Abstract
The influx of Ca2+ ions controls many important processes in excitable cells, including the regulation of the gating of Ca(2+)-activated K+ channels (the current IK[Ca]). Various IK[Ca] channels contribute to the regulation of the action-potential waveform, the repetitive discharge of spikes, and the secretion of neurotransmitters. It is thought that large-conductance IK[Ca] channels must be closely colocalized with Ca2+ channels (ICa) to be gated by Ca2+ influx. We now report that IK[Ca] channels can be preferentially colocalized with pharmacologically distinct subtypes of voltage-activated Ca2+ channel and that this occurs differently in embryonic chicken sympathetic and parasympathetic neurons. The effects of various dihydropyridines and omega-conotoxin on voltage-activated Ca2+ currents (ICa) and Ca(2+)-activated K+ currents (IK[Ca]) were examined by using perforated-patch whole-cell recordings from embryonic chicken ciliary and sympathetic ganglion neurons. Application of nifedipine or omega-conotoxin each caused a 40-60% reduction in ICa, whereas application of S-(-)-BAY K 8644 potentiated ICa in ciliary ganglion neurons. But application of omega-conotoxin had little or no effect on IK[Ca], whereas nifedipine and S-(-)-BAY K 8644 inhibited and potentiated IK[Ca], respectively. These results indicate that IK[Ca] channels are preferentially coupled to L-type, but not to N-type, Ca2+ channels on chicken ciliary ganglion neurons. Chicken sympathetic neurons also express dihydropyridine-sensitive and omega-conotoxin-sensitive components of ICa. However, in those cells, application of omega-conotoxin caused a 40-60% reduction in IK[Ca], whereas nifedipine reduced IK[Ca] but only in a subpopulation of cells. Therefore, IK[Ca] in sympathetic neurons is either coupled to N-type Ca2+ channels or is not selectively coupled to a single Ca(2+)-channel subtype. The preferential coupling of IK[Ca] channels with distinct ICa subtypes may be part of a mechanism to allow for selective modulation of neurotransmitter release. Preferential coupling may also be important for the differentiation and development of vertebrate neurons.
Full text
PDF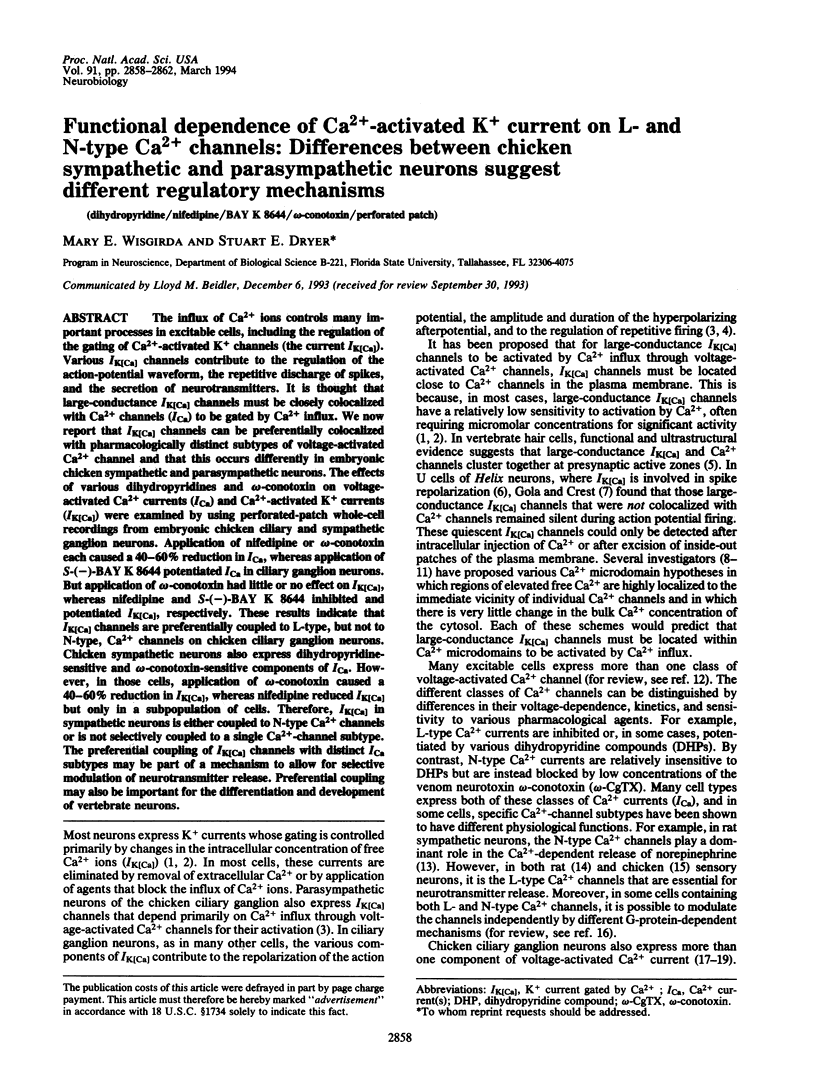
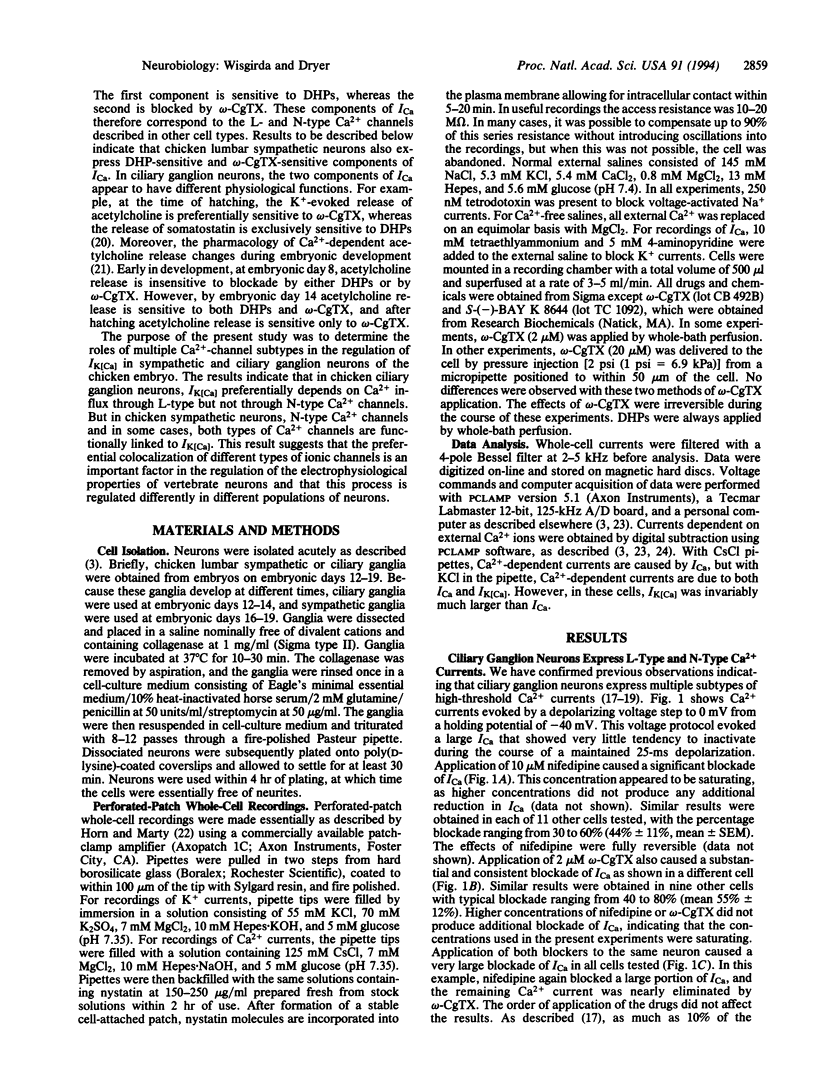
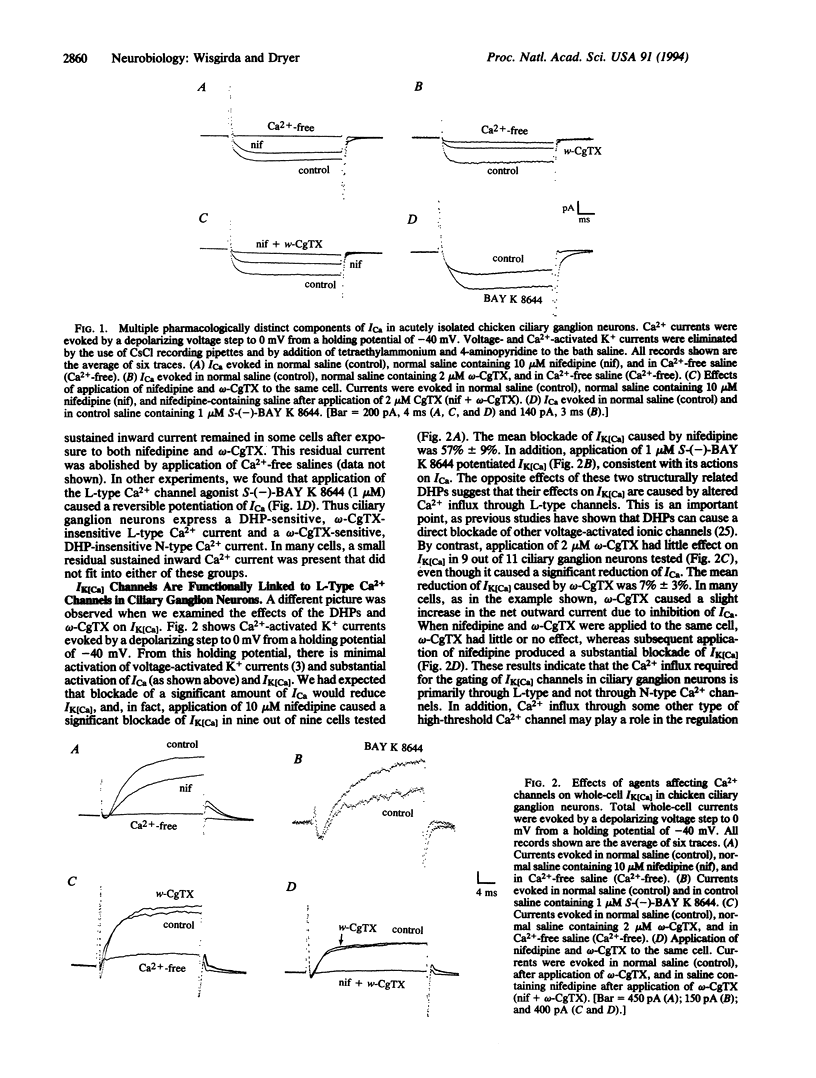
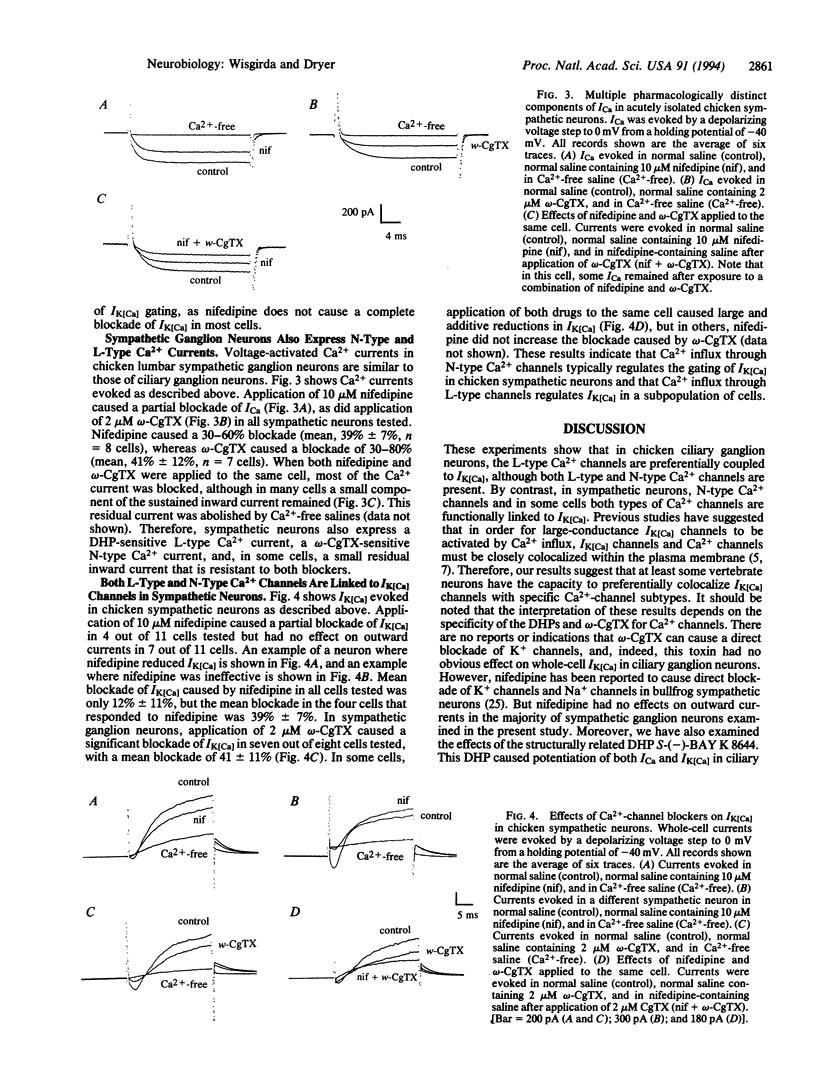
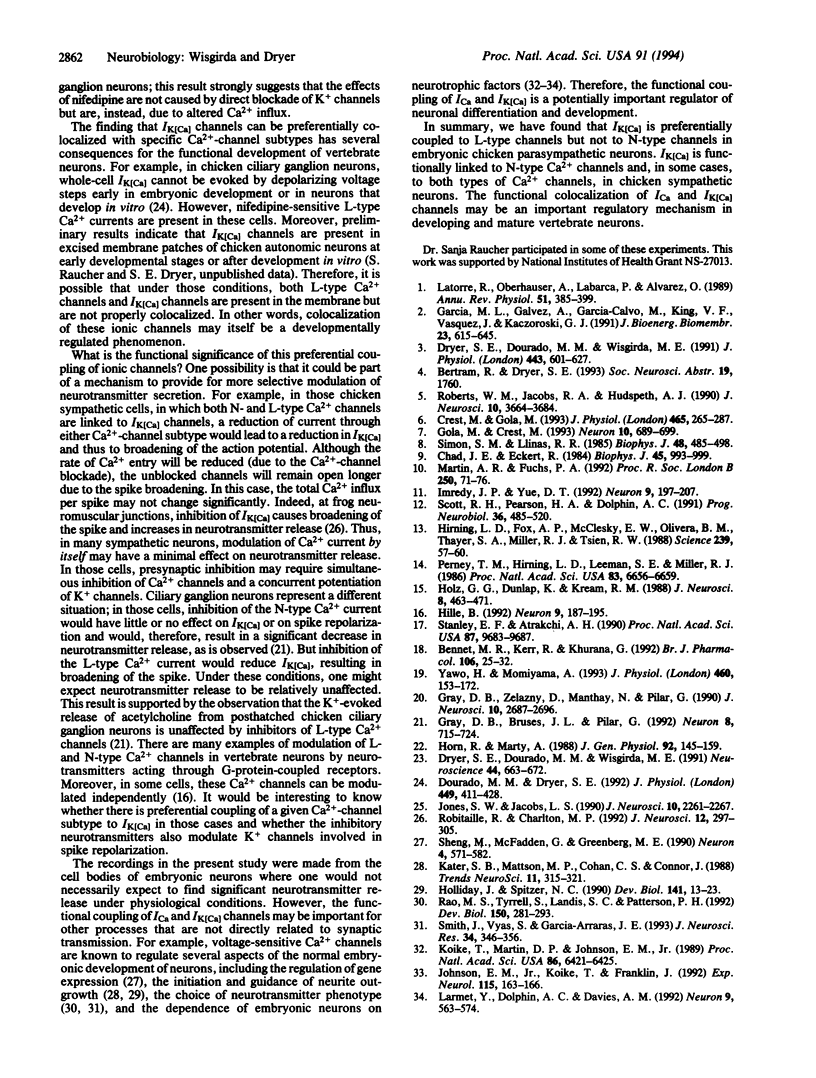
Selected References
These references are in PubMed. This may not be the complete list of references from this article.
- Bennett M. R., Kerr R., Khurana G. Adenosine modulation of calcium currents in postganglionic neurones of avian cultured ciliary ganglia. Br J Pharmacol. 1992 May;106(1):25–32. doi: 10.1111/j.1476-5381.1992.tb14287.x. [DOI] [PMC free article] [PubMed] [Google Scholar]
- Chad J. E., Eckert R. Calcium domains associated with individual channels can account for anomalous voltage relations of CA-dependent responses. Biophys J. 1984 May;45(5):993–999. doi: 10.1016/S0006-3495(84)84244-7. [DOI] [PMC free article] [PubMed] [Google Scholar]
- Crest M., Gola M. Large conductance Ca(2+)-activated K+ channels are involved in both spike shaping and firing regulation in Helix neurones. J Physiol. 1993 Jun;465:265–287. doi: 10.1113/jphysiol.1993.sp019676. [DOI] [PMC free article] [PubMed] [Google Scholar]
- Dourado M. M., Dryer S. E. Changes in the electrical properties of chick ciliary ganglion neurones during embryonic development. J Physiol. 1992 Apr;449:411–428. doi: 10.1113/jphysiol.1992.sp019093. [DOI] [PMC free article] [PubMed] [Google Scholar]
- Dryer S. E., Dourado M. M., Wisgirda M. E. Characteristics of multiple Ca(2+)-activated K+ channels in acutely dissociated chick ciliary-ganglion neurones. J Physiol. 1991 Nov;443:601–627. doi: 10.1113/jphysiol.1991.sp018854. [DOI] [PMC free article] [PubMed] [Google Scholar]
- Dryer S. E., Dourado M. M., Wisgirda M. E. Properties of Ca2+ currents in acutely dissociated neurons of the chick ciliary ganglion: inhibition by somatostatin-14 and somatostatin-28. Neuroscience. 1991;44(3):663–672. doi: 10.1016/0306-4522(91)90086-4. [DOI] [PubMed] [Google Scholar]
- Garcia M. L., Galvez A., Garcia-Calvo M., King V. F., Vazquez J., Kaczorowski G. J. Use of toxins to study potassium channels. J Bioenerg Biomembr. 1991 Aug;23(4):615–646. doi: 10.1007/BF00785814. [DOI] [PubMed] [Google Scholar]
- Gola M., Crest M. Colocalization of active KCa channels and Ca2+ channels within Ca2+ domains in helix neurons. Neuron. 1993 Apr;10(4):689–699. doi: 10.1016/0896-6273(93)90170-v. [DOI] [PubMed] [Google Scholar]
- Gray D. B., Brusés J. L., Pilar G. R. Developmental switch in the pharmacology of Ca2+ channels coupled to acetylcholine release. Neuron. 1992 Apr;8(4):715–724. doi: 10.1016/0896-6273(92)90092-r. [DOI] [PubMed] [Google Scholar]
- Gray D. B., Zelazny D., Manthay N., Pilar G. Endogenous modulation of ACh release by somatostatin and the differential roles of Ca2+ channels. J Neurosci. 1990 Aug;10(8):2687–2698. doi: 10.1523/JNEUROSCI.10-08-02687.1990. [DOI] [PMC free article] [PubMed] [Google Scholar]
- Hille B. G protein-coupled mechanisms and nervous signaling. Neuron. 1992 Aug;9(2):187–195. doi: 10.1016/0896-6273(92)90158-a. [DOI] [PubMed] [Google Scholar]
- Hirning L. D., Fox A. P., McCleskey E. W., Olivera B. M., Thayer S. A., Miller R. J., Tsien R. W. Dominant role of N-type Ca2+ channels in evoked release of norepinephrine from sympathetic neurons. Science. 1988 Jan 1;239(4835):57–61. doi: 10.1126/science.2447647. [DOI] [PubMed] [Google Scholar]
- Holliday J., Spitzer N. C. Spontaneous calcium influx and its roles in differentiation of spinal neurons in culture. Dev Biol. 1990 Sep;141(1):13–23. doi: 10.1016/0012-1606(90)90098-4. [DOI] [PubMed] [Google Scholar]
- Holz G. G., 4th, Dunlap K., Kream R. M. Characterization of the electrically evoked release of substance P from dorsal root ganglion neurons: methods and dihydropyridine sensitivity. J Neurosci. 1988 Feb;8(2):463–471. doi: 10.1523/JNEUROSCI.08-02-00463.1988. [DOI] [PMC free article] [PubMed] [Google Scholar]
- Horn R., Marty A. Muscarinic activation of ionic currents measured by a new whole-cell recording method. J Gen Physiol. 1988 Aug;92(2):145–159. doi: 10.1085/jgp.92.2.145. [DOI] [PMC free article] [PubMed] [Google Scholar]
- Imredy J. P., Yue D. T. Submicroscopic Ca2+ diffusion mediates inhibitory coupling between individual Ca2+ channels. Neuron. 1992 Aug;9(2):197–207. doi: 10.1016/0896-6273(92)90159-b. [DOI] [PubMed] [Google Scholar]
- Johnson E. M., Jr, Koike T., Franklin J. A "calcium set-point hypothesis" of neuronal dependence on neurotrophic factor. Exp Neurol. 1992 Jan;115(1):163–166. doi: 10.1016/0014-4886(92)90242-i. [DOI] [PubMed] [Google Scholar]
- Jones S. W., Jacobs L. S. Dihydropyridine actions on calcium currents of frog sympathetic neurons. J Neurosci. 1990 Jul;10(7):2261–2267. doi: 10.1523/JNEUROSCI.10-07-02261.1990. [DOI] [PMC free article] [PubMed] [Google Scholar]
- Kater S. B., Mattson M. P., Cohan C., Connor J. Calcium regulation of the neuronal growth cone. Trends Neurosci. 1988 Jul;11(7):315–321. doi: 10.1016/0166-2236(88)90094-x. [DOI] [PubMed] [Google Scholar]
- Koike T., Martin D. P., Johnson E. M., Jr Role of Ca2+ channels in the ability of membrane depolarization to prevent neuronal death induced by trophic-factor deprivation: evidence that levels of internal Ca2+ determine nerve growth factor dependence of sympathetic ganglion cells. Proc Natl Acad Sci U S A. 1989 Aug;86(16):6421–6425. doi: 10.1073/pnas.86.16.6421. [DOI] [PMC free article] [PubMed] [Google Scholar]
- Larmet Y., Dolphin A. C., Davies A. M. Intracellular calcium regulates the survival of early sensory neurons before they become dependent on neurotrophic factors. Neuron. 1992 Sep;9(3):563–574. doi: 10.1016/0896-6273(92)90193-h. [DOI] [PubMed] [Google Scholar]
- Latorre R., Oberhauser A., Labarca P., Alvarez O. Varieties of calcium-activated potassium channels. Annu Rev Physiol. 1989;51:385–399. doi: 10.1146/annurev.ph.51.030189.002125. [DOI] [PubMed] [Google Scholar]
- Martin A. R., Fuchs P. A. The dependence of calcium-activated potassium currents on membrane potential. Proc Biol Sci. 1992 Oct 22;250(1327):71–76. doi: 10.1098/rspb.1992.0132. [DOI] [PubMed] [Google Scholar]
- Perney T. M., Hirning L. D., Leeman S. E., Miller R. J. Multiple calcium channels mediate neurotransmitter release from peripheral neurons. Proc Natl Acad Sci U S A. 1986 Sep;83(17):6656–6659. doi: 10.1073/pnas.83.17.6656. [DOI] [PMC free article] [PubMed] [Google Scholar]
- Rao M. S., Tyrrell S., Landis S. C., Patterson P. H. Effects of ciliary neurotrophic factor (CNTF) and depolarization on neuropeptide expression in cultured sympathetic neurons. Dev Biol. 1992 Apr;150(2):281–293. doi: 10.1016/0012-1606(92)90242-9. [DOI] [PubMed] [Google Scholar]
- Roberts W. M., Jacobs R. A., Hudspeth A. J. Colocalization of ion channels involved in frequency selectivity and synaptic transmission at presynaptic active zones of hair cells. J Neurosci. 1990 Nov;10(11):3664–3684. doi: 10.1523/JNEUROSCI.10-11-03664.1990. [DOI] [PMC free article] [PubMed] [Google Scholar]
- Robitaille R., Charlton M. P. Presynaptic calcium signals and transmitter release are modulated by calcium-activated potassium channels. J Neurosci. 1992 Jan;12(1):297–305. doi: 10.1523/JNEUROSCI.12-01-00297.1992. [DOI] [PMC free article] [PubMed] [Google Scholar]
- Scott R. H., Pearson H. A., Dolphin A. C. Aspects of vertebrate neuronal voltage-activated calcium currents and their regulation. Prog Neurobiol. 1991;36(6):485–520. doi: 10.1016/0301-0082(91)90014-r. [DOI] [PubMed] [Google Scholar]
- Sheng M., McFadden G., Greenberg M. E. Membrane depolarization and calcium induce c-fos transcription via phosphorylation of transcription factor CREB. Neuron. 1990 Apr;4(4):571–582. doi: 10.1016/0896-6273(90)90115-v. [DOI] [PubMed] [Google Scholar]
- Simon S. M., Llinás R. R. Compartmentalization of the submembrane calcium activity during calcium influx and its significance in transmitter release. Biophys J. 1985 Sep;48(3):485–498. doi: 10.1016/S0006-3495(85)83804-2. [DOI] [PMC free article] [PubMed] [Google Scholar]
- Smith J., Vyas S., Garcia-Arraras J. E. Selective modulation of cholinergic properties in cultures of avian embryonic sympathetic ganglia. J Neurosci Res. 1993 Feb 15;34(3):346–356. doi: 10.1002/jnr.490340312. [DOI] [PubMed] [Google Scholar]
- Stanley E. F., Atrakchi A. H. Calcium currents recorded from a vertebrate presynaptic nerve terminal are resistant to the dihydropyridine nifedipine. Proc Natl Acad Sci U S A. 1990 Dec;87(24):9683–9687. doi: 10.1073/pnas.87.24.9683. [DOI] [PMC free article] [PubMed] [Google Scholar]
- Yawo H., Momiyama A. Re-evaluation of calcium currents in pre- and postsynaptic neurones of the chick ciliary ganglion. J Physiol. 1993 Jan;460:153–172. doi: 10.1113/jphysiol.1993.sp019464. [DOI] [PMC free article] [PubMed] [Google Scholar]