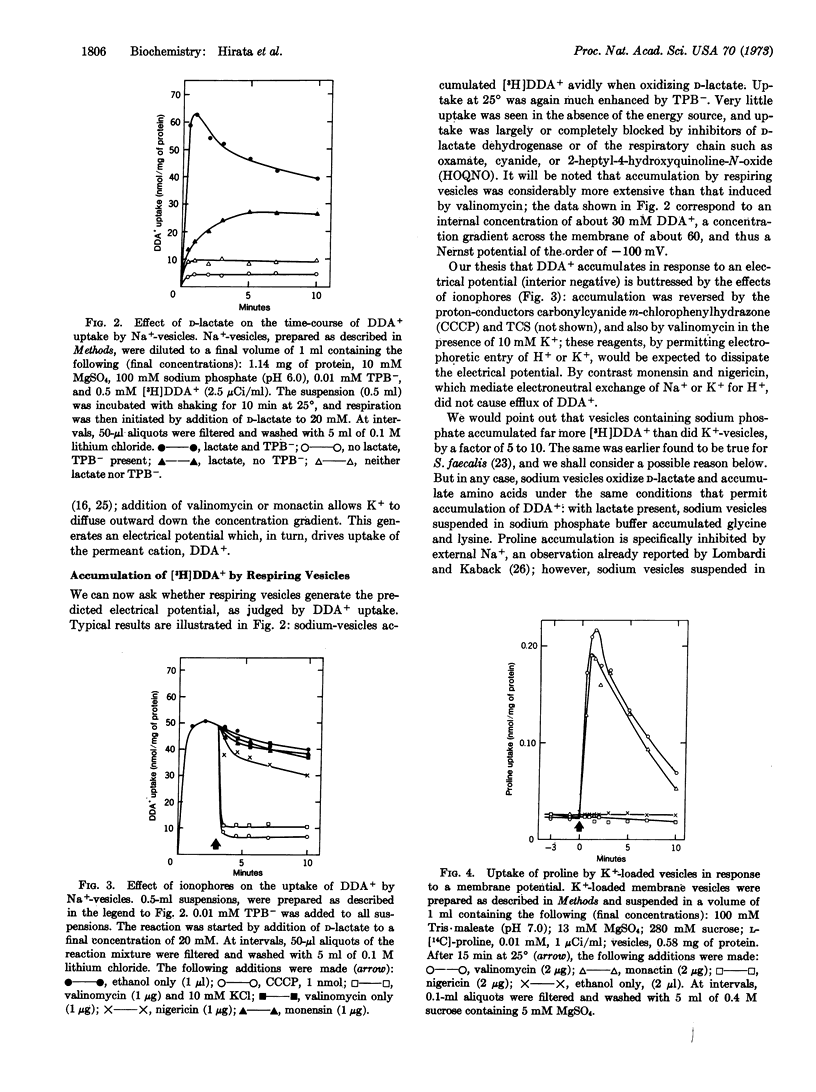
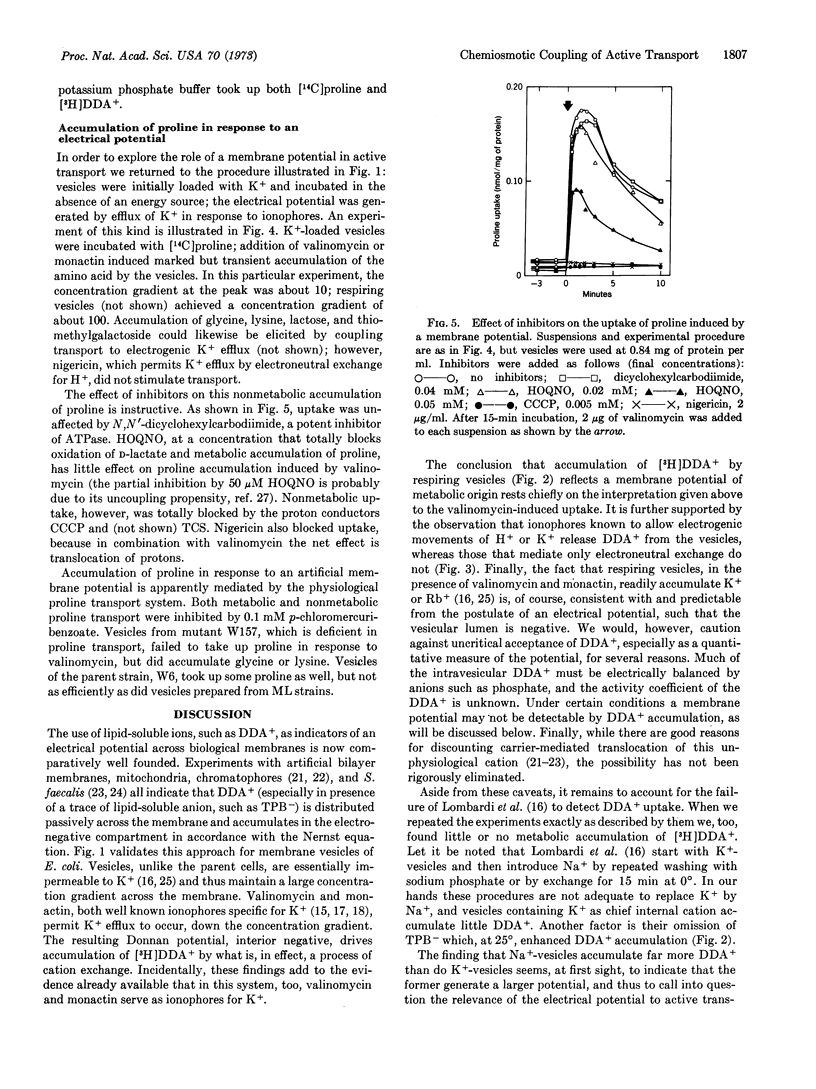
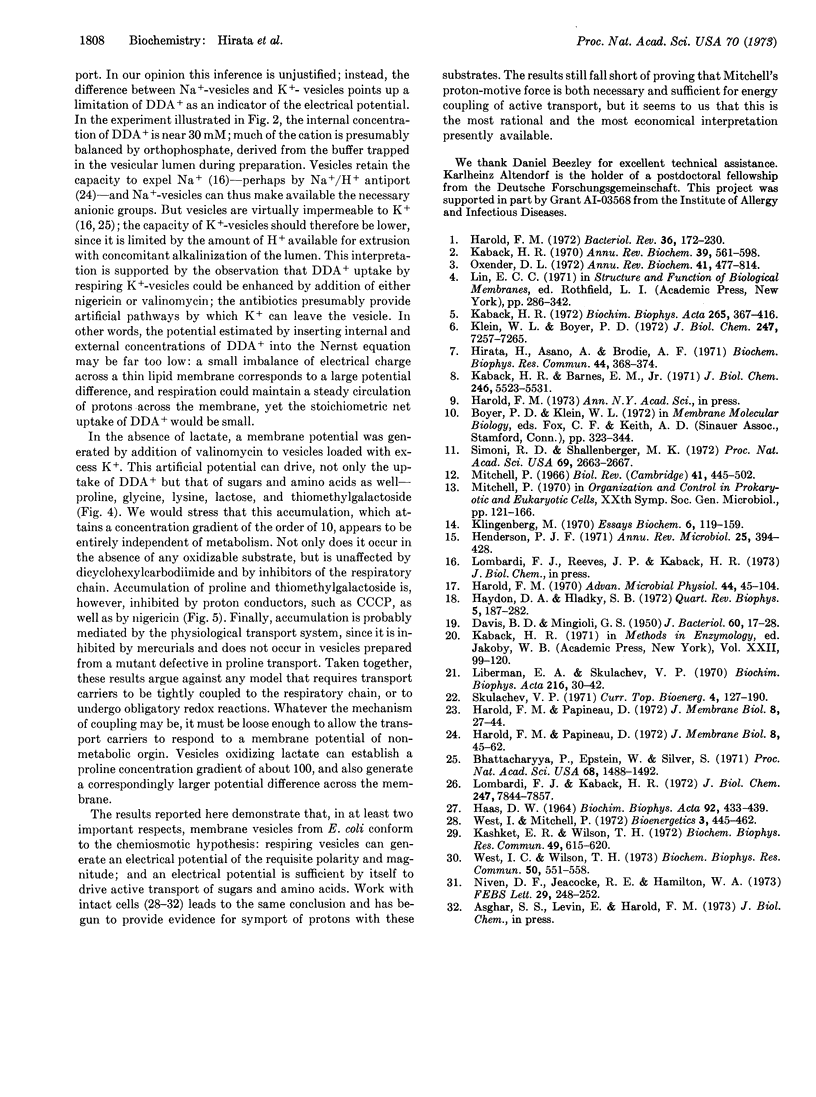
Selected References
These references are in PubMed. This may not be the complete list of references from this article.
- Bhattacharyya P., Epstein W., Silver S. Valinomycin-induced uptake of potassium in membrane vesicles from Escherichia coli. Proc Natl Acad Sci U S A. 1971 Jul;68(7):1488–1492. doi: 10.1073/pnas.68.7.1488. [DOI] [PMC free article] [PubMed] [Google Scholar]
- DAVIS B. D., MINGIOLI E. S. Mutants of Escherichia coli requiring methionine or vitamin B12. J Bacteriol. 1950 Jul;60(1):17–28. doi: 10.1128/jb.60.1.17-28.1950. [DOI] [PMC free article] [PubMed] [Google Scholar]
- HAAS D. PHOSPHORYLATION COUPLED TO THE OXIDATION OF NADH BY FUMARATE IN DIGITONIN FRAGMENTS OF BEEF-HEART MITOCHONDRIA. Biochim Biophys Acta. 1964 Dec 23;92:433–439. doi: 10.1016/0926-6569(64)90002-1. [DOI] [PubMed] [Google Scholar]
- Harold F. M. Conservation and transformation of energy by bacterial membranes. Bacteriol Rev. 1972 Jun;36(2):172–230. doi: 10.1128/br.36.2.172-230.1972. [DOI] [PMC free article] [PubMed] [Google Scholar]
- Harold F. M., Papineau D. Cation transport and electrogenesis by Streptococcus faecalis. I. The membrane potential. J Membr Biol. 1972;8(1):27–44. doi: 10.1007/BF01868093. [DOI] [PubMed] [Google Scholar]
- Harold F. M., Papineau D. Cation transport and electrogenesis by Streptococcus faecalis. II. Proton and sodium extrusion. J Membr Biol. 1972;8(1):45–62. doi: 10.1007/BF01868094. [DOI] [PubMed] [Google Scholar]
- Haydon D. A., Hladky S. B. Ion transport across thin lipid membranes: a critical discussion of mechanisms in selected systems. Q Rev Biophys. 1972 May;5(2):187–282. doi: 10.1017/s0033583500000883. [DOI] [PubMed] [Google Scholar]
- Henderson P. J. Ion transport by energy-conserving biological membranes. Annu Rev Microbiol. 1971;25:393–428. doi: 10.1146/annurev.mi.25.100171.002141. [DOI] [PubMed] [Google Scholar]
- Hirata H., Asano A., Brodie A. F. Respiration dependent transport of proline by electron transport particles from mycobacterium phlei. Biochem Biophys Res Commun. 1971 Jul 16;44(2):368–374. doi: 10.1016/0006-291x(71)90609-7. [DOI] [PubMed] [Google Scholar]
- Kaback H. R., Barnes E. M., Jr Mechanisms of active transport in isolated membrane vesicles. II. The mechanism of energy coupling between D-lactic dehydrogenase and beta-galactoside transport in membrane preparations from Escherichia coli. J Biol Chem. 1971 Sep 10;246(17):5523–5531. [PubMed] [Google Scholar]
- Kaback H. R. Transport across isolated bacterial cytoplasmic membranes. Biochim Biophys Acta. 1972 Aug 4;265(3):367–416. doi: 10.1016/0304-4157(72)90014-7. [DOI] [PubMed] [Google Scholar]
- Kaback H. R. Transport. Annu Rev Biochem. 1970;39:561–598. doi: 10.1146/annurev.bi.39.070170.003021. [DOI] [PubMed] [Google Scholar]
- Kashket E. R., Wilson T. H. Galactoside accumulation associated with ion movements in Streptococcus lactis. Biochem Biophys Res Commun. 1972 Nov 1;49(3):615–620. doi: 10.1016/0006-291x(72)90455-x. [DOI] [PubMed] [Google Scholar]
- Klein W. L., Boyer P. D. Energization of active transport by Escherichia coli. J Biol Chem. 1972 Nov 25;247(22):7257–7265. [PubMed] [Google Scholar]
- Klingenberg M. Metabolite transport in mitochondria: an example for intracellular membrane function. Essays Biochem. 1970;6:119–159. [PubMed] [Google Scholar]
- Liberman E. A., Skulachev V. P. Conversion of biomembrane-produced energy into electric form. IV. General discussion. Biochim Biophys Acta. 1970 Aug 4;216(1):30–42. doi: 10.1016/0005-2728(70)90156-8. [DOI] [PubMed] [Google Scholar]
- Lombardi F. J., Kaback H. R. Mechanisms of active transport in isolated bacterial membrane vesicles. 8. The transport of amino acids by membranes prepared from Escherichia coli. J Biol Chem. 1972 Dec 25;247(24):7844–7857. [PubMed] [Google Scholar]
- Mitchell P. Chemiosmotic coupling in oxidative and photosynthetic phosphorylation. Biol Rev Camb Philos Soc. 1966 Aug;41(3):445–502. doi: 10.1111/j.1469-185x.1966.tb01501.x. [DOI] [PubMed] [Google Scholar]
- Niven D. F., Jeacocke R. E., Hamilton W. A. The membrane potential as the driving force for the accumulation of lysine by Staphylococcus aureus. FEBS Lett. 1973 Feb 1;29(3):248–252. doi: 10.1016/0014-5793(73)80030-4. [DOI] [PubMed] [Google Scholar]
- Oxender D. L. Membrane transport. Annu Rev Biochem. 1972;41(10):777–814. doi: 10.1146/annurev.bi.41.070172.004021. [DOI] [PubMed] [Google Scholar]
- Simoni R. D., Shallenberger M. K. Coupling of energy to active transport of amino acids in Escherichia coli. Proc Natl Acad Sci U S A. 1972 Sep;69(9):2663–2667. doi: 10.1073/pnas.69.9.2663. [DOI] [PMC free article] [PubMed] [Google Scholar]
- West I. C., Wilson T. H. Galactoside transport dissociated from proton movement in mutants of Escherichia coli. Biochem Biophys Res Commun. 1973 Jan 23;50(2):551–558. doi: 10.1016/0006-291x(73)90875-9. [DOI] [PubMed] [Google Scholar]
- West I., Mitchell P. Proton-coupled beta-galactoside translocation in non-metabolizing Escherichia coli. J Bioenerg. 1972 Aug;3(5):445–462. doi: 10.1007/BF01516082. [DOI] [PubMed] [Google Scholar]