Abstract
The de novo design and structural characterization of an alpha-helical hairpin peptide (alpha-helix/turn/alpha-helix, alpha t alpha) are reported. The peptide is intended to provide a model system for the study of the interactions of secondary structural elements during protein folding. Both the diffusion-collision and framework models of protein folding envision that the earliest intermediates in protein folding are transient secondary structures or microdomains which interact and become mutually stabilizing. Design principles for the alpha t alpha peptide were drawn from the large body of work on the structure of peptides in solution. Computer modeling was not used in the design process. Study of alpha t alpha by circular dichroism and two-dimensional nuclear magnetic resonance indicates that the designed peptide is monomeric, helical, and stable in aqueous solution at room temperature. Analysis of two-dimensional nuclear magnetic resonance experiments indicates that the two helices and the turn form in the intended positions and that the helices associate in the designed orientation. Development of alpha t alpha represents an advance in protein design in that both the secondary structural elements and designed tertiary interactions have been realized and can be detected in solution by nuclear magnetic resonance. The resulting model system resembles a protein folding intermediate and will allow the study of interacting helices in a context that approximates an early stage in protein folding.
Full text
PDF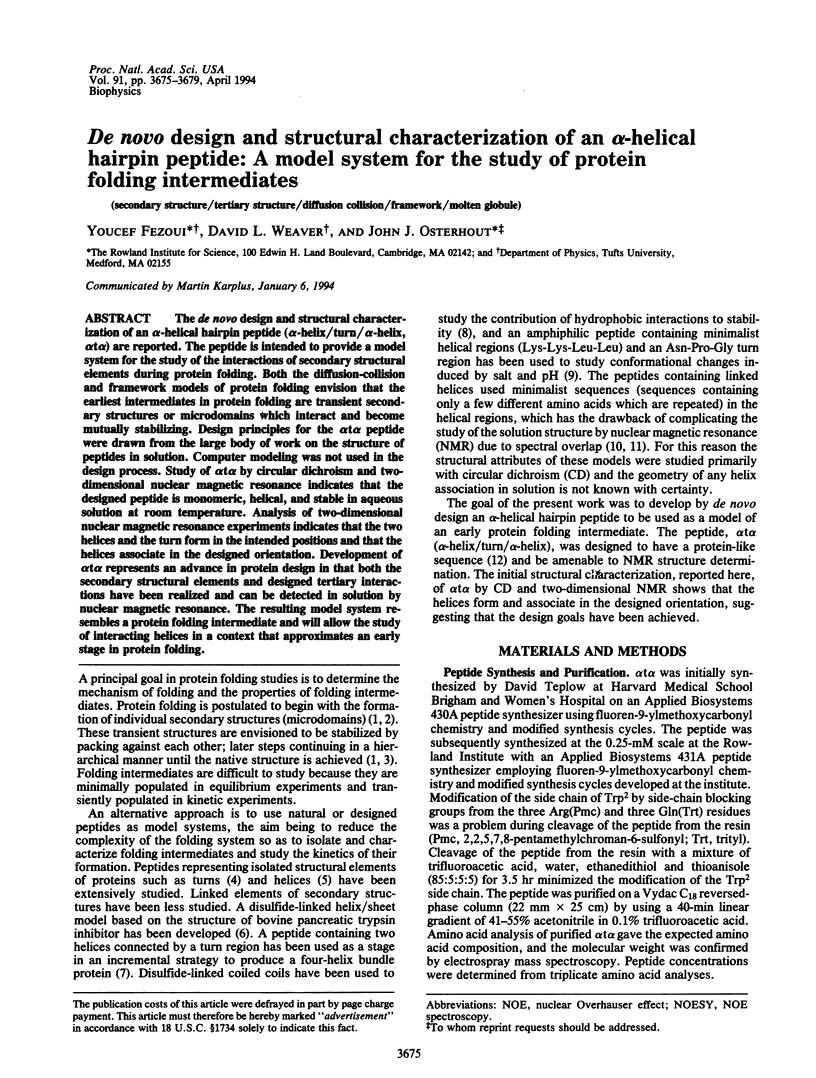
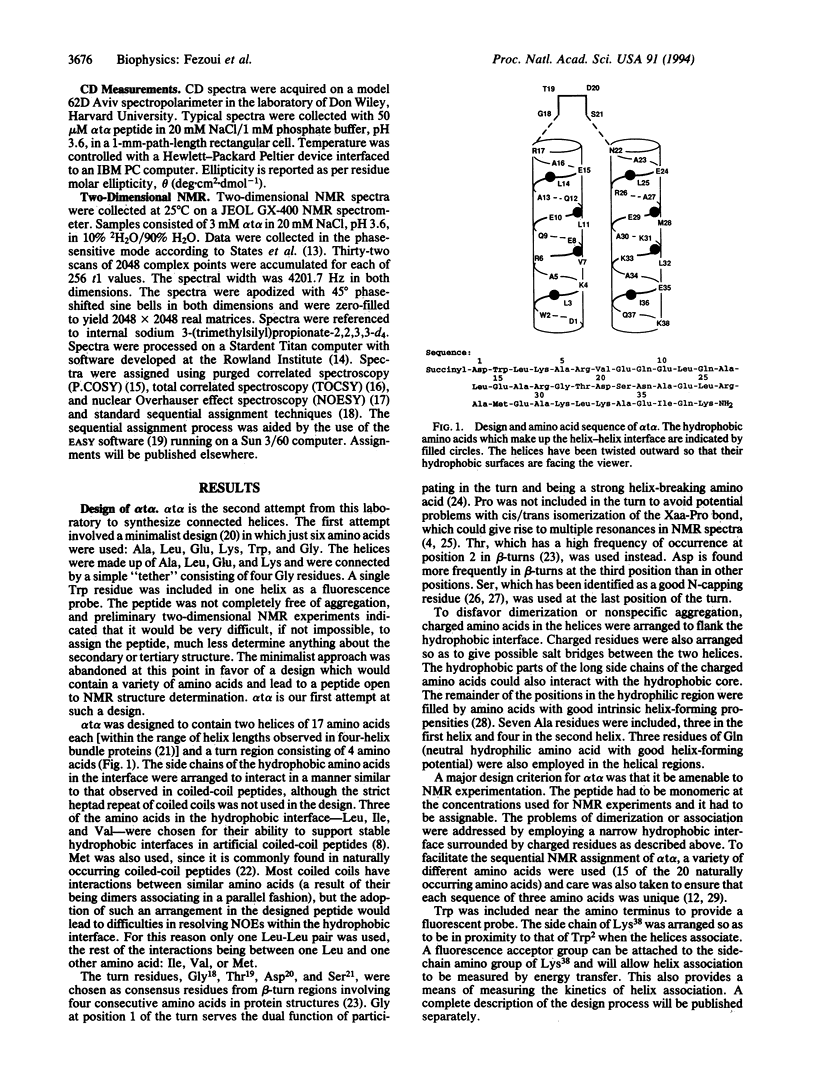
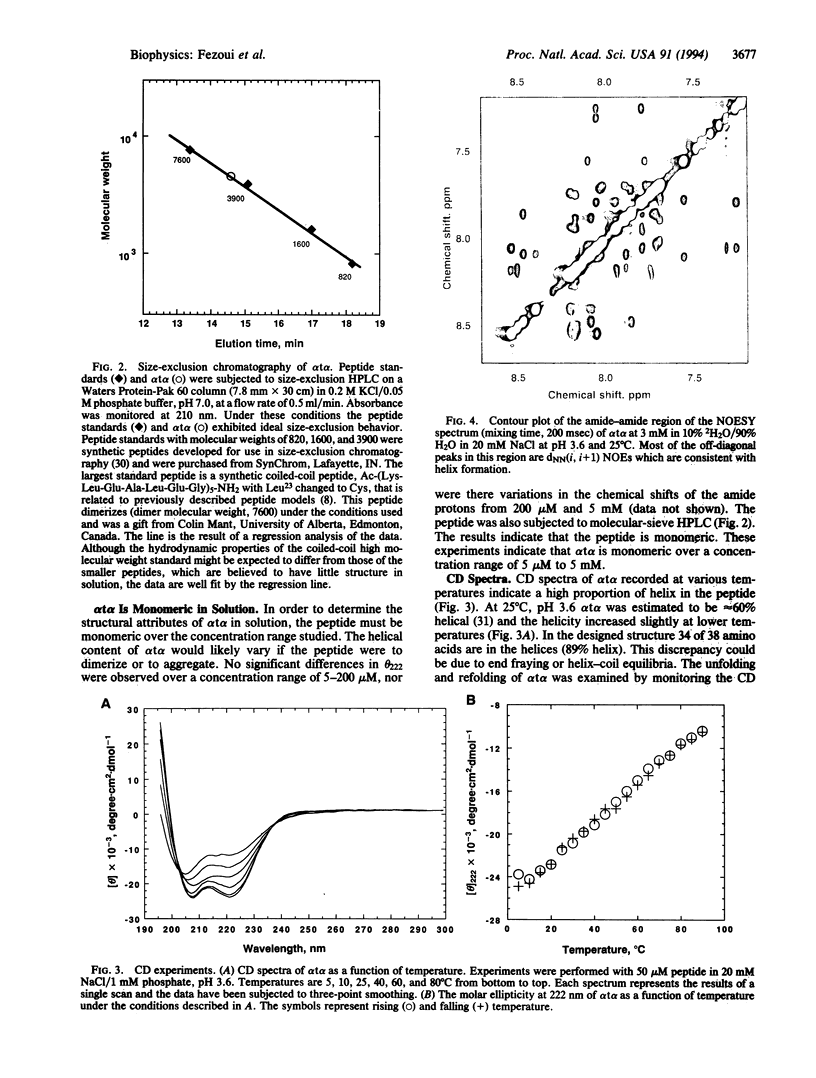
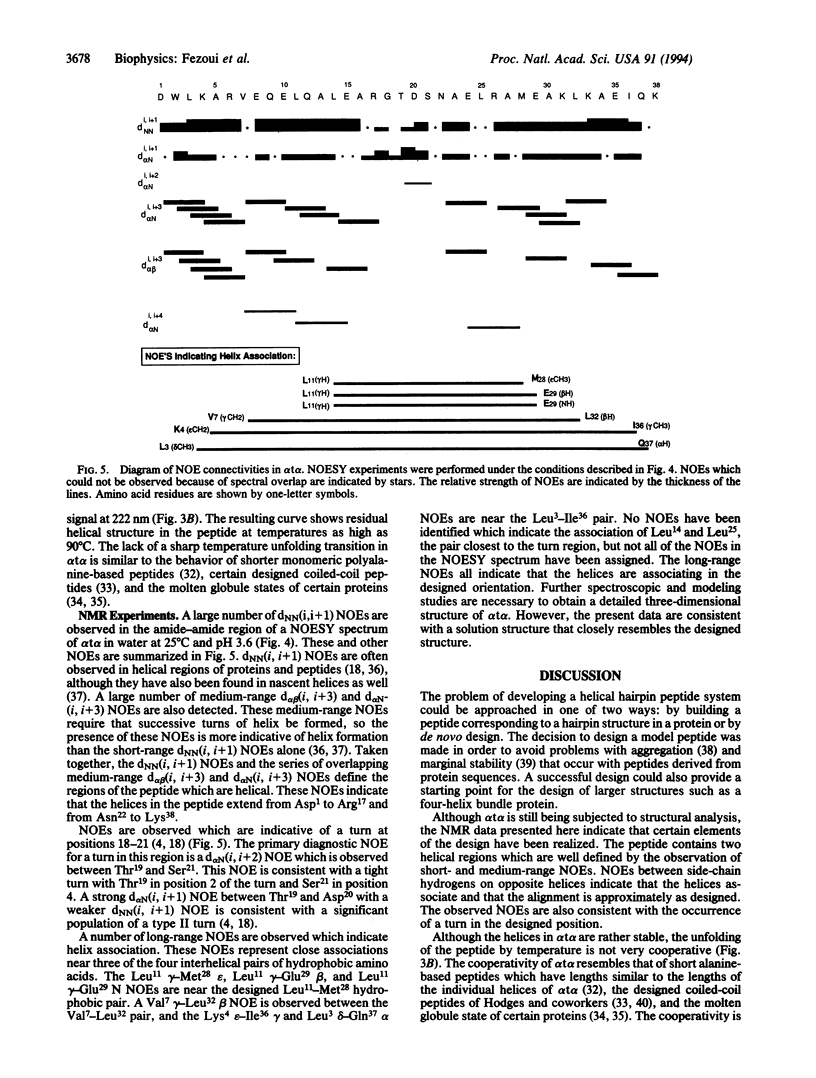
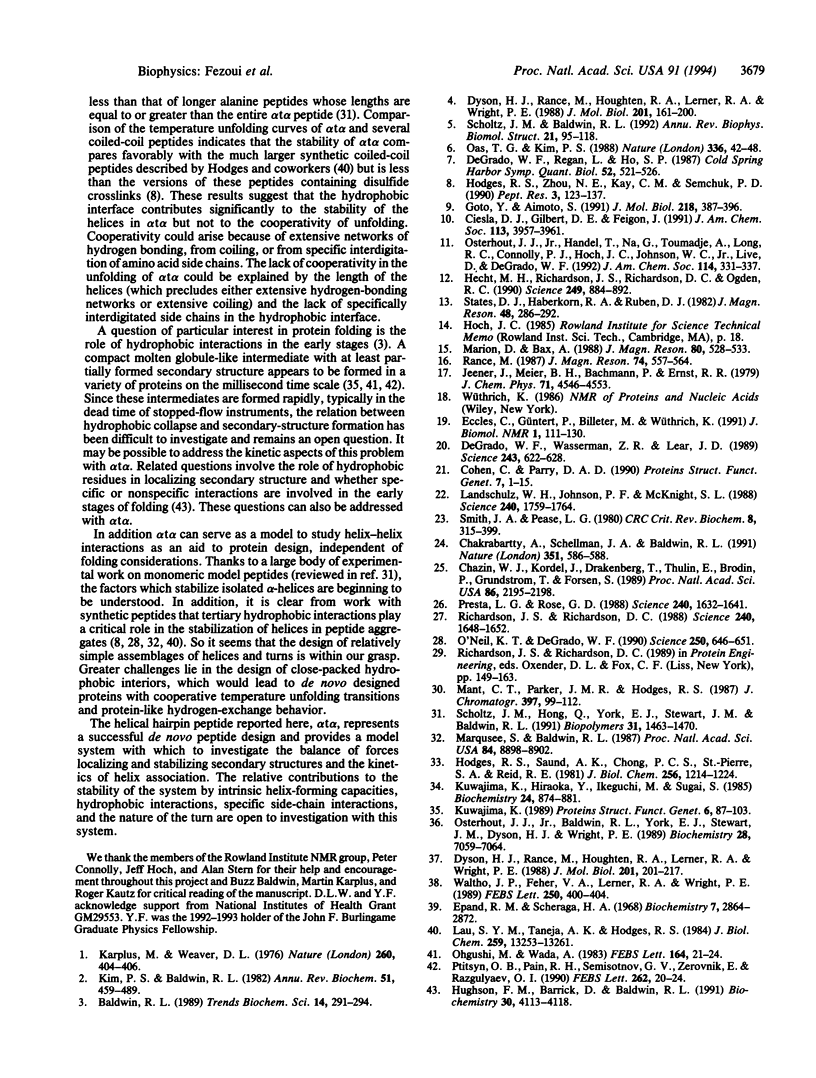
Images in this article
Selected References
These references are in PubMed. This may not be the complete list of references from this article.
- Baldwin R. L. How does protein folding get started? Trends Biochem Sci. 1989 Jul;14(7):291–294. doi: 10.1016/0968-0004(89)90067-4. [DOI] [PubMed] [Google Scholar]
- Chakrabartty A., Schellman J. A., Baldwin R. L. Large differences in the helix propensities of alanine and glycine. Nature. 1991 Jun 13;351(6327):586–588. doi: 10.1038/351586a0. [DOI] [PubMed] [Google Scholar]
- Chazin W. J., Kördel J., Drakenberg T., Thulin E., Brodin P., Grundström T., Forsén S. Proline isomerism leads to multiple folded conformations of calbindin D9k: direct evidence from two-dimensional 1H NMR spectroscopy. Proc Natl Acad Sci U S A. 1989 Apr;86(7):2195–2198. doi: 10.1073/pnas.86.7.2195. [DOI] [PMC free article] [PubMed] [Google Scholar]
- Cohen C., Parry D. A. Alpha-helical coiled coils and bundles: how to design an alpha-helical protein. Proteins. 1990;7(1):1–15. doi: 10.1002/prot.340070102. [DOI] [PubMed] [Google Scholar]
- DeGrado W. F., Regan L., Ho S. P. The design of a four-helix bundle protein. Cold Spring Harb Symp Quant Biol. 1987;52:521–526. doi: 10.1101/sqb.1987.052.01.059. [DOI] [PubMed] [Google Scholar]
- DeGrado W. F., Wasserman Z. R., Lear J. D. Protein design, a minimalist approach. Science. 1989 Feb 3;243(4891):622–628. doi: 10.1126/science.2464850. [DOI] [PubMed] [Google Scholar]
- Dyson H. J., Rance M., Houghten R. A., Lerner R. A., Wright P. E. Folding of immunogenic peptide fragments of proteins in water solution. I. Sequence requirements for the formation of a reverse turn. J Mol Biol. 1988 May 5;201(1):161–200. doi: 10.1016/0022-2836(88)90446-9. [DOI] [PubMed] [Google Scholar]
- Dyson H. J., Rance M., Houghten R. A., Wright P. E., Lerner R. A. Folding of immunogenic peptide fragments of proteins in water solution. II. The nascent helix. J Mol Biol. 1988 May 5;201(1):201–217. doi: 10.1016/0022-2836(88)90447-0. [DOI] [PubMed] [Google Scholar]
- Eccles C., Güntert P., Billeter M., Wüthrich K. Efficient analysis of protein 2D NMR spectra using the software package EASY. J Biomol NMR. 1991 Jul;1(2):111–130. doi: 10.1007/BF01877224. [DOI] [PubMed] [Google Scholar]
- Epand R. M., Scheraga H. A. The influence of long-range interactions on the structure of myoglobin. Biochemistry. 1968 Aug;7(8):2864–2872. doi: 10.1021/bi00848a024. [DOI] [PubMed] [Google Scholar]
- Goto Y., Aimoto S. Anion and pH-dependent conformational transition of an amphiphilic polypeptide. J Mol Biol. 1991 Mar 20;218(2):387–396. doi: 10.1016/0022-2836(91)90720-q. [DOI] [PubMed] [Google Scholar]
- Hecht M. H., Richardson J. S., Richardson D. C., Ogden R. C. De novo design, expression, and characterization of Felix: a four-helix bundle protein of native-like sequence. Science. 1990 Aug 24;249(4971):884–891. doi: 10.1126/science.2392678. [DOI] [PubMed] [Google Scholar]
- Hodges R. S., Saund A. K., Chong P. C., St-Pierre S. A., Reid R. E. Synthetic model for two-stranded alpha-helical coiled-coils. Design, synthesis, and characterization of an 86-residue analog of tropomyosin. J Biol Chem. 1981 Feb 10;256(3):1214–1224. [PubMed] [Google Scholar]
- Hodges R. S., Zhou N. E., Kay C. M., Semchuk P. D. Synthetic model proteins: contribution of hydrophobic residues and disulfide bonds to protein stability. Pept Res. 1990 May-Jun;3(3):123–137. [PubMed] [Google Scholar]
- Hughson F. M., Barrick D., Baldwin R. L. Probing the stability of a partly folded apomyoglobin intermediate by site-directed mutagenesis. Biochemistry. 1991 Apr 30;30(17):4113–4118. doi: 10.1021/bi00231a001. [DOI] [PubMed] [Google Scholar]
- Karplus M., Weaver D. L. Protein-folding dynamics. Nature. 1976 Apr 1;260(5550):404–406. doi: 10.1038/260404a0. [DOI] [PubMed] [Google Scholar]
- Kim P. S., Baldwin R. L. Specific intermediates in the folding reactions of small proteins and the mechanism of protein folding. Annu Rev Biochem. 1982;51:459–489. doi: 10.1146/annurev.bi.51.070182.002331. [DOI] [PubMed] [Google Scholar]
- Kuwajima K., Hiraoka Y., Ikeguchi M., Sugai S. Comparison of the transient folding intermediates in lysozyme and alpha-lactalbumin. Biochemistry. 1985 Feb 12;24(4):874–881. doi: 10.1021/bi00325a010. [DOI] [PubMed] [Google Scholar]
- Kuwajima K. The molten globule state as a clue for understanding the folding and cooperativity of globular-protein structure. Proteins. 1989;6(2):87–103. doi: 10.1002/prot.340060202. [DOI] [PubMed] [Google Scholar]
- Landschulz W. H., Johnson P. F., McKnight S. L. The leucine zipper: a hypothetical structure common to a new class of DNA binding proteins. Science. 1988 Jun 24;240(4860):1759–1764. doi: 10.1126/science.3289117. [DOI] [PubMed] [Google Scholar]
- Lau S. Y., Taneja A. K., Hodges R. S. Synthesis of a model protein of defined secondary and quaternary structure. Effect of chain length on the stabilization and formation of two-stranded alpha-helical coiled-coils. J Biol Chem. 1984 Nov 10;259(21):13253–13261. [PubMed] [Google Scholar]
- Mant C. T., Parker J. M., Hodges R. S. Size-exclusion high-performance liquid chromatography of peptides. Requirement for peptide standards to monitor column performance and non-ideal behaviour. J Chromatogr. 1987 Jun 26;397:99–112. doi: 10.1016/s0021-9673(01)84993-1. [DOI] [PubMed] [Google Scholar]
- Marqusee S., Baldwin R. L. Helix stabilization by Glu-...Lys+ salt bridges in short peptides of de novo design. Proc Natl Acad Sci U S A. 1987 Dec;84(24):8898–8902. doi: 10.1073/pnas.84.24.8898. [DOI] [PMC free article] [PubMed] [Google Scholar]
- O'Neil K. T., DeGrado W. F. A thermodynamic scale for the helix-forming tendencies of the commonly occurring amino acids. Science. 1990 Nov 2;250(4981):646–651. doi: 10.1126/science.2237415. [DOI] [PubMed] [Google Scholar]
- Oas T. G., Kim P. S. A peptide model of a protein folding intermediate. Nature. 1988 Nov 3;336(6194):42–48. doi: 10.1038/336042a0. [DOI] [PubMed] [Google Scholar]
- Ohgushi M., Wada A. 'Molten-globule state': a compact form of globular proteins with mobile side-chains. FEBS Lett. 1983 Nov 28;164(1):21–24. doi: 10.1016/0014-5793(83)80010-6. [DOI] [PubMed] [Google Scholar]
- Osterhout J. J., Jr, Baldwin R. L., York E. J., Stewart J. M., Dyson H. J., Wright P. E. 1H NMR studies of the solution conformations of an analogue of the C-peptide of ribonuclease A. Biochemistry. 1989 Aug 22;28(17):7059–7064. doi: 10.1021/bi00443a042. [DOI] [PubMed] [Google Scholar]
- Presta L. G., Rose G. D. Helix signals in proteins. Science. 1988 Jun 17;240(4859):1632–1641. doi: 10.1126/science.2837824. [DOI] [PubMed] [Google Scholar]
- Ptitsyn O. B., Pain R. H., Semisotnov G. V., Zerovnik E., Razgulyaev O. I. Evidence for a molten globule state as a general intermediate in protein folding. FEBS Lett. 1990 Mar 12;262(1):20–24. doi: 10.1016/0014-5793(90)80143-7. [DOI] [PubMed] [Google Scholar]
- Richardson J. S., Richardson D. C. Amino acid preferences for specific locations at the ends of alpha helices. Science. 1988 Jun 17;240(4859):1648–1652. doi: 10.1126/science.3381086. [DOI] [PubMed] [Google Scholar]
- Scholtz J. M., Baldwin R. L. The mechanism of alpha-helix formation by peptides. Annu Rev Biophys Biomol Struct. 1992;21:95–118. doi: 10.1146/annurev.bb.21.060192.000523. [DOI] [PubMed] [Google Scholar]
- Scholtz J. M., Qian H., York E. J., Stewart J. M., Baldwin R. L. Parameters of helix-coil transition theory for alanine-based peptides of varying chain lengths in water. Biopolymers. 1991 Nov;31(13):1463–1470. doi: 10.1002/bip.360311304. [DOI] [PubMed] [Google Scholar]
- Smith J. A., Pease L. G. Reverse turns in peptides and proteins. CRC Crit Rev Biochem. 1980;8(4):315–399. doi: 10.3109/10409238009105470. [DOI] [PubMed] [Google Scholar]
- Waltho J. P., Feher V. A., Lerner R. A., Wright P. E. Conformation of a T cell stimulating peptide in aqueous solution. FEBS Lett. 1989 Jul 3;250(2):400–404. doi: 10.1016/0014-5793(89)80764-1. [DOI] [PubMed] [Google Scholar]